Integrated watershed management planning for St. Lucia
Downloadable content.
- Cox, Christopher Anthony
- Madramootoo, Chandra (Supervisor)
- A decision support framework (DSF) to guide watershed management planning in St. Lucia was developed. The DSF, supported by three components, integrated physiographic criteria in spatially defining sustainable land management regimes, crop suitability based on agro-ecologic zoning criteria, and water quality modelling tools. In the first component, a methodology for agricultural and forestry land management zoning based on decision-rule frameworks was developed, employing GIS-based spatial multiple-criteria integration techniques. Land capability, recommended land management regimes, crop suitability and broad agricultural/forestry land utilization type zones were derived for the country. Sixteen broad agricultural and forestry land utilization types (LUTs) based on FAO agro-ecologic zoning guidelines were spatially defined over the island. These LUT zones represent relative suitability for rain-fed annual and perennial crop production, grazing and forestry. In the second component, field research to quantify rainfall, runoff and erosion from two small watersheds under contrasting land management regimes was undertaken. Over the study period the erosion rate from an intensively cultivated, degraded agricultural watershed was 20 times that of a completely forested watershed. SCS curve numbers were evaluated for both watersheds based on rainfall-runoff relationships. The third component demonstrated the application of a distributed-parameter hydrologic/water quality model, AnnAGNPS, in land management scenario evaluation, in terms of runoff and soil erosion. Data from the second component were used to calibrate and validate the model in simulation of daily runoff and erosion losses from the two watersheds over the study period. The model generally performed better in runoff simulation for the agricultural watershed compared to the forested watershed. Average annual erosion rates under current land management regimes were estimated at 73.3 and 7.2 t/ha for the agricultural and forested watersheds respectively. The model was applied to simulate runoff and erosion losses from the agricultural watershed under alternative sustainable land management regimes derived in the first component. Simulated average annual erosion losses were reduced to 9.2 t/ha. This study demonstrated the application of efficient and powerful computer-based tools in the development of a decision support framework for watershed management planning for small islands.
- Watershed management -- Saint Lucia -- Decision making.
- Water quality -- Saint Lucia -- Computer simulation.
- McGill University
- https://escholarship.mcgill.ca/concern/theses/td96k312r
- All items in eScholarship@McGill are protected by copyright with all rights reserved unless otherwise indicated.
- Department of Bioresource Engineering
- Doctor of Philosophy
- Theses & Dissertations
Thumbnail | Title | Date Uploaded | Visibility | Actions |
---|---|---|---|---|
2019-09-23 | Public |
- Bibliography
- More Referencing guides Blog Automated transliteration Relevant bibliographies by topics
- Automated transliteration
- Relevant bibliographies by topics
- Referencing guides

Opportunities and Challenges to Adopting Sustainable Watershed Management Interventions: An Overview of Experiences from Ethiopia
- First Online: 11 November 2020
Cite this chapter
- Gebreyesus Brhane Tesfahunegn 3 , 4 &
- Elias T. Ayuk 4
524 Accesses
2 Citations
Watershed management techniques in view of the water–soil–waste nexus approach and its link to food security have been widely studied in Ethiopia. However, there has been limited uptake and implementation of the recommended technologies in many watersheds. This paper aims to illustrate the experiences and lessons about watershed management interventions in the Tigray region, northern Ethiopia and identifies opportunities and challenges to the adoption of these practices. Information was collected based on discussions with farmers and development agents, field observations, personal experiences, and literature review. Positive impacts of the nexus-oriented watershed management interventions included 50% increase in income, 56% increase in food security, 62% increase in vegetation coverage, 63% increase in surface and groundwater resources, 62% restoration of soil fertility, sediment accumulation behind bunds (up to 65 t ha −1 year −1 ), and 80% reduction in soil loss. In Ethiopia, opportunities for the widespread adoption of watershed management interventions include improvement in socioeconomic conditions, experiences on natural resources restoration, farm diversification and intensification, and understanding the complementarity of crop and livestock systems. On the other hand, adoption of these interventions was challenged by a lack of improved agronomic practices, poor crop and livestock management, unpredictable climate, the large area of land under severe degradation, and high competition between crop and livestock systems. In order to improve the adoption of nexus-oriented watershed management interventions, a conceptual research strategy including local and scientific knowledge and approaches (from participatory problem identification to monitoring, and evaluation strategy) is suggested.
This is a preview of subscription content, log in via an institution to check access.
Access this chapter
- Available as PDF
- Read on any device
- Instant download
- Own it forever
- Available as EPUB and PDF
- Compact, lightweight edition
- Dispatched in 3 to 5 business days
- Free shipping worldwide - see info
- Durable hardcover edition
Tax calculation will be finalised at checkout
Purchases are for personal use only
Institutional subscriptions
Alemayehu F, Taha N, Nyssen J, Girma A, Zenebe A, Behailu M, Deckers J, Poesen J (2009) The impacts of watershed management on land use and land cover dynamics in Eastern Tigray (Ethiopia). Resour Conserv Recycl 53:192–198
Article Google Scholar
Alemu B, Kidane D (2014) The implication of integrated watershed management for rehabilitation of degraded lands: case study of Ethiopian highlands. J Agric Biodivers Res 3(6):78–90
Google Scholar
ATA-MOA (Ethiopian Agricultural Transformation Agency (ATA)—Federal Democratic Republic of Ethiopia Ministry of Agriculture (MOA)) (2014) National Strategy for Ethiopia’s agricultural extension system- vision, systemic bottlenecks and priority interventions
Behailu M (2002) Assessment and optimisation of traditional irrigation of vertisols in northern Ethiopia: a case study at Gumselasa micro-dam using maize as an indicator crop. Unpublished Ph.D. Thesis, Gent, Belgium
Benavides L, Avellán T, Caucci S, Hahn A, Kirschke S (2019) Müller A (2019) Assessing sustainability of wastewater management systems in a multi-scalar, transdisciplinary manner in Latin America. Water 11(2):249. https://doi.org/10.3390/w11020249
Bishaw B (2001) Deforestation and land degradation on the Ethiopian highlands: a strategy for physical recovery. Paper presented at International Conference on Contemporary Development Issues in Ethiopia, Kalamazoo, Michigan, U.S.A. https://www.fao.org/forestry/5856-05a0e08cfda318d93eb26e620f87190b.pdf . Accessed 28 Mar 2017
Dube AK, Fawole WO, Govindasamy R, Ozkan B (2019) Agricultural development led industrialization in Ethiopia: structural break analysis. Int J Agric, For Life Sci 3(1):193–201
Ethiopia Environmental Protection Authority. Ethiopian Environmental Report (2003) Commercial Printing Press. Addis Ababa, Ethiopia
Food and Agriculture Organization of the United Nations, FAO (1986) Ethiopian highland reclamation study. Final report, vol 1–2 (1986). Food and Agriculture Organization of the United Nations, Rome
FAO (2014) Walking the Nexus Talk: assessing the water-energy-food nexus in the context of the sustainable energy for all initiative. FAO environment and natural resources working paper # 58. https://www.fao.org/3/a-i3959e.pdf
Fantaw A (2007) An overview of salt affected soils and their management in Ethiopia. Paper presented in the third international workshop on water management (Water Man) project, 19–21 Sep 2007, Haramaya University, Haramaya, Ethiopia. https://www.wau.boku.ac.at/fileadmin/data/H03000/H81000/H81500/waterman/Workshop_III_Haramaya.pdf . Accessed 27 Jun 2017
Fenta AA, Yasuda H, Shimizu K, Haregeweyn N, Negussie A (2016) Dynamics of soil erosion as influenced by watershed management practices: a Case study of the Agula watershed in the semi-arid highlands of northern Ethiopia”. Environ Manage 58(5):889–905
Gashie W (2005) Factors controlling households energy use: implication for the conservation of the environment. Master Thesis, Addis Ababa University, Ethiopia
Gebregziabher G, Abera DA., Gebresamuel G, Giordano M, Langan S (2016) An assessment of integrated watershed management in Ethiopia. Colombo, Sri Lanka: International Water Management Institute (IWMI). IWMI Working Paper 170, p 28. https://doi.org/10.5337/2016.214 . Accessed 23 Feb 2017
Gebremichael D, Nyssen J, Poesen J, Deckers J, Haile M, Govers G, Moeyersons J (2005) Effectiveness of stone bunds on controlling soil erosion on cropland in the Tigray highlands, northern Ethiopia. Soil Use Manage 21(3):287–297
Harry V (2013) The politics of African energy development: Ethiopia’s hydro-agricultural state-building strategy and clashing paradigms of water security. Philos Trans Roy Soc A-Math Phys Eng Sci 371(2002):20120411. https://doi.org/10.1098/rsta.2012.0411
Hülsmann S, Ardakanian R (2018) The nexus approach as tool for achieving SDGs: trends and needs. In: Hülsmann S, Ardakanian R (eds) Managing water, soil and waste resources to achieve sustainable development goals, pp 1–9. https://doi.org/10.1007/978-3-319-75163-4_1
Johnson OW, Karlberg L (2017) Co-exploring the water–energy–food nexus: facilitating dialogue through participatory scenario building. Front Environ Sci 5. https://doi.org/10.3389/fenvs.2017.00024
Karlberg L, Hoff H, Amsalu T, Andersson K, Binnington T, Flores-Lopez F, de Bruin A, Gebrehiwot SG, Gedif B, zur Heide F, Johnson O, Osbeck M, Young C (2014) Applying the nexus—meeting Ethiopia’s development goals by addressing links between water, energy and food. SEI Policy Brief. Stockholm Environment Institute, Stockholm
Karlberg L, Hoff H, Amsalu T, Andersson K, Binnington T, Flores-Lopez F, de Bruin A, Gebrehiwot SG, Gedif B, zur Heide F, Johnson O, Osbeck M, Young C, (2015) Tackling complexity: understanding the food–energy–environment nexus in Ethiopia’s Lake Tana Sub-Basin. Water Altern-An Interdisc J Water Polit Dev 8(1):710–734
Kerr J (2002) Watershed development, environmental services, and poverty alleviation in India. World Dev 30(8):1387–1400
Kerse BL (2017) A review paper on: the multi-functional implication of integrated watershed management: the new approach to degraded land rehabilitation in Ethiopia. J Biol, Agric Healthc 7(17):21–30
Lakew D, Carucci V, Asrat W, Yitayew A (eds) (2005) Community based participatory watershed development. Ministry of Agriculture and Rural Development, Addis Ababa, Ethiopia
Mannschatz T, Buchroithner MF, Hülsmann S (2015) Visualization of water services in africa: data applications for nexus governance. In: Kurian M, Ardakanian R (eds) Governing the nexus. Springer International Publishing, pp 189–217. https://doi.org/10.1007/978-3-319-05747-79
Meaza H (2015) The role of community based watershed management for climate change adaptation in Adwa, Central Tigray Zone, Ethiopia. Int J Weather, Clim Change Conserv Res 1(1):11–35
Mekonen K, Tesfahunegn GB (2011) Impact assessment of soil and water conservation measures at Medego watershed in Tigray, northern Ethiopia. Maejo Int J Sci Technol 5(03):312–330
Mekonnen GT, Fekadu A (2015) Experiences and challenges of integrated watershed management in Central Zone of Southern Ethiopia. Int J Curr Res 7(10):20973–20979
Meshesha TW, Tripathi SK (2015) An Evaluation of watershed management practice in Ethiopia: a preliminary review. Int J Environ Sci 4(1):24–30
Ministry of Agriculture and Rural Development (MoAR) (2010) Sustainable land management technologies and approaches in Ethiopia: published by the Sustainable Land Management Project (SLMP), Natural resources management sector, Ministry of Agriculture and Rural Development of the Federal Democratic Republic of Ethiopia
Stockholm Environment Institute (SEI) (2014) Cross-sectoral integration in the sustainable development goals: a nexus approach. Discussion Brief. https://www.idaea.csic.es/medspring/sites/default/files/Cross-sectoral-integration-in-the-sustainable-development-goals-a-nexus-approach.pdf . Accessed 9 Jul 2018
Solomon N, Hishe H, Annang T, Pabi O, Asante IK, Birhane E (2018) Forest cover change, key drivers and community perception in WujigMahgo Waren Forest of northern Ethiopia. Land 7(32):1–16
Stoorvogel JJ, Smaling EMA (1990) Assessment of soil nutrient depletion in Sub-Saharan Africa, 1983–2000. Report 28. DLO Winand Staring Center for Integrated Land, Soil and Water Research, Wageningen, the Netherlands.
Tamene L (2005) Reservoir siltation in the drylands of northern Ethiopia: causes, source areas and management options. Ph.D. Thesis, University of Bonn, Germany
Tesfahunegn GB (2011) Soil erosion modeling and soil quality evaluation for catchment management strategies in northern Ethiopia. Ecology and Development Series No. 8, Center for Development Research, University of Bonn, Germany, (ISBN 978–3–940124–35–7, ISSN: 1864–0443)
Tesfahunegn GB, Vlek PLG (2013) Assessing sediment-nutrient export rate and soil degradation in mai-negus catchment, Northern Ethiopia. ISRN Soil Science, Article ID 748561. https://doi.org/10.1155/2013/748561
United Nations, (UN) (2015) Transforming our world: the 2030 agenda for sustainable development. Resolution adopted by the General Assembly on 25 (Sept) A/RES/70/1. United Nations General Assembly, New York
Wang X, Dong S, Yang B, Li Y, Su X (2014) The effects of grassland degradation on plant diversity, primary productivity and soil fertility in the alpine region of Asia’s headwaters. Environ Monit Assess 186:6903–6917
Article CAS Google Scholar
World Bank (2008) Project Appraisal Document on a Proposed Grant in the Amount of US$20 million and Proposed Grant from the Global Environment Facility Trust Fund in the Amount of US$9 Million to the Federal Democratic Republic of Ethiopia for Sustainable Land Management Project. Environmental & Natural Resources Management Sustainable Development Department AFTSN Africa Region, The Document of the World Bank, Report No: 42927-ET
Worku T, Tripathi SK (2015) Watershed management in highlands of Ethiopia: a review. Open Access Libr J 02:e1481. https://doi.org/10.4236/oalib.1101481
Download references
Acknowledgements
The authors are grateful to UNU-FLORES for the financial support for the first author to present this paper in the Dresden Nexus Conference (17–19 May 2017) in Germany. The support from UNU-INRA and Aksum University (Ethiopia) during fieldwork, development, and organization of this paper is also highly appreciated.
Author information
Authors and affiliations.
College of Agriculture, Aksum University, Shire-Campus, P. O. Box 314, Shire, Ethiopia
Gebreyesus Brhane Tesfahunegn
International House 2nd Floor, United Nations University Institute for Natural Resources in Africa (UNU-INRA), University of Ghana, Legon Campus, Accra, Ghana
Gebreyesus Brhane Tesfahunegn & Elias T. Ayuk
You can also search for this author in PubMed Google Scholar
Corresponding author
Correspondence to Gebreyesus Brhane Tesfahunegn .
Editor information
Editors and affiliations.
Institute for Integrated Management of Material Fluxes and of Resources, United Nations University, Dresden, Sachsen, Germany
Stephan Hülsmann
Mahesh Jampani
Rights and permissions
Reprints and permissions
Copyright information
© 2021 Springer Nature Switzerland AG
About this chapter
Tesfahunegn, G.B., Ayuk, E.T. (2021). Opportunities and Challenges to Adopting Sustainable Watershed Management Interventions: An Overview of Experiences from Ethiopia. In: Hülsmann, S., Jampani, M. (eds) A Nexus Approach for Sustainable Development . Springer, Cham. https://doi.org/10.1007/978-3-030-57530-4_11
Download citation
DOI : https://doi.org/10.1007/978-3-030-57530-4_11
Published : 11 November 2020
Publisher Name : Springer, Cham
Print ISBN : 978-3-030-57529-8
Online ISBN : 978-3-030-57530-4
eBook Packages : Earth and Environmental Science Earth and Environmental Science (R0)
Share this chapter
Anyone you share the following link with will be able to read this content:
Sorry, a shareable link is not currently available for this article.
Provided by the Springer Nature SharedIt content-sharing initiative
- Publish with us
Policies and ethics
- Find a journal
- Track your research
Thank you for visiting nature.com. You are using a browser version with limited support for CSS. To obtain the best experience, we recommend you use a more up to date browser (or turn off compatibility mode in Internet Explorer). In the meantime, to ensure continued support, we are displaying the site without styles and JavaScript.
- View all journals
- Explore content
- About the journal
- Publish with us
- Sign up for alerts
- Open access
- Published: 26 January 2023
A comparative analysis of urban forests for storm-water management
- Mohammad A. Rahman ORCID: orcid.org/0000-0001-9872-010X 1 ,
- Yanin Pawijit 1 ,
- Chao Xu 2 ,
- Astrid Moser-Reischl 3 ,
- Hans Pretzsch 3 ,
- Thomas Rötzer 3 &
- Stephan Pauleit 1
Scientific Reports volume 13 , Article number: 1451 ( 2023 ) Cite this article
3301 Accesses
2 Citations
5 Altmetric
Metrics details
- Plant evolution
- Urban ecology
Large-scale urban growth has modified the hydrological cycle of our cities, causing greater and faster runoff. Urban forests (UF), i.e. the stock of trees and shrubs, can substantially reduce runoff; still, how climate, tree functional types influence rainfall partitioning into uptake and runoff is mostly unknown. We analyzed 92 published studies to investigate: interception (I), transpiration (T), soil infiltration (IR) and the subsequent reduction in runoff. Trees showed the best runoff protection compared to other land uses. Within functional types, conifers provided better protection on an annual scale through higher I and T but broadleaved species provided better IR. Regarding tree traits, leaf area index (LAI) showed a positive influence for both I and T. For every unit of LAI increment, additional 5% rainfall partition through T (3%) and I (2%) can be predicted. Overall, runoff was significantly lower under mixed species stands. Increase of conifer stock to 30% in climate zones with significant winter precipitation and to 20% in areas of no dry season can reduce runoff to an additional 4%. The study presented an overview of UF potential to partition rainfall, which might help to select species and land uses in different climate zones for better storm-water management.
Similar content being viewed by others

Microbial competition for phosphorus limits the CO2 response of a mature forest
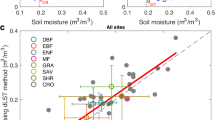
Global critical soil moisture thresholds of plant water stress
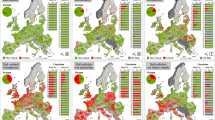
A unifying modelling of multiple land degradation pathways in Europe
Introduction.
Rapid and large-scale urban growth has modified the hydrological cycle of our cities by replacing natural vegetation surfaces with impervious surfaces, causing greater and faster runoff 1 , 2 . Barron et al. 3 reported an increase of the annual runoff coefficient from 1% in pre-development condition to 39% and reduction in evapotranspirative water loss from 443 to 154 mm after urbanization in an urbanized watershed in Western Australia. Between 1980 and 2018, direct economic impacts of urban flooding has exceeded US $1 trillion globally, with the loss of hundreds of thousands of lives 4 . In the context of advancing climate change and increasing storm intensity, cities have therefore been prompted to invest in ways to naturally capture, store and slowly release runoff through "green infrastructure" 5 . In Europe, the concept of nature-based solutions (NBS) is emphasized in policies to provide a wide range of ecosystem services 6 thus improving urban sustainability and resilience 5 , 7 , 8 . In recent years, concepts of NBS for storm-water management have appeared under many terms worldwide, i.e., Sustainable Urban Drainage System, Low Impact Developments in US, Blue-Green Cities in the UK, Water Sensitive Urban Design in Australia 9 , Low Impact Developments Urban Design in New Zealand and Sponge City in China 10 . Despite the differences in the names and objectives, each of the solutions share a common ground, aiming to integrate GI into urban areas to restore natural water cycles.
Vegetation can significantly reduce runoff 11 , 12 ; however, concepts and policies for urban forestry as NBS, peaked only in the mid-1990s 13 . Still, research on the interaction between urban forests, i.e. the urban stock of trees, and storm-water has been relatively understudied compared to other topics such as microclimatic amelioration 14 , air quality and carbon sequestration benefits 15 , 16 , 17 . Urban forests return water to the atmosphere through interception and evaporation, regulate ground-water flow by through-fall (rain that falls through the canopy) and stem flow (water that flows down the trunk or stem) leading towards soil infiltration 18 . Finally transpire water out of the soil, leading to increased soil water‐holding capacities 19 . Arguably, transpiration is the major component of water partitioning at annual scale though that can be different in closed canopy multilayered forests compared to isolated urban trees without any humus layer or rows of hedgerows or grass lawns and higher atmospheric demand 20 .
From an urban hydrological point of view, canopy interception is the most important effect and trees with their greatest stature can provide the greatest benefit in this respect. In forest condition, interception could be as large as 50% that of gross precipitation 21 . Whereas for individual trees this has been variously reported between 15 and 28% 22 . Depending on tree species, their canopies can retain between 0.03 and 2.24 mm H 2 O m −2 of leaf area as shown in a rainfall simulator study 23 . The whole process of rainfall partition is affected by three main factors: nature and magnitude of the rainfall event, functional types of vegetation, and weather conditions 22 . In general, conifers intercept and evaporate 20–40% of annual rainfall while deciduous forests intercept 10–20% 24 , compared to 15–32% by mixed forest stands 25 .
Reducing surface-runoff, increasing soil infiltration for further enhancing transpiration (hence cooling effect) cannot be promoted by a one-size-fits-all solution. Runoff from conifers is usually lower compared to deciduous stands, in particular where winter rainfall is significantly higher. Again, considering individual storm events, deciduous stands might retain more water than conifers 26 . Apart from tree crown structure and rainfall intensity and duration, overall water detention is largely dependent on the climatic conditions i.e., radiation, air humidity temperature, and wind speed 18 .
Studies on rainfall partitioning are largely fragmented, focusing on just one or few parts of the partition, with a wide range of values across studies. Moreover, global scientific discourse on urban forestry is limited to few regions. Ostoić and van den Bosch 13 reported 58% of all publications related to urban forestry is from the USA alone. Thus, comprehensive understanding of the magnitude of surface-runoff reduction potentials of different urban forests is mostly missing. Notably, vegetation with high species and functional diversity can reduce runoff mostly based on the canopy structure attributes. However, belowground traits in terms of improving the soil infiltration potential is mostly ignored. Importantly, the influence zone of a tree stem can be much higher than their canopy extent. Chandler and Chappell 27 showed significant positive influence on increasing soil infiltration of an old oak ( Quercus robur ) up to 10 m from the main stem. Therefore, knowledge concerning the rainfall partitioning through interception, infiltration, transpiration as part of the annual water balance in an urban context over higher spatial and temporal scales is needed. Such overview would provide storm water managers with a useful insight on different climate regions and different functional types of vegetation.
The objective of this study is to present an overview of how urban forests partition rainfall on an annual basis in different climate regions, to understand the implications of climate and different functional types of greenspaces on storm-water management. The research questions are:
How does climate affect rainfall partitioning into uptake and runoff of the urban forest?
How can different functional types of urban forests influence the reduction in storm-water runoff?
How do characteristics of tree species affect storm-water partitioning?
Search and selection of studies
Relevant studies were searched using internet search engines and websites of environmental organizations. Due to the lack of data in urban settings, forest stands were also included to fill the data gaps. Moreover, runoff coefficient and infiltration potential are mostly measured at landscape level, information on tree species were difficult to isolate. Consequently, runoff coefficient and soil hydraulic conductivity values were collected from studies covering different land use and land cover i.e., mixed forest, monospecific plantation, afforestation (1–30 years), and open spaces including bare soil in various climate zones. Keywords used were urban OR trees OR forest OR plantation OR storm-water OR interception OR infiltration OR evapotranspiration OR transpiration OR runoff OR infiltration rate OR hydraulic conductivity. Both empirical and modelling studies published between 1995 and 2021 were considered. The selection criteria following Stewart 28 includes: (1) studies regarding specific trees or shrubs in or around cities with quantifiable data on interception, evapotranspiration, infiltration or runoff; (2) specific land use containing values that can be converted into percentage of the total precipitation. Through-fall and stem flow were not explored since both end up in the soil and contribute either to runoff or infiltration.
Finally, 92 studies were selected for in-depth analyses (S-Table 1 ); 28 studies (81 data points) were considered for interception (50% from urban settings), 19 studies (67 data points) were selected for transpiration (75% from urban settings). There were 36 studies (201) data points for runoff coefficient and 9 studies (42 data points) for infiltration.
Classification
Location of each data point was assigned to a climate classification based on Beck et al. 29 ’s updated Köppen–Geiger climate classification (Fig. 1 ). For analysis, only the first two letters (climate group and seasonal precipitation) for climate groups C and D were used. Data from climate groups A, B and E were classified into three broad climate groups, without considering subgroups, as there were less data points. For studies that did not report climate classification, the place was located through Google Maps and the approximate location was compared manually.
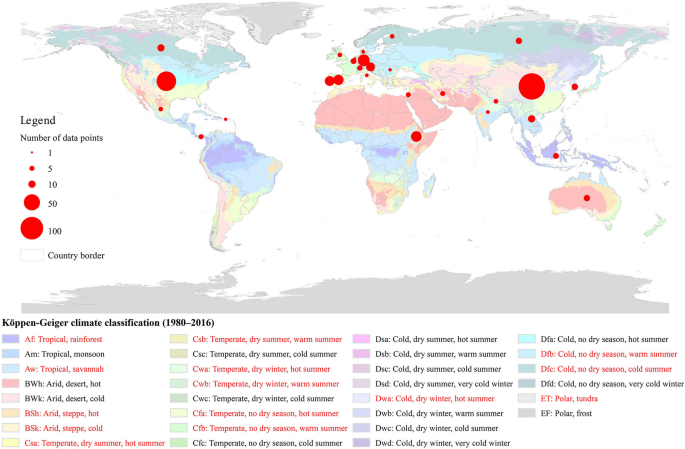
Overview of the Köppen–Geiger climate classes including the considered climate zones in this study (red colored). The sizes of the circles are proportional to the number of data points collected from each country.
Tree and land use
Trees were categorized into five groups: broadleaved evergreen (BE); broadleaved deciduous (BD); evergreen coniferous (EC), deciduous conifers (DC) and shrubs (S). For interception, along with the volume, rainfall duration and intensity were also considered.
Land use under infiltration and runoff were categorized into 15 groups of stands/types: mixed forest (MiF); afforested land (Af); mixed coniferous (MiC); mixed deciduous (MiD); mono fir (MoF); mono spruce (MoS); mono pine (MoP); mono beech MoB); mono oak (MoO); mono broadleaved (MoBr: in the case of a mix of several species, but limited to broadleaved); mono Eucalyptus (MoE); agricultural land (Ag); shrubland (Sh); grassland (Gr) and bare soil (Bs) (S-Table 1 ). To understand the broad differences between broadleaf and conifers, several land uses were grouped together under these two broad categories. ‘Broadleaf’ group includes: MiD; MoO; MoBr and MoE. ‘Conifers’ group includes: MiC; MoF; MoS and MoP.
Normalization
Transpiration rates in mm day −1 was analyzed either as per canopy or plot area. In case of missing values, sapwood area (water conducting xylem tissues within the main stem), diameter at breast height and canopy or plot area were used. Where only flux density was provided, transpiration rate (Ec) was calculated (Eq. 1 ) following Rahman et al. 6 , 14 :
where Js is the daily sap flux density in g H 2 O m −2 s −1 , A s is the sapwood area in cm 2 , and A c is the canopy area in m 2 .
For infiltration, saturated hydraulic conductivity (Ks) values in mm h −1 were used. In cases of several values of tree types or land uses i.e., interception rate for different seasons (leafed/leafless period) or runoff coefficient for different rainfall intensities, the mean value was used to represent the annual rate. When independent variables such as rainfall intensity or leaf area index (LAI) were provided without a corresponding dependent variable, the mean value was used to represent the dataset.
Normalization was applied to runoff coefficient values to adjust the effects of plot size and rainfall intensity. Plot size normalization values were based on Moreno-de las Heras et al. 30 , who conducted runoff experiments on plot sizes with 1, 2, 3 and 15 m (micro-catchment) lengths on five different slopes, three of which were degraded and two were less-degraded. The runoff coefficient values from the results were summed within the same plot length categories and a percentage reduction was calculated (S Table 2 ). Consequently, only runoff coefficient for plot sizes < 1–3 m 2 were normalized to the 15 m length equivalent following Moreno-de las Heras et al. 30 (S-Table 2 ). Plot lengths between 4 and 15 m were not considered. Plot sizes with lengths over 15 m were not normalized. Runoff coefficient values of degraded slopes were applied to bare soils, grassland and shrub land, whereas values of less-degraded slopes to other land uses with plant cover. The normalized runoff coefficient were calculated (Eq. 2 ):
where Rn is the normalized runoff coefficient, R o is the runoff coefficient and r is the percentage reduction as in S-Table 2 .
Rainfall intensity over 45 mm h −1 was normalized following Wu et al. 31 (S-Table 3 ) (Eq. 3 ):
where Rn is the normalized runoff coefficient, R o is the runoff coefficient and r is the increased rate of runoff coefficient from one rainfall intensity to the other.
Slope effects were not normalized since the results of many studies were inconclusive 31 .
The water balance of the rainfall partitioning was estimated as Pg = I + T + R + IR , where Pg is the annual precipitation as 100%, I is the annual interception rate as a ratio to the precipitation (%), T is the annual transpiration rate per canopy area as a ratio of the annual precipitation (%), R is the runoff coefficient (%) and IR is the infiltration rate which cannot be expressed as a ratio to precipitation; therefore, IR was obtained as IR = Pg − ( I + T + R ).
All the 36 studies (S-Table 1 ) considered for runoff coefficient used empirical data. Similarly, all the 19 studies considered for transpiration (S-Table 1 ) used field measured transpiration rates either using leaf porometer, stem flux density or eddy covariance data. For annual precipitation data, the studies used 30 years average annual precipitation from a meteorological station (some studies did not explicitly mention about 30-year average and meteorological stations name). Since transpiration data was mostly collected during the foliage time of the year, we paid attention to the data collection period. All the transpiration data within the investigated studies covered the rainy seasons (higher soil moisture potential), hence, the chance of underestimation is low.
Out of 28 studies (S-Table 1 ) considered for interception, ten used different modelling approaches. Among different models, the Rutter 32 and Gash models 33 are most commonly used for rainfall interception studies. Véliz-Chávez et al. 34 compared these two models, validated the model results with the measured data and reported that the Gash model is more accurate. Among nine modelling studies, six studies namely: Fan et al. 35 ; Ghimire et al. 36 ; Pereira et al. 37 ; Pypker et al. 38 ; Ringgaard et al. 39 ; Price and Carlyle-Moses 40 used a revised Gash model and validated the simulations with measured data. Considering the limitations of the Rutter and Gash models to predict interception and water retention in single trees, Guevara-Escobar et al. 22 used semiariograms as descriptors of through-fall variability for modeling water flux in a surface response. Some studies used liner regression models (e.g., Fathizadeh et al. 41 ) to estimate canopy storage from the relationship between cumulative gross precipitation and through-fall. Livesley et al. 42 used regression analyses between gross rainfall and through-fall and stem flow to calculate interception. Similarly, Xiao and McPherson 43 used a single tree model considering the tree morphological (dimension, leaf surface area) and meteorological data as input to predict interception by different tree species at different stages.
In terms of rainfall amount, 9 out of 28 studies had no cited sources or data were averages from less than 10 years. 13 out of 28 studies have more than 10-years averages, at least 18 years. The remaining studies have cited meteorological data whereby the number of years was not explicitly specified. However, the percentage of interception were all calculated based on the incident precipitation, not on the average annual rainfall. All the studies used measured data except Xiao and McPherson 43 , where the authors used computer simulations using a 25-year rainfall event. For studies with measurement periods of less than 12 months, interception rate was taken either as mentioned in the paper or was calculated relative to the specific months measured.
Data extraction was done using WebPlotDigitizer 44 and data analysis using RStudio (1.3.1073) 45 with packages read_xl; tidyverse; aov; ggplot, cowplot, gpubr and dplyr.
Data were first assessed for normal distribution, and there was no violation of variance homogeneity and later subjected to one or two way ANOVA. To understand the relationship between morphological characteristics or soil physical properties liner regression analyses were carried out. Following García-Palacios et al. 46 and Le Provost et al. 47 , the relative importance (effect) of the influencing factors based on a multivariate regression model was evaluated. Means were reported significant when p < 0.05.
Interception
Annual rainfall amounts showed a strong influence on the interception rate, the lower the rainfall, the higher the values for the interception rate, as for example in arid climate (B). An exception was the climate zone DF (Ontario, Canada). Overall, the interception rate was significantly higher for evergreen coniferous (mean, µ = 39%), followed by broadleaved evergreen (µ = 28%), broadleaved deciduous (µ = 23%) and shrubs (µ = 19%) (p = 7.6e −04 ) (Fig. 2 ). Only in arid climate broadleaved evergreen showed a significantly higher interception rate (µ = 63%) compared to evergreen conifers (µ = 42%). Both climate and vegetation types showed separate (p = 0.001 for climate and p = 6.72e −05 for vegetation type) and interaction effect (p = 0.02) on interception rate.
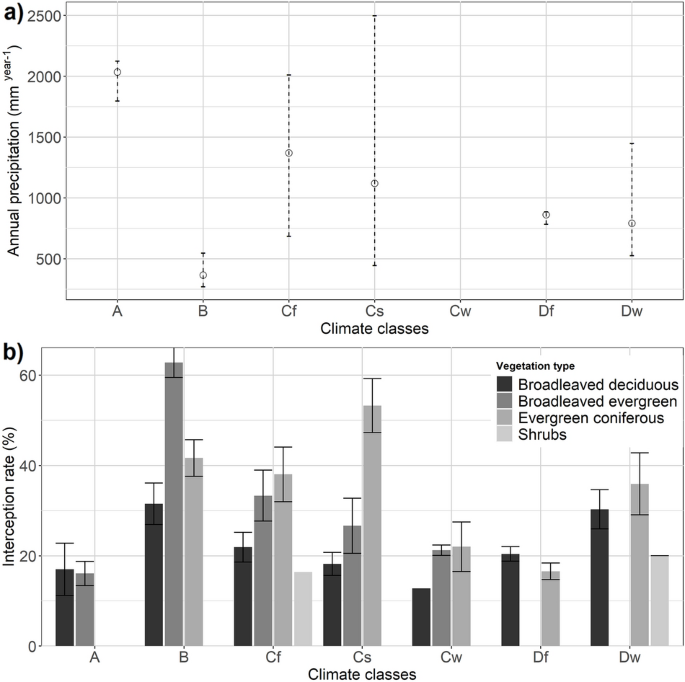
( a ) Range and mean (circle) annual precipitation of different climate zones used in this review. ( b ) Bar chart showing the interception rate of different vegetation types at different climate zones (± SE). Here, A stands for tropical, B for arid, Cf temperate with no dry season, Cs temperate with dry summer, Cw temperate with dry winter, Df continental with no dry season, Dw continental with dry summer and E for polar climate.
Transpiration
Due to the lack of transpiration data in particular across climate zones, only climate zones C and D were compared. The range of transpiration rate varied between 0.1 and 2 mm day −1 at annual scale. The highest mean transpiration was found in Df region (0.7 mm day −1 ) and the lowest in Cs (0.3 mm day −1 ) (Fig. 3 a). In general, coniferous evergreen trees showed the highest mean transpiration rate (0.7 mm day −1 ) compared to broadleaved deciduous trees (0.5 mm day −1 ), shrubs (0.4 mm day −1 ) and broadleaved evergreen (0.2 mm day −1 ) (Fig. 3 b).
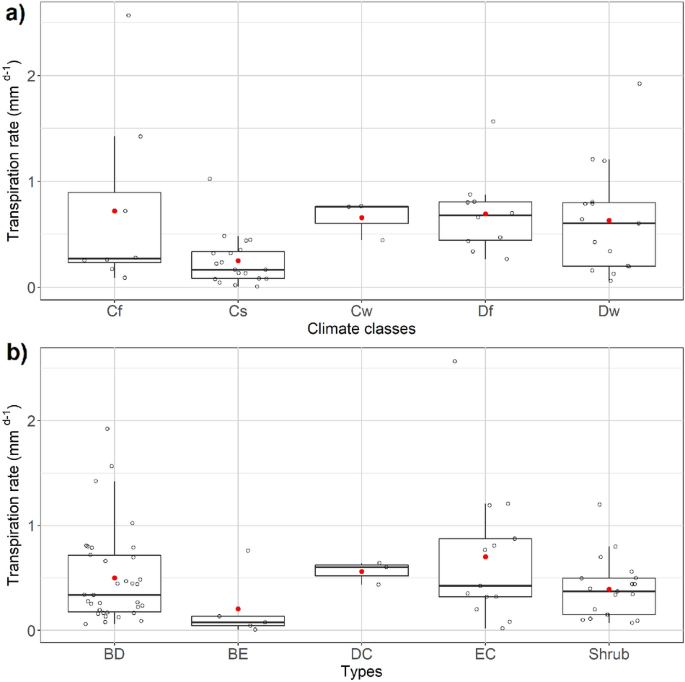
Transpiration rate on an annual scale ( a ) by climate zones; ( b ) by tree types (empty dots show the distribution of individual data points, red dot = mean, dark line = median).
Morphological traits influencing interception and transpiration
Both interception and transpiration rates were significantly related to DBH (p = 0.03, n = 35 for interception and p = 0.01, n = 53 for transpiration), though results were inconsistent. The significance level varied between climate zones, vegetation functional types and between rural and urban sites. LAI showed a consistent pattern over all independent variables (Fig. 4 ).
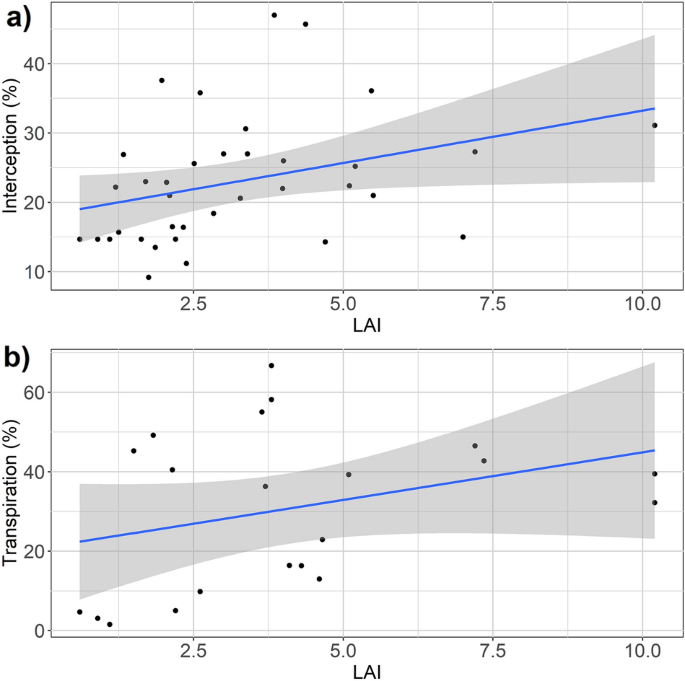
Relationship between leaf area index (LAI) for trees with ( a ) interception rate; ( b ) transpiration rate.

Infiltration, runoff and water balance
Overall, forests showed substantially higher Ks compared to agriculture, shrubs and grasslands (Fig. 5 b). The highest Ks was found in broadleaved stands followed by mixed and coniferous stands and least for afforested stands (Fig. 5 b). Mixed forest, mixed deciduous, spruce, pine and oak stand had the highest Ks at 0–10 cm soil depth, whereas Eucalyptus stand had the highest Ks at 40 cm. Shrub and grassland both indicated negligible Ks over 20–30 cm depth. Bulk density were negatively related to porosity (p = 2.2e −16 ) and in turn, both bulk density and porosity showed significant effect on Ks in particular, within the 0–20 cm soil depth (p = 0.04, n = 122).
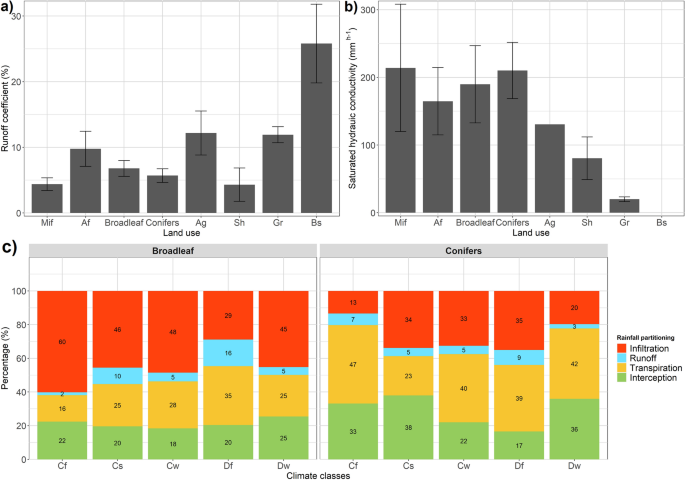
Runoff coefficient ( a ) and saturated hydraulic conductivity ( b ) by land use ( Mif mixed forest, Af afforestation, broadleaf mix broadleaved stands, conifers mix coniferous stands, Ag agriculture, Sh shrubland, Gr grassland, Bs bare soil) and water balance ( c ) as percentage of rainfall by climate zones for broadleaf trees and conifers.
Among all land uses, runoff coefficient of bare soil was the highest (26%), followed by grassland and agricultural land (Fig. 5 a). Among the forest stands, mixed forest had the lowest runoff coefficient followed by shrubs, broadleaved and conifer stands and afforested land (Fig. 5 a). There was a large variation within the groups. The values of the coniferous species, ranged from 2.0% for spruce stands to 10.3% for fir stands, the values of broadleaved species ranged from 1.4% for oak stands to 17.3% for mixed deciduous stands.
Considering the annual rainfall partitioning and the climate zones, runoff coefficient was lower under coniferous (3–9%) than broadleaved stands (2–16%) (Fig. 5 c). Interception was the most influencing mechanism within coniferous stands, in particular within climate zone Cs (temperate with dry summer). Contrarily, within the broadleaved stands transpiration was the most influencing mechanism in continental climate with no dry season (Df). Regarding soil infiltration, broadleaved showed the highest potential (up to 60%) compared to the coniferous stands (up to 35%).
Relative effect sizes
To evaluate the relative importance of the influencing factors, we expressed their effect as the percentage of variance they explain, based on the comparison between the absolute values of their standardized regression coefficients and the sum of all standardized regression coefficients. The parameter estimates of the model predictors are shown with their associated standard errors along with the relative importance of each predictor (Fig. 6 ). This method is similar to a variance partitioning analysis since we previously transformed all factors to z-scores.
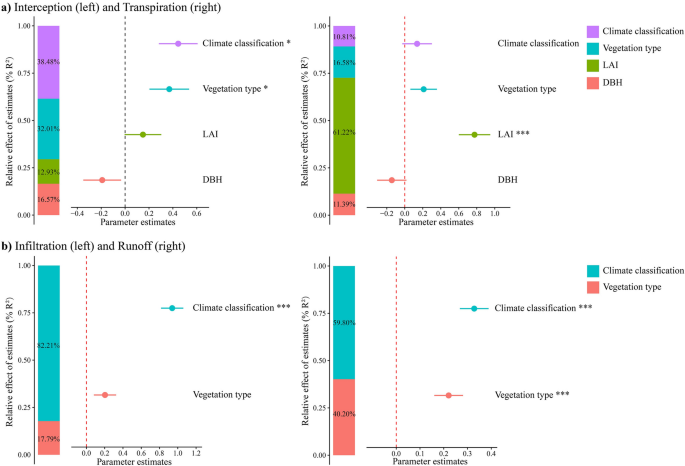
Relative effect of variables: climate, vegetation type, LAI and DBH on ( a ) Interception and transpiration; ( b ) Infiltration and runoff coefficient (* p < 0.05, *** p < 0.001).
Apart from DBH, all other factors positively influenced interception and transpiration. Vegetation types and climate had the biggest influence on interception, whereas for transpiration, LAI was the single most important factor followed by vegetation types, DBH and climate. For infiltration and runoff, climate showed the largest impact. However, vegetation types was significant for runoff coefficient, not for infiltration though.
Climate plays a significant role in overall storm-water management, and therefore, the relative effect size is mostly higher than the vegetation types (Fig. 6 ). For example, while comparing the runoff coefficient of similar vegetation types (mono-pine stand) at Cw (temperate with dry winter—mean annual precipitation 1370 mm) showed significantly less runoff compared to Cf (Temperate with no dry season—1270 mm) (S-Fig. 1 ).
Comparative analyses of tree species-specific traits excluding climatic variables are difficult since they directly influence the storm-water partitioning mechanisms. At the same time, data availability for statistical analysis on tree traits is low for identifying particular mechanisms. However, the analyses of specific traits and particular rainfall partitioning mechanisms yielded important insights such as the relative strength of DBH and foliage density on transpiration and interception, crown shape on interception, leaf surface hydrophobicity on interception and rooting depth on soil infiltration (Table 1 ). For the two biggest components of precipitation partitioning, interception and transpiration, higher foliage density and growth showed the most positive relationship both for conifer and broadleaved species. Every unit of LAI increment revealed an additional 5% rainfall partition through transpiration (3%) and interception (2%). At the same time, site conditions such as tree arrangement displayed counterintuitive results as more open arrangements resulted in higher interception and transpiration rates, while more cluster like arrangements showed an increase in mean soil hydraulic conductivity and a reduction of runoff. Similarly, older trees are mostly important for all the mechanisms of rainfall partitioning except transpiration rate.
Storm-water control components of rainfall partitioning are interception, transpiration and infiltration, while runoff is the residual from the total precipitation. Climate, vegetation types as well as particular tree traits all influence rainfall partioning separately or conjointly. Concerning the vegetation types, trees with their developed canopy and root systems that increases interception and infiltration 48 , undoubtedly offer the best solution to control storm-water runoff. Oliveira et al. 49 found that removing matured trees had the potential to increase runoff up to five fold while analyzing the conversion of an undisturbed forest in Mexico to pasture and croplands, and up to 20 fold under bare soil conditions. However, studies also found that the runoff decreases as vegetation mature 50 , 51 . Conifers tend to have lower antecedent soil water content due to higher interception and transpiration rate resulting in drier soil conditions compared to broadleaf stands, delaying the runoff initiation time and increasing its water holding capacity 52 . All these characteristics suggest that the optimal solution would be the presence of mixed vegetation strata for reducing storm-water runoff. Apparently, only transpiration per unit area decreases; interception, infiltration, increases and runoff decreases with increasing stem density (S-Fig. 2 ).
Climate effect
Interception is one of the largest components of rainfall partitioning. Sadeghi et al. 53 showed an inverse relationship of interception with rainfall intensity and duration; although investigated in non-urban settings, the results could be transferred to urban settings. Subsequently, the highest interception in arid regions and the lowest in the tropical regions. On the other hand, evergreen species with their leaves all year round showed higher interception percentage on annual basis within the climate zones with wet winter such as Cs. Urban trees with comparatively unrestricted crown diameter 54 and higher vapour pressure deficit 55 might allure higher retention of rainfall than their rural counterpart. However, interception rate can be less than 10% for storms with depths over 20 mm and rainfall intensity over 7.6 mm h −1 56 . Additionally, wind direction and velocity are important since they influence the saturation phase 22 .
Transpiration, another significant component of rainfall partitioning, is more dependent on the soil moisture availability and the rainless higher atmospheric demand situations 57 such as in the Cf and Df climate zones (with no dry season). Similarly, transpiration from coniferous even within the wet winter climate zones did not show higher amount annually since the most active transpiration takes place during the summer 9 . Although higher amounts of precipitation showed higher transpiration, the trend of soil moisture and tree transpiration is still inconclusive 58 . Moreover, the genetic constituents of different trees with deeper rooting 1 and stomatal regulations during summer drought 59 makes it difficult to generalize climatic influence on tree transpiration.
Vegetation types
The intercepted water mostly comes to the soil and this is soil hydraulic conductivity (Ks), which dictates the amount of available moisture for the rooting system for the subsequent transpiration rate. K s is a complex issue due to the combination of anthropological and geomorphic processes 60 . Woodlands in general have higher Ks compared to agricultural and other land use types. Grassland and shrub land could potentially infiltrate water faster, but had the tendency to saturate faster due to shallow root systems, whereas forest soils infiltrated water slower but had deeper water table. Similarly, conifers such as spruce and pine have shallow rooting system, whilst broadleaf’s such as beech and oaks have deeper rooting system 50 , 52 . Species with shallow rooting systems did not show much differences at 0–10 cm, but started to slow down further below (S-Fig. 3 ). This has two big implications, in particular, for cities with higher soil bulk density at the top soil layer, since Ks was found to be negatively related to the bulk density (S-Fig. 4 ). Any type of vegetation would increase the macro-porosity and reduce the bulk density to at least delay the storm water runoff. On long-terms, broadleaf trees might be better in increasing Ks through deeper rooting system and higher faunal activity through litter fall, which is less acidic than the needle leaves 27 . Evergreen conifers could transpire more compared to deciduous 61 per annum in particular in temperate regions; however, with low winter precipitation such as New Zealand or Japan, there is no significant difference between annual evapotranspiration from broadleaved and coniferous trees 62 .
Species characteristics
In general, conifers with higher LAI, leaf area density (LAD), smaller mean distance between leaves as well as less steep leaf angle can intercept more than broadleaved 63 . Among morphological characteristics, DBH and LAI showed the most consistent influence on interception rate. At large, DBH shows a symmetric relationship with growth 64 and LAI is widely used in quantifying the foliage retention properties 35 , 65 . Different crown architecture and leaf characteristics also effect the interception rate (Table 1 ). For instance, an umbrella-shaped canopy tree ( Sophora japonica ) can intercept more 66 than funnel-shaped canopy tree ( Acer truncatum ) that would direct more water as stem flow instead of dripping off as through-fall 63 . At the same time, trees with higher hydrophobicity (e.g. Broussonetia papyrifera ) were found to have low interception rate 62 compared to rigid and rough leaf surface such as Pistacia chinensis 63 , 67 . Similarly, trees with higher LAD such as Fagus grandifolia can capture inclined rainfall more efficiently, whereas a greater horizontal and shallower surface canopy i.e. Liriodendron tulipifera can capture uninclined droplets more efficiently 68 . The relationship between transpiration and xylem anatomy or sap wood area as well as DBH was inconclusive. The consistent finding was the positive relationship between LAI and transpiration.
Despite mounting evidence of the benefits of trees in storm-water management, there are still limitations to this area of study, particularly in urban settings, where many of the rainfall partitioning data had to be filled in with studies from rural settings. Apart from the limitation of data from rural settings, most studies were confined in North America and Europe, generally in the temperate climate zone (C). In particular, there is a great paucity of transpiration data for tropical (A), arid (B) and polar regions (E). Numerical assessment of urban trees in storm-water management is mostly based on rough estimations 69 such as in a review by the Centre for Watershed Protection 70 . They found only six studies, three of which used measured data from a single plot, the other three used models. Moreover, tree traits such as leaf area, age or site conditions as e.g. soil 71 were not considered in most of the studies. Even though standardization was done for better comparability; however, in many cases data were collected at a particular time of the year, which might bias the results. For example, some studies reported interception loss only during the rainy season or transpiration not covering the entire growing season. Additionally, the reviewed studies lack standardized study protocols, methodologies, and addition of site descriptions, relevant morphological and anatomical data especially in tropical, sub-tropical and polar region that could have increased the comparability of studies. Overall, runoff studies in urban settings were limited to the effect of vegetation coverage on runoff, but no empirical study was carried out to compare different functional types of urban vegetation. Results from studies in natural settings should only be applied with great caution in the urban settings as the latter differ in vegetation types, let alone the micro-climatic variations. Moreover, most of the vegetation within urban settings are confined to small growth pits and subjected to soil compaction. Future research incorporating arboricultural (e.g. maintenance practices of individual trees), canopy hydrological processes can help to better calibrate hydrologic models to inform urban planning 69 . In order to avoid ambiguity in terms of morphological characteristics provided in the studies such as foliage density in terms of crown density, one sided leaf area, plant area index, alternative approaches like runoff avoided from volume per unit canopy area should be standardized. Finally, further studies across climate zones with standardized methods of rainfall partitioning mechanisms with different tree traits would allow more accurate and specific treatment to be applied for data normalization for more robust meta-analysis in future.
Tree selection for storm-water management should focus on the interception and transpiration partitioning as they are the “first line of defense”, simply because trees in urban watersheds can restore natural hydrologic regimes by higher amounts of interception, transpiration, and infiltration, and consequently, delay of runoff and capture of storm water compared to other types of vegetation 18 . Present selection of species for green infrastructure planning is largely biased by only few species, predominantly broadleaved deciduous trees, at-least in the temperate central Europe 72 . Recent analyses of tree cadasters of 44 central European cities revealed only 5% of conifers within the tree assemblage 73 . The review showed that mixed cultures would be the best solution, but the recipe can vary according to climate zone. In the temperate climate zone with dry summers, a higher proportion of evergreen species (30%) and climate zones with dry winter or no dry season, a higher proportion of deciduous (80%) also with tropical or riparian origin can ultimately lead to lower runoff (Fig. 7 ). Keeping all other factors constant, the proposed mix can further reduce runoff by around 4%. Within the tropical climate zone, where a foliage-free period is not that pronounced, trees with high LAI, deep and coarse root system can be recommended. In case of arid climates, the focus should be more towards the collection of water for better tree growth. Therefore, species with hydrophobic leaf surfaces, inclined branches (more conical crown) to affluent stem flow as well as deep-rooted species to withstand summer drought would be better.
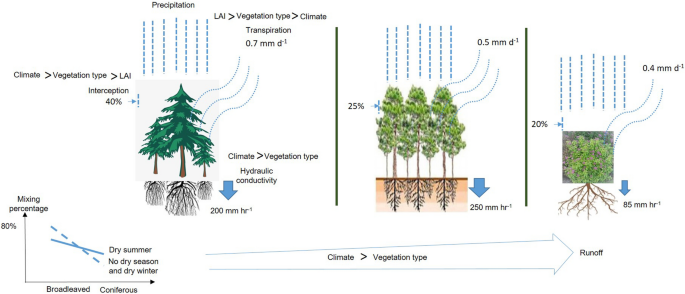
Schematic diagram of major rainfall partitioning pathways by different vegetation types along with the relative effect sizes of variables, consequent runoff coefficient and the proposed species mix for climate zones with distinctive precipitation patterns.
With ongoing urbanization and climate change, storm water management has become increasingly important. More attention has been directed towards selection of species or variety of species for planting over hardscaped surfaces such as streets, parking lots or in urban parks having varying impacts on storm water management. Shallow rooted vegetation types including shrubs and grasslands can effectively reduce runoff; however, as soon as the rainfall depth and intensity increases, runoff increases linearly. Thus, big stature vegetation such as trees could potentially intercept more, endure higher stem and through-fall, and improve the soil conditions for increasing water infiltration and transpiration. Among the major rainfall partitioning mechanisms, we have shown that the interception rate is higher in areas with less rainfall but transpiration rate is minimal. Within the vegetation types, conifers showed higher amounts of interception and transpiration water loss at annual scale compared to broadleaved trees, but the soil infiltration rate was higher under the canopies of broadleaved species. Regarding the species traits, canopy density was positively related to both interception and transpiration water loss. Therefore, increasing a mix of conifer and broadleaved species within urban settings will maximise the reduction of storm-water runoff, especially in climate zones with significant winter precipitation or with no distinct dry seasons. However, GI strategies with a narrow focus have practical limits and might not be the most cost-effective option in particular considering the vegetation and land use types along with climate. Therefore, mixed species selection along with shrubs and grasslands to increase the overall functional diversity of greenspaces can facilitate storm-water management. Nevertheless, water attenuation benefits of urban forests are related to a range of ecosystem services, thus, other important regulating (i.e., cooling, air pollution removal, carbon storage); provisioning and cultural services as well as potential disservices such as storm breakage, snow breakage, allergenicity should be taken into account before the final selection. Future comparative research following a standardized protocol can help to understand the broad range of hydrological impacts of diversified urban vegetation across climate regimes.
Data availability
Data will be available upon request to the corresponding author.
Rahman, M. A. et al. Comparing the infiltration potentials of soils beneath the canopies of two contrasting urban tree species. Urban For. Urban Green. 38 , 22–32. https://doi.org/10.1016/j.ufug.2018.11.002 (2019).
Article Google Scholar
Zölch, T., Henze, L., Keilholz, P. & Pauleit, S. Regulating urban surface runoff through nature-based solutions - An assessment at the micro-scale. Environ. Res. 157 , 135–144. https://doi.org/10.1016/j.envres.2017.05.023 (2017).
Article CAS Google Scholar
Barron, O. V., Barr, A. D. & Donn, M. J. Effect of urbanisation on the water balance of a catchment with shallow groundwater. J. Hydrol. 485 , 162–176. https://doi.org/10.1016/j.jhydrol.2012.04.027 (2013).
Article ADS Google Scholar
Rosenzweig, B. R. et al. The value of urban flood modeling. Earth’s Future 9 , e2020EF001739. https://doi.org/10.1029/2020EF001739 (2021).
Pauleit, S., Fryd, O., Backhaus, A. & Jensen, M. B. In Encyclopedia of Sustainability Science and Technology (ed. Meyers, R. A.) 1–29 (Springer, 2020).
Google Scholar
Rahman, M. A. et al. Traits of trees for cooling urban heat islands: A meta-analysis. Build. Environ. 170 , 106606. https://doi.org/10.1016/j.buildenv.2019.106606 (2020).
Ziter, C. D., Pedersen, E. J., Kucharik, C. J. & Turner, M. G. Scale-dependent interactions between tree canopy cover and impervious surfaces reduce daytime urban heat during summer. Proc. Natl. Acad. Sci. USA 116 , 7575–7580. https://doi.org/10.1073/pnas.1817561116 (2019).
Article ADS CAS Google Scholar
Waldrop, M. M. News feature: The quest for the sustainable city. Proc. Natl. Acad. Sci. 116 , 17134–17138. https://doi.org/10.1073/pnas.1912802116 (2019).
Cleugh, H. A., Bui, E., Simon, D., Xu, J. & Mitchell, V. G. The Impact of Suburban Design on Water Use and Microclimate (2005).
Chan, F. K. S. et al. “Sponge City” in China—A breakthrough of planning and flood risk management in the urban context. Land Use Policy 76 , 772–778. https://doi.org/10.1016/j.landusepol.2018.03.005 (2018).
Morgan, R. P. C. Soil Erosion and Conservation (Wiley, 2005).
Xu, C. et al. Surface runoff in urban areas: The role of residential cover and urban growth form. J. Clean. Prod. 262 , 121421. https://doi.org/10.1016/j.jclepro.2020.121421 (2020).
Ostoić, S. K. & van den Bosch, C. C. K. Exploring global scientific discourses on urban forestry. Urban For. Urban Green. 14 , 129–138. https://doi.org/10.1016/j.ufug.2015.01.001 (2015).
Rahman, M. A. et al. Tree cooling effects and human thermal comfort under contrasting species and sites. Agric. For. Meteorol. 287 , 107947. https://doi.org/10.1016/j.agrformet.2020.107947 (2020).
Rötzer, T., Rahman, M. A., Moser-Reischl, A., Pauleit, S. & Pretzsch, H. Process based simulation of tree growth and ecosystem services of urban trees under present and future climate conditions. Sci. Total Environ. 676 , 651–664. https://doi.org/10.1016/j.scitotenv.2019.04.235 (2019).
Grote, R. et al. Functional traits of urban trees: Air pollution mitigation potential. Front. Ecol. Environ. 14 , 543–550. https://doi.org/10.1002/fee.1426 (2016).
Pace, R. et al. A single tree model to consistently simulate cooling, shading, and pollution uptake of urban trees. Int. J. Biometeorol. 65 , 277–289. https://doi.org/10.1007/s00484-020-02030-8 (2021).
Kuehler, E., Hathaway, J. & Tirpak, A. Quantifying the benefits of urban forest systems as a component of the green infrastructure stormwater treatment network. Ecohydrology https://doi.org/10.1002/eco.1813 (2017).
Rahman, M. A., Moser, A., Gold, A., Rötzer, T. & Pauleit, S. Vertical air temperature gradients under the shade of two contrasting urban tree species during different types of summer days. Sci. Total Environ. 633 , 100–111. https://doi.org/10.1016/j.scitotenv.2018.03.168 (2018).
Rahman, M. A., Smith, J. G., Stringer, P. & Ennos, A. R. Effect of rooting conditions on the growth and cooling ability of Pyrus calleryana . Urban For. Urban Green. 10 , 185–192. https://doi.org/10.1016/j.ufug.2011.05.003 (2011).
Schellekens, J., Scatena, F. N., Bruijnzeel, L. A. & Wickel, A. J. Modelling rainfall interception by a lowland tropical rain forest in northeastern Puerto Rico. J. Hydrol. 225 , 168–184. https://doi.org/10.1016/S0022-1694(99)00157-2 (1999).
Guevara-Escobar, A., González-Sosa, E., Véliz-Chávez, C., Ventura-Ramos, E. & Ramos-Salinas, M. Rainfall interception and distribution patterns of gross precipitation around an isolated Ficus benjamina tree in an urban area. J. Hydrol. 333 , 532–541. https://doi.org/10.1016/j.jhydrol.2006.09.017 (2007).
Xiao, Q. F. & McPherson, E. G. Surface water storage capacity of twenty tree species in Davis, California. J. Environ. Qual. 45 , 188–198. https://doi.org/10.2134/jeq2015.02.0092 (2016).
Xiao, Q. F., McPherson, E. G., Ustin, S. L. & Grismer, M. E. A new approach to modeling tree rainfall interception. J. Geophys. Res. Atmos. 105 , 29173–29188. https://doi.org/10.1029/2000jd900343 (2000).
Carlyle-Moses, D. E. & Gash, J. H. C. In Forest Hydrology and Biogeochemistry: Synthesis of Past Research and Future Directions (eds Levia, D. F. et al. ) 407–423 (Springer, 2011).
Chapter Google Scholar
Hirano, T. et al. The difference in the short-term runoff characteristic between the coniferous catchment and the deciduous catchment: The effects of storm size on storm generation processes of small forested catchment. J. Jpn. Soc. Hydrol. Water Resour. 22 , 24–39. https://doi.org/10.3178/jjshwr.22.24 (2009).
Chandler, K. R. & Chappell, N. A. Influence of individual oak ( Quercus robur ) trees on saturated hydraulic conductivity. For. Ecol. Manage. 256 , 1222–1229. https://doi.org/10.1016/j.foreco.2008.06.033 (2008).
Stewart, I. D. A systematic review and scientific critique of methodology in modern urban heat island literature. Int. J. Climatol. 31 , 200–217. https://doi.org/10.1002/joc.2141 (2011).
Beck, H. E. et al. Present and future Köppen-Geiger climate classification maps at 1-km resolution. Sci. Data 5 , 180214. https://doi.org/10.1038/sdata.2018.214 (2018).
Moreno-de las Heras, M., Nicolau, J. M., Merino-Martín, L. & Wilcox, B. P. Plot-scale effects on runoff and erosion along a slope degradation gradient. Water Resour. Res. 46 , W04503. https://doi.org/10.1029/2009WR007875 (2010).
Wu, L., Peng, M., Qiao, S. & Ma, X.-Y. Effects of rainfall intensity and slope gradient on runoff and sediment yield characteristics of bare loess soil. Environ. Sci. Pollut. Res. 25 , 3480–3487. https://doi.org/10.1007/s11356-017-0713-8 (2018).
Rutter, A. J., Kershaw, K. A., Robins, P. C. & Morton, A. J. A predictive model of rainfall interception in forests, 1. Derivation of the model from observations in a plantation of Corsican pine. Agric. Meteorol. 9 , 367–384. https://doi.org/10.1016/0002-1571(71)90034-3 (1971).
Gash, J. H. C. An analytical model of rainfall interception by forests. Q. J. R. Meteorol. Soc. 105 , 43–55. https://doi.org/10.1002/qj.49710544304 (1979).
Véliz-Chávez, C., Mastachi-Loza, C. A., Gonzalez-Sosa, E., Becerril-Pia, R. & Ramos-Salinas, N. M. Canopy storage implications on interception loss modeling. Am. J. Plant Sci. 05 , 3032–3048. https://doi.org/10.4236/ajps.2014.520320 (2014).
Fan, J., Oestergaard, K. T., Guyot, A. & Lockington, D. A. Measuring and modeling rainfall interception losses by a native Banksia woodland and an exotic pine plantation in subtropical coastal Australia. J. Hydrol. 515 , 156–165. https://doi.org/10.1016/j.jhydrol.2014.04.066 (2014).
Ghimire, C. P., Bruijnzeel, L. A., Lubczynski, M. W. & Bonell, M. Rainfall interception by natural and planted forests in the Middle Mountains of Central Nepal. J. Hydrol. 475 , 270–280. https://doi.org/10.1016/j.jhydrol.2012.09.051 (2012).
Pereira, F. L. et al. Modelling interception loss from evergreen oak Mediterranean savannas: Application of a tree-based modelling approach. Agric. For. Meteorol. 149 , 680–688. https://doi.org/10.1016/j.agrformet.2008.10.014 (2009).
Pypker, T. G., Bond, B. J., Link, T. E., Marks, D. & Unsworth, M. H. The importance of canopy structure in controlling the interception loss of rainfall: Examples from a young and an old-growth Douglas-fir forest. Agric. For. Meteorol. 130 , 113–129. https://doi.org/10.1016/j.agrformet.2005.03.003 (2005).
Ringgaard, R., Herbst, M. & Friborg, T. Partitioning forest evapotranspiration: Interception evaporation and the impact of canopy structure, local and regional advection. J. Hydrol. 517 , 677–690. https://doi.org/10.1016/j.jhydrol.2014.06.007 (2014).
Price, A. G. & Carlyle-Moses, D. E. Measurement and modelling of growing-season canopy water fluxes in a mature mixed deciduous forest stand, southern Ontario, Canada. Agric. For. Meteorol. 119 , 69–85. https://doi.org/10.1016/S0168-1923(03)00117-5 (2003).
Fathizadeh, O., Hosseini, S. M., Zimmermann, A., Keim, R. F. & Darvishi Boloorani, A. Estimating linkages between forest structural variables and rainfall interception parameters in semi-arid deciduous oak forest stands. Sci. Total Environ. 601–602 , 1824–1837. https://doi.org/10.1016/j.scitotenv.2017.05.233 (2017).
Livesley, S. J., Baudinette, B. & Glover, D. Rainfall interception and stem flow by eucalypt street trees—the impacts of canopy density and bark type. Urban For. Urban Green. 13 , 192–197. https://doi.org/10.1016/j.ufug.2013.09.001 (2014).
Xiao, Q. & McPherson, E. G. Rainfall interception by Santa Monica’s municipal urban forest. Urban Ecosyst. 6 , 291–302. https://doi.org/10.1023/B:UECO.0000004828.05143.67 (2002).
Rohatgi, A. WebPlotDigitizer (4.4) , 2020).
Team, R. C. (R Foundation for Statistical Computing, 2020).
García-Palacios, P., Gross, N., Gaitán, J. & Maestre, F. T. Climate mediates the biodiversity–ecosystem stability relationship globally. PNAS 115 , 8400–8405. https://doi.org/10.1073/pnas.1800425115 (2018).
Le Provost, G. et al. Land-use history impacts functional diversity across multiple trophic groups. PNAS 117 , 1573–1579. https://doi.org/10.1073/pnas.1910023117 (2020).
El Kateb, H., Zhang, H., Zhang, P. & Mosandl, R. Soil erosion and surface runoff on different vegetation covers and slope gradients: A field experiment in Southern Shaanxi Province, China. CATENA 105 , 1–10. https://doi.org/10.1016/j.catena.2012.12.012 (2013).
Oliveira, P. T. S. et al. The water balance components of undisturbed tropical woodlands in the Brazilian cerrado. Hydrol. Earth Syst. Sci. 19 , 2899–2910. https://doi.org/10.5194/hess-19-2899-2015 (2014).
Hümann, M. et al. Identification of runoff processes – The impact of different forest types and soil properties on runoff formation and floods. J. Hydrol. 409 , 637–649. https://doi.org/10.1016/j.jhydrol.2011.08.067 (2011).
Sun, D. et al. Soil erosion and water retention varies with plantation type and age. For. Ecol. Manage. 422 , 1–10. https://doi.org/10.1016/j.foreco.2018.03.048 (2018).
Jost, G., Schume, H., Hager, H., Markart, G. & Kohl, B. A hillslope scale comparison of tree species influence on soil moisture dynamics and runoff processes during intense rainfall. J. Hydrol. 420–421 , 112–124. https://doi.org/10.1016/j.jhydrol.2011.11.057 (2012).
Sadeghi, S. M. M., Attarod, P., Van Stan, J. T. & Pypker, T. G. The importance of considering rainfall partitioning in afforestation initiatives in semiarid climates: A comparison of common planted tree species in Tehran, Iran. Sci. Total Environ. 568 , 845–855. https://doi.org/10.1016/j.scitotenv.2016.06.048 (2016).
Pretzsch, H. et al. Climate change accelerates growth of urban trees in metropolises worldwide. Sci. Rep. https://doi.org/10.1038/s41598-017-14831-w (2017).
Rahman, M. A., Moser, A., Rötzer, T. & Pauleit, S. Microclimatic differences and their influence on transpirational cooling of Tilia cordata in two contrasting street canyons in Munich, Germany. Agric. For. Meteorol. 232 , 443–456. https://doi.org/10.1016/j.agrformet.2016.10.006 (2017).
Nytch, C. J., Meléndez-Ackerman, E. J., Pérez, M. E. & Ortiz-Zayas, J. R. Rainfall interception by six urban trees in San Juan, Puerto Rico. Urban Ecosyst. 22 , 103–115. https://doi.org/10.1007/s11252-018-0768-4 (2018).
Rahman, M. A. et al. Comparative analysis of shade and underlying surfaces on cooling effect. Urban For. Urban Green. 63 , 127223. https://doi.org/10.1016/j.ufug.2021.127223 (2021).
Chen, L., Zhang, Z. & Ewers, B. E. Urban tree species show the same hydraulic response to vapor pressure deficit across varying tree size and environmental conditions. PLoS One https://doi.org/10.1371/journal.pone.0047882 (2012).
Moser-Reischl, A., Rahman, M. A., Pauleit, S., Pretzsch, H. & Rötzer, T. Growth patterns and effects of urban micro-climate on two physiologically contrasting urban tree species. Landsc. Urban Plan. 183 , 88–99. https://doi.org/10.1016/j.landurbplan.2018.11.004 (2019).
Hao, M. et al. Impacts of changes in vegetation on saturated hydraulic conductivity of soil in subtropical forests. Sci. Rep. 9 , 8372. https://doi.org/10.1038/s41598-019-44921-w (2019).
Peters, E. B., McFadden, J. P. & Montgomery, R. A. Biological and environmental controls on tree transpiration in a suburban landscape. J. Geophys. Res. Biogeosci. https://doi.org/10.1029/2009jg001266 (2010).
Komatsu, H., Kume, T. & Otsuki, K. Increasing annual runoff—broadleaf or coniferous forests?. Hydrol. Process. 25 , 302–318. https://doi.org/10.1002/hyp.7898 (2011).
Li, X. et al. Process-based rainfall interception by small trees in Northern China: The effect of rainfall traits and crown structure characteristics. Agric. For. Meteorol. 218–219 , 65–73. https://doi.org/10.1016/j.agrformet.2015.11.017 (2016).
Lukaszkiewicz, J. & Kosmala, M. Determining the age of streetside trees with diameter at breast height-based multifactorial model. Arboricult. Urban For. 34 , 137–143. https://doi.org/10.48044/jauf.2008.018 (2008).
Buttle, J. M. & Farnsworth, A. G. Measurement and modeling of canopy water partitioning in a reforested landscape: The Ganaraska Forest, southern Ontario, Canada. J. Hydrol. 466–467 , 103–114. https://doi.org/10.1016/j.jhydrol.2012.08.021 (2012).
Yang, B., Lee, D. K., Heo, H. K. & Biging, G. The effects of tree characteristics on rainfall interception in urban areas. Landsc. Ecol. Eng. 15 , 289–296. https://doi.org/10.1007/s11355-019-00383-w (2019).
Klamerus-Iwan, A. & Witek, W. Variability in the Wettability and Water Storage Capacity of Common Oak Leaves (Quercus robur L). Water 10 , 695. https://doi.org/10.3390/w10060695 (2018).
Van Stan, J. T., Siegert, C. M., Levia, D. F. & Scheick, C. E. Effects of wind-driven rainfall on stemflow generation between codominant tree species with differing crown characteristics. Agric. For. Meteorol. 151 , 1277–1286. https://doi.org/10.1016/j.agrformet.2011.05.008 (2011).
Selbig, W. R. et al. Quantifying the stormwater runoff volume reduction benefits of urban street tree canopy. Sci. Total Environ. 806 , 151296. https://doi.org/10.1016/j.scitotenv.2021.151296 (2022).
Centre for Watershed Protection. Review of the Available Literature and Data on the Runoff and Pollutant Removal Capabilities of Urban Trees (Center for Watershed Protection, 2017).
Berland, A. et al. The role of trees in urban stormwater management. Landsc. Urban Plan. 162 , 167–177. https://doi.org/10.1016/j.landurbplan.2017.02.017 (2017).
Pauleit, S. Urban street tree plantings: Indentifying the key requirements. Proc. Inst. Civ. Eng. Municipal Eng. 156 , 43–50. https://doi.org/10.1680/muen.2003.156.1.43 (2003).
Weller, M. Tree Inventory Data of Central European Cities—Studies on the Composition and Structure of Urban Tree Populations and Derivation of Ecosystem Services . MSC thesis, Technical University of Munich, Germany (2021).
Download references
Acknowledgements
Thanks go to the German Research Foundation (Deutsche Forschungsgemeinschaft) for providing funds for the projects PR 292/21-1 and PA 2626/3-1 ‘Impact of trees on the urban microclimate under climate change: mechanisms and ecosystem services of urban tree species in temperate, Mediterranean and arid major cities’.
Open Access funding enabled and organized by Projekt DEAL.
Author information
Authors and affiliations.
Strategic Landscape Planning and Management, School of Life Sciences, Weihenstephan, Technische Universität München, Emil-Ramann-Str. 6, 85354, Freising, Germany
Mohammad A. Rahman, Yanin Pawijit & Stephan Pauleit
Research Center for Eco-Environmental Engineering, Dongguan University of Technology, Daxue Road 1, Dongguan, 523808, China
Forest Growth and Yield Science, School of Life Sciences, Weihenstephan, Technische Universität München, Hans-Carl-von-Carlowitz-Platz 2, 85354, Freising, Germany
Astrid Moser-Reischl, Hans Pretzsch & Thomas Rötzer
You can also search for this author in PubMed Google Scholar
Contributions
M.R. conceptualized the study. M.R. and Y.P. collected the data and conducted the analysis with the help from C.X. and A.M. and produced the figures. M.R., A.M., H.P., T.R. and S.P. acquired the funding. M.R. wrote the manuscript with the help of all authors.
Corresponding author
Correspondence to Mohammad A. Rahman .
Ethics declarations
Competing interests.
The authors declare that they have no known competing financial interests or personal relationships that could have appeared to influence the work reported in this paper.
Additional information
Publisher's note.
Springer Nature remains neutral with regard to jurisdictional claims in published maps and institutional affiliations.
Supplementary Information
Supplementary information., rights and permissions.
Open Access This article is licensed under a Creative Commons Attribution 4.0 International License, which permits use, sharing, adaptation, distribution and reproduction in any medium or format, as long as you give appropriate credit to the original author(s) and the source, provide a link to the Creative Commons licence, and indicate if changes were made. The images or other third party material in this article are included in the article's Creative Commons licence, unless indicated otherwise in a credit line to the material. If material is not included in the article's Creative Commons licence and your intended use is not permitted by statutory regulation or exceeds the permitted use, you will need to obtain permission directly from the copyright holder. To view a copy of this licence, visit http://creativecommons.org/licenses/by/4.0/ .
Reprints and permissions
About this article
Cite this article.
Rahman, M.A., Pawijit, Y., Xu, C. et al. A comparative analysis of urban forests for storm-water management. Sci Rep 13 , 1451 (2023). https://doi.org/10.1038/s41598-023-28629-6
Download citation
Received : 08 February 2022
Accepted : 20 January 2023
Published : 26 January 2023
DOI : https://doi.org/10.1038/s41598-023-28629-6
Share this article
Anyone you share the following link with will be able to read this content:
Sorry, a shareable link is not currently available for this article.
Provided by the Springer Nature SharedIt content-sharing initiative
By submitting a comment you agree to abide by our Terms and Community Guidelines . If you find something abusive or that does not comply with our terms or guidelines please flag it as inappropriate.
Quick links
- Explore articles by subject
- Guide to authors
- Editorial policies
Sign up for the Nature Briefing newsletter — what matters in science, free to your inbox daily.

Theses and Dissertations Collection
Open Access Repository of University of Idaho Graduate ETD
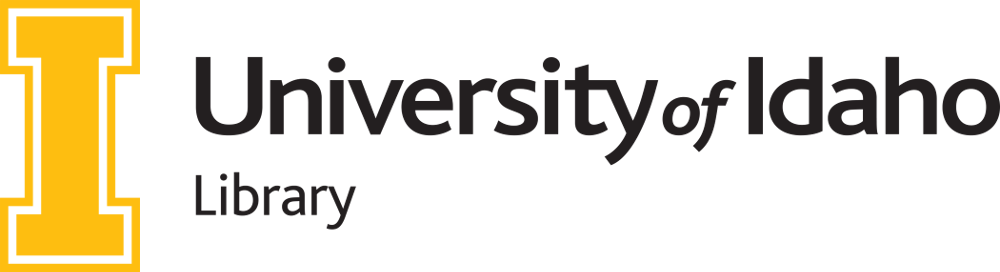
Prescribed Streamflow to Improve Juvenile Steelhead Habitat
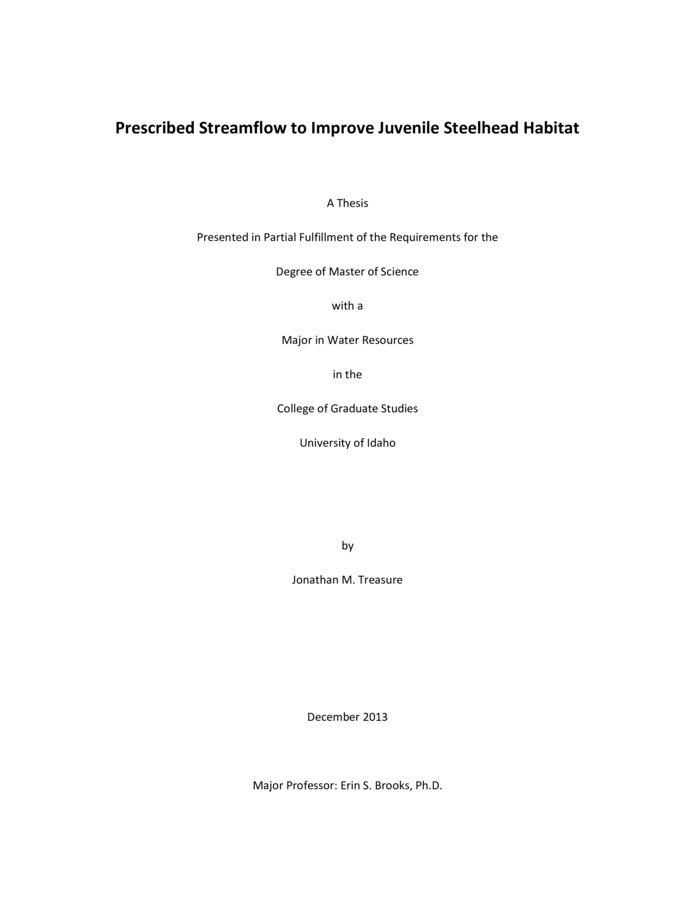
Treasure, Jonathan Michael. (2013). Prescribed Streamflow to Improve Juvenile Steelhead Habitat. Theses and Dissertations Collection, University of Idaho Library Digital Collections. https://www.lib.uidaho.edu/digital/etd/items/etd_407.html
Contact us about this record

Watershed Management and Ecohydrology
Natural resources program: watershed management & ecohydrology emphasis.
Request info
A watershed management and ecohydrology emphasis within our graduate program prepares you to manage water resources by understanding how hydrologic processes are affected by management activities and land surface conditions in upland watersheds, especially in the arid Southwest.
About the Watershed Management & Ecohydrology Emphasis
This degree emphasis combines courses such as dryland ecohydrology and vegetation dynamics, wildland water quality, ecological climatology, fire ecology, and economic evaluation of water and environmental policy with an individual plan of study focused on your selected area of research.
Degree Requirements
You are encouraged to review the degree options and requirements before applying. In addition to research and seminar opportunities, you will have the ability to pursue advanced coursework in areas like:
- Dendrochronology
- Climate Change and Dryland Ecosystem Ecology
- Watershed Hydrology and Management
- Wildland Water Quality
- Ecological Climatology
- Conservation Planning & Wildland Recreation
- Biosphere-Atmosphere Interactions
- Ecosystem Ecology and a Sustainable Future
- Economic Evaluation of Water and Environmental Policy
Degree options & requirements
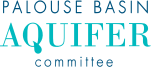
The Palouse Groundwater Basin is the sole source of drinking water across eastern Washington (Pullman, WA) and northern Idaho (Moscow, ID). The Palouse Basin Aquifer Committee (PBAC) is a consortium of public entities including cities, counties, and universities, along with the respective state water agencies. By working together, their goal is to implement the Palouse Basin Groundwater Management Plan, enacted in 1992, by analyzing and researching the Palouse Groundwater Basin. The Committee monitors groundwater levels and annual water usage, continually advocates for education opportunities within the community, and explores implementing supplemental water sources.
General Information
2015 Informational Update to the 1992 Groundwater Management Plan || Download
Original 1992 Groundwater Management Plan || Download
Boundary Map of the Palouse Basin Aquifer System || Download
Entity Action Plans || Download
PBAC Fact Sheet || Download
PBAC Mission and Goals || Download
PBAC Interagency Agreement || Download
History of Groundwater Management on the Palouse || Download
PBAC Bylaws || Download
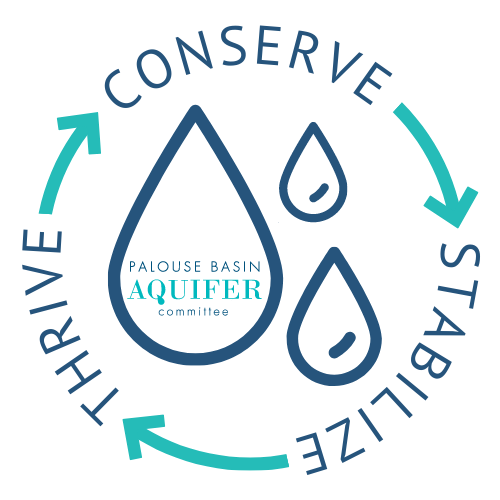
208-885-6429
[email protected], 875 perimeter dr ms 3310 moscow id, 83844.

IMAGES
VIDEO
COMMENTS
Water and Environmental Studies Department of Thematic Studies Linköping University Master's programme Science for Sustainable Development Master's Thesis, 30 ECTS credits ISRN: LIU-TEMAV/MPSSD-A--09/004--SE Linköpings Universitet Participatory watershed management to decrease land degradation and sediment transport in Kagera
Tonameca watershed is located in the state of Oaxaca, South Pacific coast of Mexico. Tonameca watershed covers 49 800 hectares with a total population of 28 000 habitants and around 52 habitants per km2 within six municipalities. Only Tonameca municipality has 15 546 habitants, where 96 % are zapotecs, and 5000 individuals speaking zapotec ...
Integrated watershed management is a method of continuous restoration, ... Bekele M (2003) Forest property rights, the role of the state, institutional exigency. Doctoral thesis in Uppsala, 409 (3): 227. Bekele S, Tilahun K (2007) Regulated deficit irrigation scheduling of onion in a semiarid region of Ethiopia. Agric Water Manag 89(1-2):148 ...
The positive roles of drought-tolerant crop variety and farmland soil and water management techniques were reported in southern and northern Ethiopia (Getie et al., 2020; Bedeke et al., 2018). Integrated watershed management is one of the crucial activities that are widely implemented in Ethiopia and successful lessons recorded.
PDF | On Jan 1, 2007, Honghai Qi published Integrated Watershed Management and Agricultural Land-use Optimization under Uncertainty | Find, read and cite all the research you need on ResearchGate
This metric is used to evaluate the performance of the storm water. management strategies in comparison to peak flow. As the HFR captures both the timing. and area being inundated, this metric would give a better understanding on the. downstream impacts caused due to the development and strategies used to in watershed.
A watershed plan is a strategy and a work plan for achieving water resource goals that provide assessment and management information for a geographically defined watershed. Remote sensing and GIS, which plays an important role in detecting and monitoring the physical characteristics of watershed area by reflected and emitted radiation, is also ...
Consult the top 38 dissertations / theses for your research on the topic 'Sustainable watershed management.'. Next to every source in the list of references, there is an 'Add to bibliography' button. Press on it, and we will generate automatically the bibliographic reference to the chosen work in the citation style you need: APA, MLA, Harvard ...
A decision support framework (DSF) to guide watershed management planning in St. Lucia was developed. The DSF, supported by three components, integrated physiographic criteria in spatially defining sustainable land management regimes, crop suitability based on agro-ecologic zoning criteria, and water quality modelling tools.
Create a spot-on reference in APA, MLA, Chicago, Harvard, and other styles. Consult the top 50 dissertations / theses for your research on the topic 'Watershed management.'. Next to every source in the list of references, there is an 'Add to bibliography' button. Press on it, and we will generate automatically the bibliographic reference to the ...
Enhance capacities to undertake risk-sensitive, sustainable watershed management planning in urban areas. Promote innovative approaches to overcome capacity limitations, such as fostering public-private sector partnerships. Support community and civil society involvement in watershed planning processes to.
The concept of watershed management has existed for millennia. The Atharva Veda text from 800 BC contains what may well be the first written reference to watershed management. Atharva Veda verse 19, 2.1 states that: "one should take proper managerial action to use and conserve water from mountains, wells, rivers and also rainwater for use in drinking, agriculture, industries" (Chandra 1990).
Runoff Estimation and Water Management for Holetta River, Awash subbasin, Ethiopia Thesis Submitted to Ethiopian Institute of Water Resources in Partial Fulfilment of the Requirements for the Degree of Master of Science In Water Resources Engineering and Management (Specialization: Surface Water Hydrology) By Mahtsente Tibebe Tadese
Jeba Watershed, Deder Woreda, Ethiopia A Thesis Submitted to the School of Natural Resources Management and Environmental Sciences, Postgraduate Program Directorate ... Impact watershed management on Environmental and Ecological Change 32 4.3.5. Impact on Social Interaction 33 4.4. Variability and Trend of Rainfall and Temperature 34
aggregate welfare gains from watershed management practices were calculated to be 931581.09 Birr and 753909.23 Birr per year from double bounded dichotomous choice and open-ended format, respectively. Therefore, the policymakers should make households to pay for the services of watershed management practices in the study area.
several water management practices, institutional settings, and regional policies on municipal and agricultural sectors. Generally, the agent-based adoption of water management strategies can alleviate the harm of water scarcity while providing positive feedbacks to reducing municipal costs and increasing agricultural profit from production.
The maximum watershed length is close to twice the diameter of a circle having the same area as the watershed for circular-shaped catchments (Schumm, Citation 1956). L b reveals the watershed's main channel, which carries most water, and is commonly used to compute the time element of water flow over a watershed.
Watershed management is supposed to be practiced as a means to increase rain-fed and irrigation agricultural production, conserve natural resources and reduce poverty in Ethiopia, a country characterized by low agricultural productivity, severe natural resource degradation, and high levels of poverty (Kerr 2002). Under the current conditions in ...
Water resource management is a grave challenge today particularly in areas deficient in water resources. Optimum utilization of surface and groundwater, their storage and conservation are the core issues concerning water resource management. ... An M. Tech Dissertation submitted to the Department of Civil Engineering, National Institute of ...
The study suggests that the hydrologic regime in the MCEW will shift from snow to rain dominated by the middle of the 21 st century. Canopy removal emphasizes more on annual water yield changes, whereas climate change influences more on streamflow timing. Description: Thesis (Ph. D., Environmental Science)--University of Idaho, October 1, 2010.
Abstract. Large-scale urban growth has modified the hydrological cycle of our cities, causing greater and faster runoff. Urban forests (UF), i.e. the stock of trees and shrubs, can substantially ...
Thesis (M.S., Environmental Science)--University of Idaho, December 2013. ... The objectives of this study were to monitor water quality conditions as a response of summer baseflow augmentation upstream and downstream of an effluent discharge point, quantify instream losses and create a reservoir management plan to improve salmonid summer ...
A watershed management and ecohydrology emphasis within our graduate program prepares you to manage water resources by understanding how hydrologic processes are affected by management activities and land surface conditions in upland watersheds, especially in the arid Southwest. Apply now. About the Watershed Management & Ecohydrology Emphasis
The Palouse Basin Aquifer Committee (PBAC) is a consortium of public entities including cities, counties, and universities, along with the respective state water agencies. By working together, their goal is to implement the Palouse Basin Groundwater Management Plan, enacted in 1992, by analyzing and researching the Palouse Groundwater Basin.