Featured Topics
Featured series.
A series of random questions answered by Harvard experts.

Explore the Gazette
Read the latest.
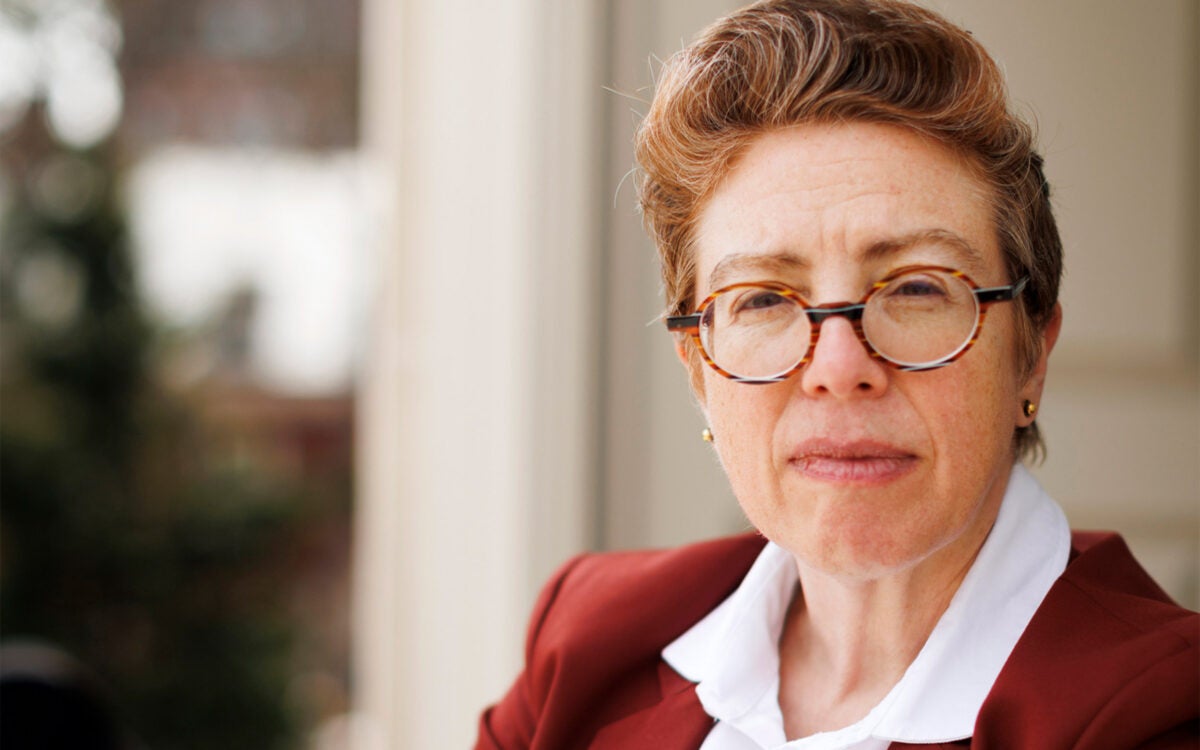
Footnote leads to exploration of start of for-profit prisons in N.Y.
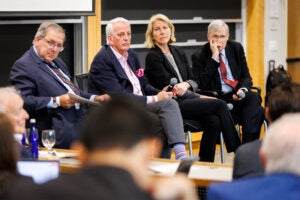
Should NATO step up role in Russia-Ukraine war?
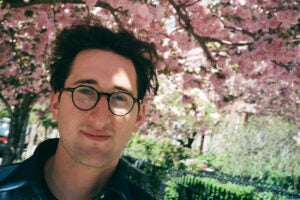
It’s on Facebook, and it’s complicated
Employment in STEM occupations has grown 79 percent in the past three decades, according to U.S. Bureau of Labor Statistics.
iStock by Getty Images
Growing gap in STEM supply and demand
Brigid O’Rourke
Harvard Correspondent
Experts cite online learning, digital tools as ways to build inclusive and equitable STEM workforce
The evolution and impact of STEM education and its accompanying career opportunities reflect a positive in the fields of science, technology, engineering, and mathematics. But as the need grows for a specialized STEM-focused workforce, it’s becoming clear that not everyone has an equal opportunity.
During the Harvard-sponsored talk, “New Pathways to STEM,” panelists cited a large subset of students who are not being fully prepared for STEM careers. They then discussed ways the gap could be closed, pointing to online learning and the rapid advancement of new digital tools as ways to make STEM education more readily available. These new ways of learning, they said, can ultimately expand access to STEM education and create a more inclusive and equitable STEM workforce.
The need for a vast, talented workforce in STEM-related fields has never been more necessary, said Bridget Long, dean of the Harvard Graduate School of Education. Long cited the U.S. Bureau of Labor Statistics, which shows employment in STEM occupations has grown 79 percent in the past three decades. In addition, STEM jobs are projected to grow an additional 11 percent from 2020 to 2030. In Massachusetts alone, “40 percent of all employment revolves around innovation industries, such as clean energy, information technology, defense and advanced manufacturing,” said Long.
But, she added, “the importance of STEM education is about so much more than just jobs. STEM fields demand curious individuals eager to solve the world’s most pressing problems.”
“We need to have a new vision of how we prepare students to think critically about the world … as well as educating a society such that it has scientific literacy,” said Joseph L. Graves Jr., (upper left). Joining Graves were Brigid Long, Mike Edmonson, Amanda Dillingham, and Martin West.
The study of STEM subjects, she continued, teaches critical-thinking skills, and instills a mindset that will help students find success across numerous areas and disciplines. However, Long said, “too often the opportunity to learn and to be inspired by STEM is not available.
“Only 20 percent of high school graduates are prepared for college-level coursework in STEM majors,” she cited, adding, “fewer than half of high schools in the United States even offer computer science classes. So that begs the question — are kids going to be ready to meet the evolving and growing landscape of STEM professions?”
While STEM education opportunities are often scarce for high school students across the board, it’s even more pervasive when you consider how inequitably access is distributed by income, race, ethnicity, or gender. For example, Long said, “Native American, Black and Latinx students are the least likely to attend schools that teach computer science, as are students from rural areas, and [those with] economically disadvantaged backgrounds.
“It’s not surprising that these differences in educational opportunities lead to very large differences in what we see in the labor force. We are shutting students out of opportunity,” she said.
So what can be done to ensure more students from all backgrounds are exposed to a wide variety of opportunities? According to Graduate School of Education Academic Dean Martin West, who is also a member of the Massachusetts Board of Elementary and Secondary Education, a concerted effort is being made at the state level to work with — and through — teachers to convey to students the breadth of STEM opportunities and to assure them that “it’s not all sitting in front of a computer, or being in a science lab, but showing them that there are STEM opportunities in a wide range of fields.”
The relatively recent emergence of digital platforms, such as LabXchange, are helping to bridge the gap. LabXchange is a free online learning tool for science education that allows students, educators, scientists, and researchers to collaborate in a virtual community. The initiative was developed by Harvard University’s Faculty of Arts and Sciences and the Amgen Foundation . It offers a library of diverse content, includes a biotechnology learning resource available in 13 languages, and applies science to real-world issues. Teachers and students from across the country and around the world can access the free content and learn from wherever they are.
Many of the panelists also pointed to the need for steady funding in helping to address the inequities.
“Bottom line, if this nation wants to be a competitive leader in STEM, it has to revitalize its vision of what it needs to do, particularly in the public schools where most Black and brown people are, with regard to producing the human and physical infrastructure to teach STEM,” said Joseph L. Graves Jr., professor of biological sciences, North Carolina Agricultural and Technical State University. Graves is also a member of the Faculty Steering Committee, LabXchange’s Racial Diversity, Equity, and Inclusion in Science Education Initiative.
The panel noted how LabXchange is partnering with scholars from several historically Black colleges and universities to develop new digital learning resources on antiracism in education, science, and public health. The content, which will be freely available and translated into Spanish, is being funded by a $1.2 million grant from the Amgen Foundation. Aside from the highly successful LabXchange program, Mike Edmondson, vice president, Global Field Excellence and Commercial, Diversity Inclusion & Belonging at Amgen, noted the Amgen Biotech Experience and the Amgen Scholars program — both of which help to ensure that everyone has the opportunity to engage in science and to see themselves in a STEM career.
We also have to do a better job at helping people understand that that we cannot afford to fall behind in STEM education, Graves argued. “That means it’s going to cost us some money. So, America needs to be willing to pay … to build out STEM education infrastructure, so that we can produce the number of STEM professionals we need going forward,” he said. “We need to have a new vision of how we prepare students to think critically about the world … as well as educating a society such that it has scientific literacy.”
Amanda Dillingham, the program director of science and biology at East Boston High School, is on the front lines of this challenge, and says she believes that supporting teachers is one of the most critical steps that can be taken to address the issue in the immediate future.
When more funding is brought to the table, teachers “are able to coordinate networks … and build biotech labs in our classrooms and build robotics labs in our classrooms …. and are actually able to introduce students to [these fields and these careers] at a very early age,” said Dillingham.
Long and the panel also paid tribute to Rob Lue, the brainchild behind LabXchange, who passed away a year ago.
“Rob challenged science learners, scientists and educators to commit to ending racial inequity,” Long said. “Access was at the core of all of Rob’s many contributions to education at Harvard and beyond. He envisioned a world without barriers and where opportunity was available to anyone, especially in science. In everything that he did, he created an environment in which learners of all ages of diverse backgrounds could come together to imagine, learn, and achieve live exchange. Rob’s free online learning platform for science was his most expansive vision, and one that continues to inspire educators and learners around the world.”
Share this article
You might like.
Historian traces 19th-century murder case that brought together historical figures, helped shape American thinking on race, violence, incarceration
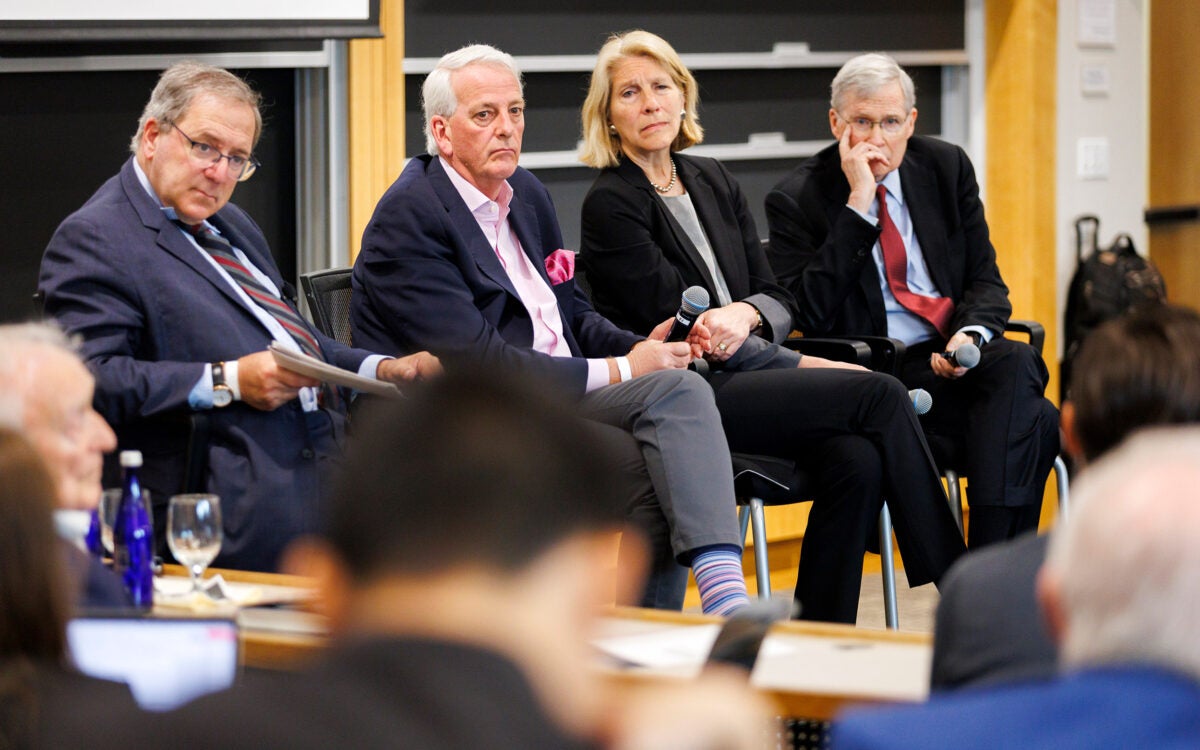
National security analysts outline stakes ahead of July summit

‘Spermworld’ documentary examines motivations of prospective parents, volunteer donors who connect through private group page
Six receive honorary degrees
Harvard recognizes educator, conductor, theoretical physicist, advocate for elderly, writer, and Nobel laureate
Everything counts!
New study finds step-count and time are equally valid in reducing health risks
Bridging social distance, embracing uncertainty, fighting for equity
Student Commencement speeches to tap into themes faced by Class of 2024
- Explore Your Interests – First-Years/Sophomores
- Explore Your Interests – Juniors/Seniors
- Charles Hamilton Houston Internship Program
- Meiklejohn Fellows Program
- Arts & Communication
- Business & Finance
- Education Professions
- Health Professions
- Science & Technology
- Social Impact
- Law School Advising
- Medical School Advising
- Alumni-in-Residence
- Job and Internship Fair – Diverse, Equitable and Inclusive Workplaces
- Sophomore Summit
- Arts and Humanities in Action
- African-American / Black / Caribbean Ancestry
- Asian-American / South Pacific
- DACA and Undocumented Students
- First Generation and/or Low-Income
- Indigenous Peoples of the Americas / First Nation
- International
- Latinx American
- Students With Disabilities
- Women/Trans/Non-Binary
- Create a Resume/Cover Letter/LinkedIn Profile
- Expand Your Network/Mentor
- Job Search Strategy Toolkit
- Search for a Job/Internship
- Prepare for an Interview
- Prepare for Graduate School
- Negotiate an Offer
- Job Market Trends
- Student Staff
- Student Outcomes
- Timeline: Career Development at Amherst College
- Michael Loeb ’77 P’22
- Student Policies and Expectations
- Advisory Council
10 Tips to Enhance Your Critical Thinking Skills in STEM Careers
- Share This: Share 10 Tips to Enhance Your Critical Thinking Skills in STEM Careers on Facebook Share 10 Tips to Enhance Your Critical Thinking Skills in STEM Careers on LinkedIn Share 10 Tips to Enhance Your Critical Thinking Skills in STEM Careers on X
This article emphasizes the pivotal role of professionals in STEM careers as drivers of innovation and highlights critical thinking as an indispensable skill in navigating complex challenges. It offers ten actionable tips for refining critical thinking capabilities in STEM.

CTE Pathways to STEM Occupations
Download the full brief
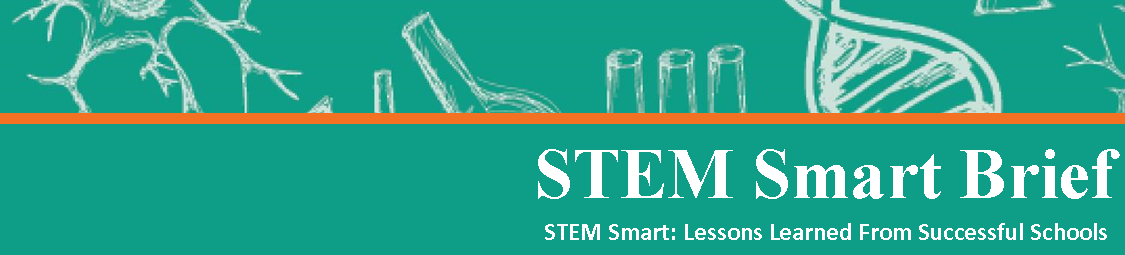
THE PROBLEM
U.S. employers are scrambling to fill jobs that are key to competing in the global economy. Many of these jobs—in computing, energy, manufacturing, and other fields—demand sophisticated STEM skills and knowledge, pay well, and require some higher education, but do not require a bachelor’s degree. Some call these “middle-skill” jobs. Yet in The Hidden STEM Economy , Jonathan Rothwell suggests a more robust term, “high-STEM,” for all occupations that require a “high level of knowledge” in any one STEM field.
Whether you call these jobs middle-skill or high-STEM, the reality is the same. As the number of new jobs that demand advanced STEM skills grows, there is a shortage of skilled workers. For the U.S. to remain competitive, business as usual will not work. The educational community and employers need to join forces to prepare an ample high-STEM workforce. Two undervalued key players—secondary career and technical education (CTE) and community colleges—will be instrumental in this effort.
“ Workers in STEM fields play a direct role in driving economic growth. Yet, because of how the STEM economy has been defined, policymakers have mainly focused on supporting workers with at least a bachelor’s degree, overlooking a strong potential workforce of those with less than a BA .” The Hidden STEM Economy
Striking Statistics: The High-STEM Workforce—Status, Growth, and Gaps
- Over 50% of STEM jobs do not require a bachelor’s degree, and almost 50% of students who receive four-year STEM degrees begin their learning at community colleges. 1
- At all levels of educational attainment, people employed in STEM jobs earn 11% higher wages compared with their same-degree counterparts in other jobs. 2
- U.S. Department of Labor projections show that 15 of the 20 fastest growing occupations require significant science or mathematics training to compete successfully for a job. 3
- Educational institutions “are not on track to keep pace with” the growing need for Computer System Analysts, Web Developers, and several key Big Data occupations—all of which require extensive skills and knowledge, but not necessarily a BA. 4
- In a National Association of Manufacturers survey, 67% of respondents reported that they do not have enough skilled employees—technicians, machinists, craft workers—and 56% reported this gap would grow through 2016. 5
- Only 22% of the $4.3 billion in government funding spent on STEM education goes to “sub-bachelor’s degree” education or training. 6
KEY ISSUES AND PROMISING PROGRAMS
This brief, the ninth in a series, explores issues and promising practices in paving CTE pathways to high-STEM occupations—a key piece of the STEM college and career readiness puzzle. Other briefs focus on related parts of the puzzle, such as the role that math and science standards play in students’ college and career readiness, providing rich STEM learning in preschool and out-of-school time programs, and strategies to ensure all students enjoy a successful STEM education. 7
Secondary Career and Technical Education Secondary CTE can make STEM learning more meaningful and engaging through applied, student-centered approaches. These applied learning approaches help youth acquire key employability skills such as critical thinking, problem solving, collaboration, communication, and creativity. 8 When secondary CTE infuses applied STEM learning into rigorous programs of study, aligns with post-secondary programs, awards credentials, and offers dual enrollment programs that provide college credits, it propels youth toward college and career goals. Despite these strengths, CTE has received scant respect in the K–12 arena. In 2014, this is changing. Policymakers’ interest and student enrollment in secondary CTE are both on the rise. Yet recent reports highlight potential obstacles to programs’ ability to expand and enhance secondary CTE:
- The primary federal source of CTE funding, Perkins, is failing to keep pace with the demand to expand CTE and create new CTE programs of study relevant to STEM occupations. The recent FY 14 Omnibus Bill restored $53 million of the FY 13 sequestration cuts, but did nothing to restore FY 11 cuts ($140 million). With level or declining funding, this reflects a pattern of “erosion” in Perkins funds. 9
- Findings from a survey of 850 secondary CTE educators offer a glimpse into current needs. Since 2008, program enrollment increased for 60% of respondents, while the budgets of 73% remained flat or decreased. Respondents’ top priority for funds is not expanding programs, but attending to areas hit with deep budget cuts: equipment, professional development, technology, and curriculum. Nearly 60% reported they integrate academic learning into CTE, yet 70% said this integration is one-way; academic courses do not connect to CTE. 10
- As noted on page 3, numerous reports point to the urgent need to build bridges between secondary and post-secondary CTE—through rigorous programs of study that integrate secondary and postsecondary curricula and shared standards (e.g., Common Career Technical Core), for example—to ensure a strong coherent continuum of STEM learning. Without such bridges, as the authors of one report note, “…many students earn college credit in high school that has limited value in the postsecondary space beyond a single institution of higher education.” 11 Reports also underscore the need to improve professional development 12 and assessment approaches 13 to ensure youth are ready for post-secondary STEM learning.
Although these obstacles are formidable, a recent policy analysis conducted by the National Association of State Directors of Career Technical Education Consortium (NASDCTEc) and the Association for Career and Technical Education (ACTE) indicates that all but three states took legislative action to improve secondary CTE in 2013, and over 30 states invested new resources in secondary CTE. Eleven of the 30 states “addressed STEM in some way.” 14
In addition to state support, partnerships between STEM employers and community colleges offer a powerful mechanism to strengthen secondary CTE. Connections with STEM employers help secondary CTE teachers and programs keep abreast of changing workforce skill needs, enable students to explore careers, provide students with mentors and role models—as well as internships and externships—and deepen understanding of high-STEM occupations. Collaboration with community colleges helps create an aligned progression of learning that ensures students stay on track to meet their goals and fosters a skilled high-STEM workforce.
Community Colleges In recent years, college and career readiness has, for many K–12 schools, families, students, and policymakers, come to mean a straight progression, first to college then career. Yet, an alternate trajectory—some post-secondary learning, employment and on-the-job-learning, then more post-secondary learning—benefits many students. This path can also help meet the urgent and growing demand for a high-STEM workforce. Community colleges are in the vanguard of guiding students through this alternate trajectory. These institutions help prepare an exceedingly diverse population of students for a wide array of careers, including high-STEM occupations, yet face complex challenges.
A 2011 NSF-supported National Academies Summit identified five challenges: 15
- Overcoming students’ inadequate academic preparation for STEM study
- Recruiting and retaining students in STEM education
- Creating and sustaining effective partnerships between two- and four-year institutions
- Finding the resources to support and sustain STEM education program improvement
- Aligning STEM education with workforce demands and practice
Other recent reports capture additional dimensions of community colleges’ challenges, many of which involve alignment and strongly affect secondary CTE programs:
- NASDCTEc has identified a speed bump in creating a smooth learning progression and ensuring credit transfer for students moving from secondary to post-secondary CTE and from one community college to another. Only 13 states have state-approved postsecondary CTE standards (in contrast to 46 states with approved secondary CTE standards). Only two states have standards that are fully aligned between secondary and postsecondary CTE systems. 16
- The National Governors Association (NGA) has issued an urgent call for governors to link STEM employers with colleges, pilot “new models of STEM education” in community colleges, reward colleges and students for “STEM course-completion,” and support students’ mastery of key mathematics skills—an obstacle to course completion. The NGA also suggests mandating that all community college credits and credentials be “stackable” (offer multiple entry and exit points while counting toward degrees), “portable” (count for credit at other institutions), and align with industry-validated certificates. 17
- The Center for Law and Social Policy (CLASP) and Georgetown Public Policy Institute argue for stronger, more coherent systems—including data systems—to ensure all learners receive credit for rigorous post-secondary coursework, credentials, and training. Georgetown calls for the government to help CTE craft programs of study that are aligned across secondary and post-secondary levels to avoid duplication of coursework and ensure credit. 18 CLASP recommends the U.S. institute a national, “competency-based system for measuring learning and awarding postsecondary credit.” 19
Nationwide, initiatives funded by the NSF’s ATE program and the Department of Labor’s Trade Adjustment Assistance Community College and Career Training (TAACCCT) program are addressing some of these challenges. The ATE program is working to “prepare technicians for the high-technology workplaces that the United States needs to prosper.” Thirty-nine ATE centers and many ATE-funded projects are using innovative approaches to tackle workforce preparation issues through strategic partnerships among K–12 education, secondary CTE, two- and four-year colleges, and employers. 20 TAACCCT grantees are using problem- and project-based approaches to create rigorous programs focused on high-STEM careers, engaging employers in program design, creating career-coaching systems, and helping students attain key mathematics and literacy skills (e.g., Massachusetts Community College and Workforce Development Transformation Agenda). 21
STEM Employers As the Department of Commerce notes in its report, Good Jobs Now and For the Future , “U.S. businesses frequently voice concerns over the supply and availability of STEM workers.” 22 At the same time, reports highlight gaps between workplace needs and young adults’ capabilities across U.S. industries. 23 For decades, the “career” part of college and career readiness has taken a backseat, and young adults and employers alike are suffering from its omission:
- Findings from a 2006 survey of 400 employers indicate that “professionalism/work ethic, oral and written communications, teamwork/collaboration, and critical thinking/problem solving” are essential to workplace success. The same survey found employers believe that many high school graduates lack these skills—and that graduates from two- and four-year college, while better prepared, are also deficient in essential skills. 24
- Most recently, in a 2013 survey of 318 employers, 93% of respondents said that an applicant’s ability to “think critically, communicate clearly, and solve complex problems is more important than their undergraduate major.” Over 75% wanted colleges to place more emphasis on critical thinking, problem solving, communication, and “applied knowledge in real-world settings.” And, 40% said colleges are only doing a “fair” job of preparing students for the workplace. 25
Nationwide, there is a call for STEM employers to team up with K–20 educators to create clear, well-defined pathways to higher learning and workplace success. 26 By collaborating with post-secondary CTE and community colleges, in particular, STEM employers can help shape programs to ensure youth are ready for high-STEM occupations. Some employers feel they do not have a role to play. When asked “Who has primary responsibility for workforce readiness?,” one survey of employers found that 76% felt that K–12 schools were responsible; only 19% felt employers were responsible, and 11% felt the business community was responsible. 27 In contrast, other employers are diving in to help by joining efforts such as Change the Equation, the National Network of Business and Industry Associations, and 100Kin10. And, nationwide there are examples of promising initiatives in which employers are engaging in partnerships with secondary CTE and community colleges and providing innovative mentoring, on-the-job learning opportunities, and student and teacher externship programs.
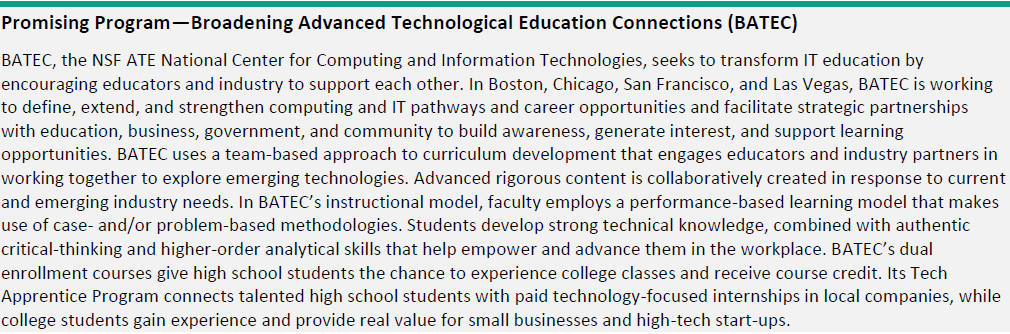
CONCLUSIONS
To foster a skilled high-STEM workforce, business, government, and education leaders must jettison old paradigms of successful STEM learning that focus on four-year colleges as the sole path to career readiness. Other routes—attaining some post-secondary training, entering the workforce, and continuing to pursue learning, credits, degrees, and/or credentials while on the job—are equally valid and important. It will require decisive action to realize the full potential of secondary CTE and community colleges in preparing students for college and careers:
- Create a strong, rigorous, seamless STEM learning continuum that is aligned across K–12 education, secondary CTE, community colleges, four-year institutions, and industry.
- Adopt, align, and implement common standards across secondary and post-secondary CTE to ensure that all learning is aligned, stackable, and portable.
- Draw on the active, applied learning strengths of CTE to spark students’ interest in STEM, enable high school students to explore STEM careers, and increase the workplace relevance of four-year STEM learning.
- Ensure that all secondary CTE programs and community colleges have resources—including specialized equipment, educator professional development, and effective assessments—to provide the future high-STEM workforce with outstanding STEM learning.
- Explore options to retool key aspects of dual enrollment—including tuition and financial aid, high school-college partnerships, and articulation agreements—to make this beneficial program accessible to more students.
- Continue to look to STEM employers as a partner in ensuring programs reflect employers’ evolving needs, strengthening programs, and scaling up effective new models.

This project is funded by the National Science Foundation, grants # 0822241, 1449550, 1650648, 1743807, and 1813076 . Any opinions, findings, and conclusions or recommendations expressed in these materials are those of the author(s) and do not necessarily reflect the views of the National Science Foundation.

An official website of the United States government
The .gov means it’s official. Federal government websites often end in .gov or .mil. Before sharing sensitive information, make sure you’re on a federal government site.
The site is secure. The https:// ensures that you are connecting to the official website and that any information you provide is encrypted and transmitted securely.
- Publications
- Account settings
Preview improvements coming to the PMC website in October 2024. Learn More or Try it out now .
- Advanced Search
- Journal List
- Springer Nature - PMC COVID-19 Collection

Ways of thinking in STEM-based problem solving
Lyn d. english.
Queensland University of Technology, Brisbane, Australia
Associated Data
The data sets generated during and/or analysed during the current study are available from the corresponding author on reasonable request.
This article proposes an interconnected framework, Ways of thinking in STEM-based Problem Solving , which addresses cognitive processes that facilitate learning, problem solving, and interdisciplinary concept development. The framework comprises critical thinking, incorporating critical mathematical modelling and philosophical inquiry, systems thinking, and design-based thinking, which collectively contribute to adaptive and innovative thinking. It is argued that the pinnacle of this framework is learning innovation, involving the generation of powerful disciplinary knowledge and thinking processes that can be applied to subsequent problem challenges. Consideration is first given to STEM-based problem solving with a focus on mathematics. Mathematical and STEM-based problems are viewed here as goal-directed, multifaceted experiences that (1) demand core, facilitative ways of thinking, (2) require the development of productive and adaptive ways to navigate complexity, (3) enable multiple approaches and practices, (4) recruit interdisciplinary solution processes, and (5) facilitate the growth of learning innovation. The nature, role, and contributions of each way of thinking in STEM-based problem solving and learning are then explored, with their interactions highlighted. Examples from classroom-based research are presented, together with teaching implications.
Introduction
With the prevailing emphasis on integrated STEM education, the power of mathematical problem solving has been downplayed. Over two decades we have witnessed a decline in research on mathematical problem solving and thinking, with more questions than answers emerging (English & Gainsburg, 2016 ; Lester & Cai, 2016 ). This is of major concern, especially since work and non-work life increasingly call for resources beyond “textbook” problem solving (Chin et al., 2019 ; Krause et al., 2021 ). Such changing demands could not have been more starkly exposed than in the recent COVID 19 crisis, where mathematics played a crucial role in public and personal discourse, in describing and modelling current and potential scenarios, and in explaining and justifying societal regulations and restrictions. As Krause et al. ( 2021 ) highlighted, “No mathematical task we can create could be a richer application of mathematics than this real situation” (p. 88).
Modelling and statistical analyses that led the search for strategies to “flatten the curve” with minimal social or economic detriment were prominent in the media (Rhodes & Lancaster, 2020 ; Rhodes et al., 2020 ). As nations strive to rebuild their economies including grappling with crippling energy costs, advanced modelling again plays a key role (Aviso et al., 2022 ; Oxford Economics, 2022 ; Teng et al., 2022 ). Unfortunately, the gap between the mathematical modelling applied during the pandemic and how the public interpreted the models has been “palpable and evident” (Aguilar & Castaneda, 2021 ). Sadly, as Di Martino (Krause et al., 2021 ) pointed out regarding his nation:
People’s reactions in this pandemic underlined the spread of a widely negative attitude toward mathematics among the adult population in Italy. We have witnessed the proliferation of strange, unscientific, and dangerous theories but also the risk of refusing to approach facts that involve math and the resulting dependence to fully rely on others when mathematics is used to justify decisions. Also, on this occasion many people showed their own fear of math and their rejection of mathematical arguments as relevant factors in justification (p. 93).
This heightened visibility of mathematics in society coupled with a general lack of mathematical literacy sparked major questions about the repercussions for education and research (Bakker & Wagner, 2020 ; Kollosche & Meyerhöfer, 2021 ). One such repercussion is the need to reconsider perspectives on STEM-based problem solving and how different ways of thinking can enhance or hinder solutions. With reference to engineering education, Dalal et al. ( 2021 ) indicated how numerous calls for a focus on ways of thinking have largely been taken for granted or at least treated at a superficial level. As these authors pointed out, while perceived as a theoretical concept, ways of thinking “can and should be used in practice as a structure for solution-oriented outlooks and innovation” (p. 109).
Given the above points, I propose an interconnected framework, Ways of thinking in STEM-based Problem Solving (Fig. 1 ), which addresses cognitive processes that facilitate learning, problem solving, decision-making, and interdisciplinary concept development (cf. Slavit et al., 2021 ). The framework comprises critical thinking (including critical mathematical modelling and philosophical inquiry), systems thinking, and design-based thinking. Collectively, these thinking skills contribute to adaptive and innovative thinking (McKenna, 2014 ) and ultimately lead to the development of learning innovation (Sect. 3.4 ; English, 2018 ).

These thinking skills have been chosen because of their potential to enhance STEM-based problem solving and interdisciplinary concept development (English et al., 2020 ; Park et al., 2018 ; Slavit et al., 2021 ). Highlighting these ways of thinking, however, is not denying the importance of other thinking skills such as creativity, which is incorporated within the adaptive and innovative thinking component of the proposed framework (Fig. 1 ), and is considered multidimensional in nature (OECD, 2022 ). Other key skills such as communication and collaboration (Stehle & Peters-Burton, 2019 ) are acknowledged but not explored here.
In line with Dalal et al. ( 2021 ), I consider the proposed ways of thinking as providing an organisational structure both individually and interactively when enacted in practice—notwithstanding the contextual and instructional influences that can have an impact here (Slavit et al., 2022 ). Prior to exploring these ideas, I consider STEM-based problem solving with a focus on mathematics.
STEM-based problem solving: a focus on mathematics
Within our STEM-intensive society, we face significant challenges in promoting STEM education from the earliest grades while also maintaining the integrity of the individual disciplines (Tytler, 2016 ). With the increasing need for STEM skills across multiple workforce domains, contrasted with difficulties in STEM implementation in many schools (e.g., Dong et al., 2020 ), the urgency to advance STEM education has never been greater. With the massive disruption caused by COVID-19, coupled with problematic international relations, our school students’ futures have become even more uncertain—we cannot ignore the rapid changes that will continue to impact their lives. Unlike business and industry, where disruption creates a “force-to-innovate” approach (Crittenden, 2017 , p. 14), much of school education seems oblivious to preparing students for these disruptive forces or at least are restricted in doing so by set curricula.
Preparing our students for an increasingly uncertain and complex future requires rethinking the nature of their learning experiences, in particular, the need for more relevant and innovative problems that are challenging but manageable, and importantly, facilitate adaptive learning and problem solving (McKenna, 2014 ). A failure to provide such opportunities may have detrimental effects on young students’ learning and their future achievements (Engel et al., 2016 ). Despite mathematics being cited as the core of STEM education and foundational to the other disciplines (e.g., Larson, 2017 ; Roberts et al., 2022 ; Shaughnessy, 2013 ), it is frequently ignored in integrated STEM activities (English, 2016 ; Maass et al., 2019 ; Mayes, 2019 ; Shaughnessy, 2013 ). For example, quantitative reasoning, which is critical to integrated STEM problem solving, is frequently “misrepresented, underdeveloped, and ignored in STEM classrooms” (Mayes, 2019, p. 113). Likewise, Tytler ( 2016 ) warned that there needs to be an explicit focus on the mathematical concepts and thinking processes that arise in STEM activities. Without this focus, STEM programs run the risk of reducing the valuable contributions of mathematical thinking. If children fail to see meaningful links between their learning in mathematics and the other STEM domains, they can lose interest not only in mathematics but also in the other disciplines (Kelley & Knowles, 2016 ).
Traditional notions of mathematical problem solving (e.g., Charles, 1985 ) are now quite inadequate when applied to our current world. At a time of increasing creative disruption, it is essential for mathematical problem solvers to be adaptive in dealing with unforeseen local, national, and international problems. Increasingly, STEM-based problems in the real world encompass more than just disciplinary content and practices. While not denying the essential nature of these components, issues pertaining to cultural, social, political, and ethical dimensions (Kollosche & Meyerhöfer, 2021 ; Pheasants, 2020) can also impact the solution process, necessitating the application of appropriate thinking skills. As Pheasants stressed, “If STEM education is to prepare students to grapple with complex problems in the real world, then more attention ought to be given to approaches that are inclusive of the non-STEM dimensions that exist in those problems.”
In light of the above arguments, I view mathematical and STEM-based problems as goal-directed experiences that (1) demand STEM-relevant ways of thinking, (2) require the development of productive and adaptive ways to navigate complexity, (3) enable multiple approaches and practices (Roberts et al., 2022 ), (4) recruit interdisciplinary solution processes, and (5) facilitate growth of learning innovation for all students regardless of their background (English, 2018 ). In contrast to traditional expectations, such problems need to embody affordances that facilitate learning innovation, where all students can move beyond their existing competence in standard problem solving and be challenged to generate new knowledge in solving unanticipated problems. Even students who achieve average results on standardised tests display conceptual understanding and advanced mathematical thinking not normally seen in the classroom—especially when current common practices emphasise number skills at the expense of problem solving and reasoning with numbers (Kazemi, 2020 ).
Limited attention, however, has been paid to how problem experiences can be developed that press beyond basic content knowledge (Anderson, 2014; Li, Schoenfeld et al., 2019), encompass the STEM disciplines, and develop important ways of thinking. In their recent article, Slavit et al. ( 2021 ) argued that STEM education should be “grounded in our knowledge of how students think in STEM-focused learning environments” (p. 1), and that fostering twenty-first-century skills is essential. Yet, as these authors highlighted, there is not much research on STEM ways of thinking, with even fewer theoretical perspectives and frameworks on which to draw. In the next section, I consider these ways of thinking, defined in Table Table1, 1 , and provide examples of their applications to STEM-based problem solving.
Critical thinking
Although long recognised as a significant process in a range of fields, research on critical thinking in education, especially in primary education, has been limited (Aktoprak & Hursen, 2022 ). Critical thinking has long been associated with mathematical reasoning and problem solving, but their association remains under-theorized (Jablonka, 2020 ). Likewise, connections between critical thinking and design thinking have had limited attention largely due to their shared conceptual structures not being articulated (Ericson, 2022 ). As a twenty-first century skill, critical thinking is increasingly recognised as essential in STEM and mathematics education (Kollosche & Meyerhöfer, 2021 ) but is sadly lacking in many school curricula (Braund, 2021 ). As applied to STEM-based problem solving, critical thinking builds on inquiry skills (Nichols et al., 2019 ) and entails evaluating and judging problem situations including statements, claims, and propositions made, analysing arguments, inferring, and reflecting on solution approaches and conclusions drawn. Although critical thinking can contribute significantly to each of the other ways of thinking, its application is often neglected. For example, critical thinking is increasingly needed in design and design thinking, which play a key role in product development, environmental projects, and even in forms of social interaction (as discussed in Sect. 3.3 ; Ericson, 2022 ).
Critical mathematical modelling
One rich source of problem experiences that foster critical thinking is that of modelling. The diverse field of mathematical modelling has long been prominent in the secondary years (e.g., Ärlebäck & Doerr, 2018 ) but remains under-researched in the primary years, especially in relation to its everyday applications. Effective engagement with social, political, and environmental issues through modelling and statistics demands critical thinking, yet such aspects are not often considered in school curricula (Jablonka, 2020 ). This is of particular concern, given the pressing need to tackle such issues in today’s world.
As noted in several publications, the interdisciplinary nature of mathematical modelling makes it ideal for STEM-based problem solving (English, 2016 ; Maass et al., 2019 ; Zawojewski et al., 2008 ). Numerous definitions of models and modelling exist in the literature (e.g., Blum & Leiss, 2007 ; Brady et al., 2015 ). For this article, modelling involves developing conceptual innovations in response to real-world needs; effective modelling requires moving beyond the conventional ways of thinking applied in typical school problems (Lesh et al., 2013 ) to include contextual and critical analysis.
Much has been written about the role of modelling during COVID-19. Mathematical models played a major role in grappling with COVID 19, but their projections were a source of controversy (Rhodes & Lancaster, 2020 ). There seems little appreciation of the critical nature of mathematical models in society (Barbosa, 2006 ) and how assumptions in the modelling process can sway decisions. STEM-based problem solving needs to incorporate not just modelling itself, but also critical mathematical modelling. Critiquing what a model yields, and what is learned, is of increasing social importance (Aguilar & Castaneda, 2021 ; Barbosa, 2006 ). Indeed, as Braund ( 2021 ) illustrated, the Covid-19 pandemic has revealed the urgent need for “critical STEM literacy” (p. 339)—an awareness of the complex and problematic interactions of STEM and politics, and a knowledge and understanding of the underlying STEM concepts and representations is essential:
There are two imperatives that emerge: first, that there is sufficient STEM literacy to negotiate the complex COVID-19 information landscape to enable personal decision taking and second, that this is accompanied by a degree of criticality so that politicians and experts are called to account (Braund, 2021 , p. 339).
Kollosche ( 2021 ) shed further light on the lack of critical thinking in the media’s reporting on COVID, with his argument that “most newspaper reports were effective in creating the problems” because of their focus on ideal forms of mathematical concepts and modelling, without discussing the assumptions and methods behind the reported data. Of concern is that “mass media still fail to present scientific models and results in a way that allows for mathematical reflection and a critical evaluation of such information by citizens.”
Modelling experiences that draw on students’ cultural and community contexts (Anhalt et al., 2018 ; English, 2021a , 2021b ; Turner et al., 2009 ) provide rich opportunities for critical thinking from a citizen’s perspective. Such opportunities can also assist students in appreciating that mathematics is not merely a means of calculating answers but is also a vehicle for social justice, where critical thinking plays a key role (Cirillo et al., 2016 ; Greer et al., 2007 ). In their studies of critical thinking in cultural and community contexts, Turner and her colleagues (Turner et al., 2009 , 2021 , 2022 ) explored culturally responsive, community-based approaches to mathematical modelling with elementary teachers and students. Using a range of authentic modelling contexts, Turner and her colleagues illustrated how students’ modelling processes generated a number of issues that required them to think critically about their lives and their lives within their community. In one activity, students were applying design thinking and processes as they redesigned their local park. They generated, for example, mathematical models to estimate how long children would have to wait to use the swings—this informed their decision that the park did not meet the needs of the community. This community-based modelling highlighted “ongoing negotiation between students’ experiences and intentions related to the community park, the constraints of the actual context, and the mathematical issues that arose” (Turner et al., 2009 , p. 148).
Another example of modelling involving critical thinking in cultural and community contexts was implemented in a sixth-grade Cyprus classroom. Students were required to develop a model for their country to purchase water supplies from a choice of nearby nations (English & Mousoulides, 2011 ). Students were to consider travel distances, water price, available supply per week, oil tanker capacity, costs of water and oil, and quality of the port facilities in the neighbouring countries. The targeted model had to select the best option not only for the present but also for the future. Students’ models ranged from a basic form, where port facilities and water supply were ignored, to more sophisticated models, where all factors were integrated, with carbon emissions also considered. One of the student groups who took into account environmental factors commented, “It would be better for the country to spend a little more money and reduce oil consumption. And there are other environmental issues, like pollution of the Mediterranean Sea.” The more sophisticated models reflected systems thinking (Sect. 3.2 ), where the impact of partial factors such as oil consumption on the whole domain (ocean ecosystems and community) was also considered.
Philosophical Inquiry
One underrepresented means of fostering critical thinking in mathematical and STEM-based problem solving is through philosophical inquiry (Calvert et al., 2017 ; English, 2013 , 2022 ; Kennedy, 2012 ; Mukhopadhyay & Greer, 2007 ). Such inquiry encourages a range of thinking skills in identifying hidden assumptions, determining alternative courses of action, and reflecting on conclusions drawn and claims made. Several studies have shown how engaging children in communities of philosophical inquiry nurtures critical thinking dispositions, which become both a goal and a method (Bezençon, 2020 ; Daniel et al., 2017 ; Lipman, 2003 , 2008 ). At the same time, philosophical inquiry can lead to “conceptual deepening” (Bezençon, 2017), where analysis of mathematical and related STEM concepts as they apply beyond the classroom can be fostered. Given the increased societal awareness of mathematics and STEM in recent years, philosophical inquiry can be a powerful tool in enhancing students’ understanding and appreciation of how these disciplines shape societies. At the same time, philosophical inquiry can stimulate consideration of ethical issues in the applications of these disciplines (Bezençon, 2020 ). For example, Mukhopadhyay and Greer ( 2007 ) indicated how mathematics education should “convey the complexity of mathematical modeling social phenomena and a sense of what demarcates questions that can be answered by empirical evidence from those that depend on value systems and world-views” (p. 186).
A comprehensive review by O’Reilly et al. ( 2022 ) identified pedagogical approaches to scaffolding early critical thinking skills including inquiry-based teaching using classroom dialogue or questioning techniques. Such techniques include philosophical inquiry and encouraging children to construct, share, and justify their ideas regarding a task or investigation. Other opportunities for philosophical inquiry include group problem solving, peer sharing of created models, and facilitating critical and constructive peer feedback. For example, Gallagher and Jones ( 2021 ) reported on integrating mathematical modelling and economics, where beginning teachers were presented with a task involving a problematic community issue following a school shooting. In such cases, numerous courses of action are typically proposed for addressing the problem. Not surprisingly, various community opinions exist on such proposals, giving rise to valuable contexts for philosophical inquiry and critical modelling, where data and their sources are carefully analysed. With the escalation of statistical data from the mass media, it is imperative to commence the foundations of critical and philosophical thinking early. Students’ skills in asking critical questions as they work with data in constructing and improving a model, reflect on what their models convey, consider consequences of their models, and justify and communicate their conclusions require nurturing throughout school (Gibbs & Young Park, 2022 ).
Systems thinking
Systems thinking cuts across the STEM disciplines as well as many other fields outside education. It is considered a key component of “critical thinking and problem solving” in 21st Century Learning (P21, 2015 ) and is often cited as a “habit of mind” in engineering education (e.g., Lippard et al., 2018 ; Lucas et al., 2014 ). Numerous definitions exist for systems thinking (e.g., Bielik et al., 2022 ; Damelin et al., 2017 ; Jacobson & Wilenski, 2022 ), with Bielik et al. ( 2022 ) identifying such thinking as the ability to “consider the system boundaries, the components of the system, the interactions between system components and between different subsystems, and emergent properties and behaviour of the system” (p. 219). In more basic terms, Shin et al. ( 2022 ) refer to systems thinking as “the ability to understand a problem or phenomenon as a system of interacting elements that produces emergent behavior” (p. 936).
Systems thinking interacts with the other thinking forms including those displayed in Fig. 1 , as well as computational thinking (Shin et al., 2022), critical thinking (Curwin et al., 2018 ) and mathematical thinking more broadly (Baioa & Carreira, 2022 ). Systems thinking is considered especially important in conceptualizing a problematic situation within a larger context and in perceiving problems in new and different ways (Stroh, 2018 ). Of special relevance to today’s world is the realisation that perfect solutions do not exist and the choices one makes in applying systems thinking will impact on other parts of the system (Meadows, 2008 ). What is often not considered in today’s complex societies—at least not to the extent required—is that we live in a world of intrinsically linked systems, where disruption in one part will reverberate in others. We see so many instances where particular courses of action are advocated or mandated in societal systems, while the impact on sub-components is perilously ignored. Examples are evident in many nations’ responses to COVID-19, where escalating lockdowns impacted economies and communities, whose demands had to be balanced against purely epidemiological factors. The reverberations of such actions stretch far and wide over long periods. Likewise, the various impacts of current climate actions are frequently ignored, such as how the construction of vast areas of renewable resources (e.g., wind turbines) can have deleterious effects on the surrounding environments including wildlife.
Despite its centrality across the STEM domains, systems thinking is almost absent from mathematics education (Curwin et al., 2018 ). This is despite claims by many researchers that modelling, systems thinking, and associated thinking processes should be significant components of students’ education (Bielik et al., 2022 ; Jacobson & Wilenski, 2022 ). Indeed, systems thinking is featured prominently in the US A Framework for K-12 Science Education (NRC, 2012 ) and the Next Generation Science Standards (NGSS Lead States, 2013 ), and has received considerable attention in science education (e.g., Borge, 2016 ; Hmelo-Silver et al., 2017 ; York et al., 2019 ) and engineering education (e.g., Lippard & Riley, 2018 ; Litzinger, 2016 ).
Given the complexity of systems thinking in today’s world, and the diverse ways in which it is applied, students require opportunities to experience how systems thinking can interact with other forms such as design thinking and critical thinking (Curwin et al., 2018 ; Shin et al., 2022). Such interactions occur in many popular STEM investigations including one in which fifth-grade students designed, constructed, and experimented with a loaded paper plane (paper clips added) in determining how load impacts on the distance travelled (English, 2021b ). Students observed that changing one design feature (e.g., wingspan or load position) unsettles some other features (e.g., fuselage depth decreases wingspan). The investigation yielded an appreciation of the intricacy of interactions among a system’s components, and their scarcely predictable mutual effects.
Other studies have revealed how very young children can engage in basic systems thinking within STEM contexts. For example, Feriver et al. ( 2019 ) administered a story reading session to individual 4- to 6-year-old children in preschools in Turkey and Germany, and then followed this with individual semi-structured interviews about the story. The reading session was based on the story book, “The Water Hole” (Base, 2001 ), which draws on basic concepts of systems within an ecosystem context. Their study found the young children to have some complex understanding of systems thinking in terms of detecting obvious gradual changes and two-step domino and/or multiple one-way causalities, as well as describing the behaviour of a balancing loop (corrective actions that try to reduce the gap between a desired level or goal and the actual lever, such as temperature and plant growth). However, the children understandably experienced difficulties in several areas, in which even adults have problems. For example, detecting a reinforcing loop, identifying unintended consequences, detecting hidden components and processes, adopting a multi-dimensional perspective, and predicting how a system would behave in the future were problematic for them. In another study, Gillmeister ( 2017 ) showed how preschool children have a more complex understanding of systems thinking than previously claimed. Their ability to utilize simple systems thinking tools, such as stock-flow maps, feedback loops and behaviour over time graphs, was evident.
Although the research has not been extensive, current findings indicate how we might capitalise on the seeds of early systems thinking across the STEM fields. With the pervasive nature of systems thinking, it is argued that its connections to other forms of thinking across STEM should be nurtured (cf., Svensson, 2022 ). This includes links to design-based thinking where designed products, for example, operate “within broader systems and systems of systems” (Buchanan, 2019 ).
Design-based thinking and STEM-based problem solving
Design-based thinking plays a major role in complex problem solving, yet its contribution to mathematics learning has been largely ignored, especially at the primary levels. Design is central in technology and engineering practice (English et al., 2020 ; Guzey et al., 2019 ), from bridge construction through to the development of medical tools. Design contributes to all phases of problem solving and drives students toward innovative rather than predetermined outcomes (Goldman & Zielezinski, 2016 ). As applied to STEM education, design thinking is generally viewed as a set of iterative processes of understanding a problem and developing an appropriate solution. It includes problem scoping, idea generation, systems thinking, designing and creating, testing and reflecting, redesigning and recreating, and communicating. Both learning about design and learning through design can help students develop more informed analytical approaches to mathematical and STEM-based problem solving (English et al., 2020 ; Strimel et al., 2018 ).
Learning about design in solving complex problems emphasises design thinking processes per se, that is, students learn about design itself. Design thinking as a means for learning about design is not as prevalent in integrated STEM education. Although learning through and about design should not be divorced during problem solving (van Breukelen et al., 2017 ), one learning goal can take precedence over the other. Both require greater attention, especially in today’s design-focused world (Tornroth & Wikberg Nilsson, 2022 ).
A plurality of solutions of varying levels of sophistication is possible in applying design thinking processes, so students from different achievement levels can devise solutions (Goldman & Kabayadondo, 2017 )—and research suggests that lower-achieving students benefit most (Chin et al., 2019 ). The question of how best to develop design learning across grades K-12, however, has not received adequate attention. This is perhaps not surprising given that comparatively few studies have investigated the design thinking of grades 6–12 and even fewer across the elementary years (Kelley & Sung, 2017 ; Strimel et al., 2018 ).
Also overlooked is how design-based thinking can foster concept development, that is, how it can foster “learning while designing”, or generative learning (English et al., 2020 ). Design-based thinking provides natural opportunities to develop understanding of the required STEM concepts (Hjalmarson et al., 2020 ). As these authors pointed out, when students create designs, they represent models of their thinking. When students have to express their conceptual understanding in the form of design, such as an engineering designed product, their knowledge (e.g., of different material properties) is tested. Studies have revealed such learning occurs when elementary and middle school students design and construct various physical artifacts. For example, in a fifth-grade study involving designing and building an optical instrument that enabled one to see around corners, King and English ( 2017 ) observed how students applied and enriched their understanding of scientific concepts of light and how it travels through a system, together with their mathematical knowledge of geometric properties, angles and measurement.
In a study in the secondary grades (Langman et al. ( 2019 ), student groups completed modules that included a model-eliciting activity (Lesh & Zawojewski, 2007 ) involving iterative design thinking processes. Students were to interpret, assess, and compare images of blood vessel networks grown in scaffolds, develop a procedure or tool for measuring (or scoring) this vessel growth, and demonstrate how to apply their procedure to the images, as well as to any image of a blood vessel network. The results showed how students designed a range of mathematical models of varying levels of sophistication to evaluate the quality of blood vessel networks and developed a knowledge of angiogenesis in doing so.
In another study implemented in a 6th-grade classroom, students were involved in both learning about and learning through design in creating a new sustainable town with the goal of at least 50% renewable energy sources. The students were also engaged in systems thinking, as well as critical thinking, as they planned, developed, and critically analysed the layout and interactions of different components of their town system (e.g., where to place renewable energy sources to minimise impact on the residences and recreational areas; where to situate essential services to reach different town components). Students also had to consider the budgetary constraints in their town designs. Their learning about design was apparent in their iterative processes of problem scoping, idea generation and modification, balancing of benefits and trade-offs (dealing with system subcomponents), critical reflections on their designs, and improvements of the overall town design. Learning though design was evident as students displayed content knowledge pertaining to renewable energy and community needs, applied spatial reasoning in positioning town features, and considered budgetary constraints in reaching their “best” design.
Engineering design processes form a significant component of design thinking and learning, with foundational links across the STEM disciplines including mathematical modelling. Engineering design-based problems help students appreciate how they can apply different ideas and approaches from the STEM disciplines, with more than one outcome possible (Guzey et al., 2019 ). Such design thinking engages students in interpreting problems, developing initial design ideas, selecting and testing a promising design, analysing results from their prototype solution, and revising to improve outcomes.
It is of concern that limited attention has been devoted to engineering design-based thinking in mathematics and the other STEM disciplines in the primary grades. This is despite research indicating how very young children can engage in design-based thinking when provided with appropriate opportunities (e.g., Cunningham, 2018 ; Elkin et al., 2018 ). Elkin et al., for example, used robotics in early childhood classrooms to introduce foundational engineering design thinking processes. Their study illustrated how these young learners used engineering design journals and engaged in design thinking as they engineered creative solutions to challenging problems presented to them. It seems somewhat paradoxical that foundational engineering design thinking is a natural part of young children’s inquiry about their world, yet this important component has been largely ignored in these informative years.
In sum, design-based thinking is a powerful and integrative tool for mathematical and STEM-based problem solving and requires greater focus in school curricula. Both learning about and through design have the potential to improve learning and problem solving well beyond the school years (Chin et al., 2019 ). At the same time, design-based thinking, together with the other ways of thinking, can contribute significantly to learning innovation (English, 2018 ).
Adaptive and innovative thinking for learning innovation
Disruption is rapidly becoming the norm in almost all spheres of life. The recent national and international upheavals have further stimulated disruptive thinking (or disruptive innovation), where perspectives on commonly accepted (and often inefficient) solutions to problems are rejected for more innovative approaches and products. Given the pressing need for problem solvers capable of developing new and adaptable knowledge, rather than applying limited simplistic procedures or strategies, we need to foster what I term, learning innovation (English, 2018 ; Fig. 2 ). Such learning builds on core content to generate more powerful disciplinary knowledge and thinking processes that can, in turn, be adapted and applied to solving subsequent challenging problems.

Learning innovation
Figure 2 (adapted from McKenna, 2014 ) illustrates how learning innovation can be fostered through the growth of mathematical and STEM knowledge, together with STEM ways of thinking. The optimal adaptability corridor represents the growth of mathematical and STEM-based problem solving from novice to adaptable solver . Adaptable problem solvers (Hatano & Oura, 2003 ) have developed the flexibility, curiosity, and creativity to tackle novel problems—skills that are needed in jobs of the future (Denning & Noray, 2020 ; OECD, 2019 ). Without the disciplinary knowledge, ways of thinking, and engagement in challenging but approachable problems, a student risks remaining merely a routine problem solver—one who is skilled solely in applying previously taught procedures to solve sets of familiar well-worn problems, as typically encountered at school. Learning innovation remains a challenging and unresolved issue across curriculum domains and is proposed as central to dealing effectively with disruption.
Particularly rich experiences for developing learning innovation involve interdisciplinary modelling, incorporating critical mathematical modelling (e.g., Hallström and Schönborn, 2019 ; Lesh et al., 2013 ). As noted, a key feature of model-eliciting experiences is the affordances they provide all students to exhibit “extraordinary abilities to remember and transfer their tools to new situations” (Lesh et al., 2013 , p. 54). Modelling enables students to apply more sophisticated STEM concepts and generate solutions that extend beyond their usual classroom problems. Such experiences require different ways of thinking in problem solving as they deal with, for example, conflicting constraints and trade-offs, alternative paths to follow, and various tools and representations to utilise. In essence, interdisciplinary modelling may be regarded as a way of creating STEM concepts, with modeling and concept development being highly interdependent and mutually supportive (cf., Lesh & Caylor, 2007 ).
Concluding points
The ill-defined problems of today, coupled with unexpected disruptions across all walks of life, demand advanced problem-solving by all citizens. The need to update outmoded forms of problem solving, which fail to take into account increasing global challenges, has never been greater (Cowin, 2021 ). The ways-of-thinking framework has been proposed as a powerful means of enhancing problem-solving skills for dealing with today’s unprecedented game-changers. Specifically, critical thinking (including critical mathematical modelling and philosophical inquiry), systems thinking, and design-based thinking are advanced as collectively contributing to the adaptive and innovative skills required for problem success. It is argued that the pinnacle of this framework is learning innovation, which can be within reach of all students. Fostering students’ agency for developing learning innovation is paramount if they are to take some control of their own problem solving and learning (English, 2018 ; Gadanidis et al., 2016 ; Roberts et al., 2022 ).
Establishing a culture of empowerment and equity, with an asset-based approach, where the strengths of all students are recognised, can empower students as learners and achievers in an increasingly uncertain world (Celedón-Pattichis et al., 2018 ). Teacher actions that encourage students to express their ideas, together with a program of future-oriented mathematical and STEM-based problems, can nurture students’ problem-solving confidence and dispositions (Goldman & Zielezinski, 2016 ; Roberts et al., 2022 ). In particular, mathematical and STEM-based modelling has been advocated as a rich means of developing multiple ways of thinking that foster adaptive and innovative learners—learners with a propensity for developing new knowledge and skills, together with a willingness to tackle ill-defined problems of today and the future. Such propensity for dealing effectively with STEM-based problem solving is imperative, beginning in the earliest grades. The skills gained can thus be readily transferred across disciplines, and subsequently across career opportunities.
Acknowledgements
Sentiments expressed in this article have arisen from recent Australian Research Council grants # DP 220100303 and DP 150100120. Views expressed in this article are those of the author and not the Council.
Open Access funding enabled and organized by CAUL and its Member Institutions.
Data availability
Declarations.
There are no financial or non-financial interests that are directly or indirectly related to this submission.
Publisher's Note
Springer Nature remains neutral with regard to jurisdictional claims in published maps and institutional affiliations.
- Aguilar MS, Castaneda A. What mathematical competencies does a citizen need to interpret Mexico’s official information about the COVID-19 pandemic? Educational Studies in Mathematics. 2021 doi: 10.1007/s10649-021-10082-9. [ PMC free article ] [ PubMed ] [ CrossRef ] [ Google Scholar ]
- Aktoprak A, Hursen C. A bibliometric and content analysis of critical thinking in primary education. Thinking Skills and Creativity. 2022; 44 :101029. doi: 10.1016/j.tsc.2022.101029. [ PMC free article ] [ PubMed ] [ CrossRef ] [ Google Scholar ]
- Anhalt CO, Staats S, Cortez R, Civil M. Mathematical modeling and culturally relevant pedagogy. In: Dori YJ, Mevarech ZR, Baker DR, editors. Cognition, metacognition, and culture in STEM education. Springer; 2018. pp. 307–330. [ Google Scholar ]
- Ärlebäck JB, Doerr HM. Students’ interpretations and reasoning about phenomena with negative rates of change throughout a model development sequence. ZDM Mathematics Education. 2018; 50 (1–2):187–200. doi: 10.1007/s11858-017-0881-5. [ CrossRef ] [ Google Scholar ]
- Aviso KB, Yu CD, Lee J-Y, Tan RR. P-graph optimization of energy crisis response in Leontief systems with partial substitution. Cleaner Energy and Technology. 2022; 9 :100510. doi: 10.1016/j.clet.2022.100510. [ CrossRef ] [ Google Scholar ]
- Baioa, A. M., & Carreira, S. (2022). Mathematical thinking about systems—Students modeling a biometrics identity verification system. Mathematical Thinking and Learning.
- Bakker A, Wagner D. Pandemic: Lessons for today and tomorrow? Educational Studies in Mathematics. 2020; 104 :1–4. doi: 10.1007/s10649-020-09946-3. [ CrossRef ] [ Google Scholar ]
- Barbosa JC. Mathematical modelling in classroom: A socio-critical and discursive perspective. ZDM Mathematics Education. 2006; 38 :293–301. doi: 10.1007/BF02652812. [ CrossRef ] [ Google Scholar ]
- Base G. In: The Water Hole. Harry N, editor. Abrs Inc.; 2001. [ Google Scholar ]
- Benessiaa A, De Marchi B. When the earth shakes … and science with it. The management and communication of uncertainty in the L’Aquila earthquake. Futures. 2017; 91 :35–45. doi: 10.1016/j.futures.2016.11.011. [ CrossRef ] [ Google Scholar ]
- Bezençon, E. (2020). Practising philosophy of mathematics with children: what, why and how? Philosophy of Mathematics Education Journal, 36 .
- Bielik T, Stephens L, McIntyre C, Damelin D, Krajcik JS. Supporting student system modelling practice through curriculum and technology design. Journal of Science Education and Technology. 2022; 31 :217–231. doi: 10.1007/s10956-021-09943-y. [ CrossRef ] [ Google Scholar ]
- Blum W, Leiss D. How do students and teachers deal with mathematical modelling problems? In: Haines C, Galbraith PL, Blum W, Khan S, editors. Mathematical modelling (ICTMA12)—Education, engineering and economics. Horwood; 2007. [ Google Scholar ]
- Borge M. Systems thinking as a design problem. In: Duschl RA, Bismack AS, editors. Reconceptualizing STEM education. Routledge; 2016. pp. 68–80. [ Google Scholar ]
- Brady C, Lesh R, Sevis S, et al. Extending the reach of the models and modelling perspective: A course-sized research site. In: Stillman GA, et al., editors. Mathematical modelling in education research and practice. Springer; 2015. pp. 55–66. [ Google Scholar ]
- Braund M. Critical STEM Literacy and the COVID-19 Pandemic. Canadian Journal of Science, Mathematics, and Technology Education. 2021; 21 :39–356. doi: 10.1007/s42330-021-00150-w. [ CrossRef ] [ Google Scholar ]
- Buchanan R. Systems thinking and design thinking: the search for principles in the world we are making. She Ji the Journal of Design, Economics, and Innovation. 2019; 5 (2):85–104. doi: 10.1016/j.sheji.2019.04.001. [ CrossRef ] [ Google Scholar ]
- Calvert K, Forster M, Hausberg A, et al. Philosophizing with children in science and mathematics classes. In: Rollins Gregory M, Haynes J, Murris K, et al., editors. The Routledge international handbook of philosophy for children. Routledge; 2017. pp. 191–199. [ Google Scholar ]
- Celedón-Pattichis S, Lunney Borden L, Pape SJ, et al. Asset-based approaches to equitable mathematics education research and practice. Journal for Research in Mathematics Education. 2018; 49 (4):373–389. doi: 10.5951/jresematheduc.49.4.0373. [ CrossRef ] [ Google Scholar ]
- Charles R. The role of problem solving. Arithmetic Teacher. 1985; 32 :48–50. doi: 10.5951/AT.32.6.0048. [ CrossRef ] [ Google Scholar ]
- Chin DB, Blair KP, Wolf RC, Conlin LD, Cutumisu M, Pfaffman J, Schwartz DL. Educating and measuring choice: A test of the transfer of design thinking in problem solving and learning. Journal of the Learning Sciences. 2019; 28 (3):337–380. doi: 10.1080/10508406.2019.1570933. [ CrossRef ] [ Google Scholar ]
- Cirillo M, Bartell TG, Wager AA. Teaching mathematics for social justice through mathematical modeling. In: Hirsch CR, RothMcDuffie AR, editors. Mathematical modeling and modeling mathematics. National Council of Teachers of Mathematics; 2016. pp. 87–96. [ Google Scholar ]
- Cowin J. The fourth industrial revolution: Technology and education. Journal of Systemics, Cybernetics and Informatics. 2021; 19 (8):53–63. doi: 10.54808/JSCI.19.08.53. [ CrossRef ] [ Google Scholar ]
- Crittenden, AB, Crittenden VI, Crittenden, WF (2017) Industry transformation via channel disruption. Journal of Marketing Channels , 24 (1–2):13–26.
- Cunningham CM. Engineering in elementary STEM education: Curriculum design, instruction, learning, and assessment. Teachers College Press; 2018. [ Google Scholar ]
- Curwin MS, Ardell A, MacGillivray L, Lambert R. Systems thinking in a second grade curriculum: Students engaged to address a statewide drought. Frontiers in Education. 2018 doi: 10.3389/feduc.2018.00090. [ CrossRef ] [ Google Scholar ]
- Dalal M, Carberry A, Archambault L, et al. Developing a ways of thinking framework for engineering education research. Studies in Engineering Education. 2021; 1 (2):108–125. doi: 10.21061/see.38. [ CrossRef ] [ Google Scholar ]
- Damelin D, Krajcik J, Mcintyre C, Bielik T. Students making systems models: An accessible approach. Science Scope. 2017; 40 (5):78–82. doi: 10.2505/4/ss17_040_05_78. [ CrossRef ] [ Google Scholar ]
- Daniel M, Gagnon M, Auriac-Slusarczyk E. Dialogical critical thinking in kindergarten and elementary school: Studies on the impact of philosophical praxis in pupils. In: Gregory MR, Haynes J, Murris K, editors. The Routledge international handbook of philosophy for children. Routledge; 2017. pp. 236–244. [ Google Scholar ]
- Denning DJ, Noray K. Earnings dynamics, changing job skills, and STEM careers. The Quarterly Journal of Economics. 2020; 135 (4):1965–2005. doi: 10.1093/qje/qjaa021. [ CrossRef ] [ Google Scholar ]
- Dong Y, Wang J, Yang Y, Karup PM. Understanding intrinsic challenges to STEM instructional practices for Chinese teachers based on their beliefs and knowledge base. International Journal of STEM Education. 2020; 7 :47. doi: 10.1186/s40594-020-00245-0. [ CrossRef ] [ Google Scholar ]
- Eleyyan S. The future of education according to the fourth industrial revolution. Journal of Educational Technology and Online Learning. 2021; 4 (1):23–30. [ Google Scholar ]
- Elkin M, Sullivan A, Bers MU. Books, butterflies, and ’bots: Integrating engineering and robotics into early childhood curricula. In: English LD, Moore T, editors. Early engineering learning. Springer; 2018. pp. 225–248. [ Google Scholar ]
- Engel M, Claessens A, Watts T, Farkas G. Mathematics content coverage andstudent learning in kindergarten. Educational Researcher. 2016; 45 (5):293–300. doi: 10.3102/0013189X16656841. [ PMC free article ] [ PubMed ] [ CrossRef ] [ Google Scholar ]
- English LD. Modeling as a vehicle for philosophical inquiry in the mathematics classroom. The Journal of Analytic Teaching and Philosophical Praxis. 2013; 34 (1):46–57. [ Google Scholar ]
- English LD. STEM education K-12: Perspectives on integration. International Journal of STEM Education. 2016; 3 (1):1–12. doi: 10.1186/s40594-016-0036-1. [ CrossRef ] [ Google Scholar ]
- English, L. D. (2018). Disruption and learning innovation across STEM. Plenary presented at the 5th International Conference of STEM in Education , Brisbane. https://stem-in-ed2018.com.au/proceedings-2/
- English LD. Mathematical and interdisciplinary modeling in optimizing young children’s learning. In: Suh J, Wickstrom M, English LD, editors. Exploring mathematical modeling with young learners. Springer; 2021. pp. 3–24. [ Google Scholar ]
- English LD. Facilitating STEM integration through design. In: Anderson J, Li Y, editors. Integrated approaches to STEM education: An international perspective. Springer; 2021. pp. 45–66. [ Google Scholar ]
- English LD. Mathematical modeling and philosophical inquiry in the elementary school. In: Kennedy N, editor. Dialogical inquiry in mathematics teaching and learning: A philosophical approach. Lit Verlag; 2022. [ Google Scholar ]
- English LD, Adams R, King D. Learning by design across STEM education. In: Johnson CJ, Schroeder MM, Moore T, English LD, editors. Handbook of research on STEM education. Routledge; 2020. pp. 76–96. [ Google Scholar ]
- English LD, Gainsburg J. Problem solving in a 21st-century mathematics curriculum. In: English LD, Kirshner D, editors. Handbook of international research in mathematics education. 3. Taylor & Francis; 2016. pp. 313–335. [ Google Scholar ]
- English LD, Mousoulides N. Engineering-based modelling experiences in the elementary classroom. In: Khine MS, Saleh IM, editors. Models and modeling: Cognitive tools for scientific enquiry. Springer; 2011. pp. 173–194. [ Google Scholar ]
- Ericson JD. Mapping the relationship between critical thinking and design thinking.Journal of the. Knowledge Economy. 2022; 13 :406–429. doi: 10.1007/s13132-021-00733-w. [ CrossRef ] [ Google Scholar ]
- Feriver S, Olgan R, Teksöz G, Barth M. Systems thinking skills of preschool children in early childhood education contexts from Turkey and Germany. MDPI: Sustainability. 2019; 11 :1478. [ Google Scholar ]
- Gadanidis G, Hughes JM, Minniti L, White BJ. Computational thinking, Grade 1 students and the binomial theorem. Digital Experiences in Mathematics Education. 2016 doi: 10.1007/s40751-016-0019-3. [ CrossRef ] [ Google Scholar ]
- Gallagher MA, Jones JP. Supporting students’ critical literacy: Mathematical modeling and economic decisions. In: Suh J, Wickstrom M, English LD, editors. Exploring mathematical modeling with young learners. Springer; 2021. pp. 373–388. [ Google Scholar ]
- Gibbs AM, Young Park J. Unboxing mathematics: Creating a culture of modeling as critic. Educational Studies in Mathematics. 2022; 110 :167–192. doi: 10.1007/s10649-021-10119-z. [ PMC free article ] [ PubMed ] [ CrossRef ] [ Google Scholar ]
- Gillmeister KM. Development of early conceptions in systems thinking in an environmental context: An exploratory study of preschool students' understanding of stocks and flows. State University of New York; 2017. [ Google Scholar ]
- Goldman S, Kabayadondo Z, editors. Taking Design Thinking to School: How the technology of design can transform teachers, learners, and classrooms. Routledge; 2017. pp. 3–19. [ Google Scholar ]
- Goldman S, Zielezinski MB. Teaching with design thinking: developing newvision and approaches to twenty-first century learning. In: Annetta L, Minogue J, editors. Connecting science and engineering education practices in meaningful ways. Contemporary trends and issues in science education. Springer; 2016. p. 44. [ Google Scholar ]
- Greer B. Estimating Iraqi deaths: A case study with implications for mathematics education. ZDM Mathematics Education. 2009; 41 (1–2):105–116. doi: 10.1007/s11858-008-0147-3. [ CrossRef ] [ Google Scholar ]
- Greer B, Verschaffel L, Mukhopadhyay S. Modeling for life: Mathematics and children’s experience. In: Blum W, Henne W, Niss M, editors. Applications and modeling in mathematics education (ICMI study 14) Kluwer; 2007. pp. 89–98. [ Google Scholar ]
- Guzey SS, Ring-Whalen EA, Harwell M, Peralta Y. Life STEM: A case study of life science learning through engineering design. International Journal of Science and Mathematics Education. 2019; 17 (1):23–42. doi: 10.1007/s10763-017-9860-0. [ CrossRef ] [ Google Scholar ]
- Hallström J, Schönborn KJ. Models and modelling for authentic STEM education: reinforcing the argument. International Journal of STEM Education. 2019; 6 :22. doi: 10.1186/s40594-019-0178-z. [ CrossRef ] [ Google Scholar ]
- Hatano G, Oura Y. Commentary: Reconceptualizing school learning using insight from expertise research. Educational Researcher. 2003; 32 (8):26–29. doi: 10.3102/0013189X032008026. [ CrossRef ] [ Google Scholar ]
- Hjalmarson MA, Holincheck N, Baker CK, Galanti TM. Learning models and modeling across the STEM disciplines. In: Johnson CJ, Schroeder MM, Moore T, English LD, editors. Handbook of research on STEM education. Routledge; 2020. pp. 223–233. [ Google Scholar ]
- Hmelo-Silver CE, Jordan R, Eberbach C, Sinha S. Systems learning with a conceptual representation: A quasi-experimental study. Instructional Science. 2017; 45 :53–72. doi: 10.1007/s11251-016-9392-y. [ CrossRef ] [ Google Scholar ]
- Jablonka E. Critical thinking in mathematics education. In: Lerman S, editor. Encyclopedia of mathematics education. Springer; 2020. [ Google Scholar ]
- Jacobson MJ, Wilenski U. Complex systems and the learning sciences: Educational, theoretical, and methodological implications. In: Sawyer K, editor. The Cambridge handbook of the learning sciences. Cambridge University Press; 2022. pp. 504–522. [ Google Scholar ]
- Kazemi E. Mathematical modeling with young learners: A commentary. In: Suh J, Wickstrom M, English LD, editors. Exploring mathematical modeming with younglearners. Springer; 2021. pp. 337–342. [ Google Scholar ]
- Kelley TR, Sung E. Examining elementary school students’ transfer of learning through engineering design using think-aloud protocol analysis. Journal of Technology Education. 2017; 28 (2):83–108. doi: 10.21061/jte.v28i2.a.5. [ CrossRef ] [ Google Scholar ]
- Kelley TR, Knowles JG. A conceptual framework for integrated STEMeducation. International Journal of STEM Education. 2016; 3 (1):1–11. doi: 10.1186/s40594-016-0046-z. [ CrossRef ] [ Google Scholar ]
- Kennedy NS. What are you assuming? Mathematics Teaching in the Middle School. 2012; 18 (2):86–91. doi: 10.5951/mathteacmiddscho.18.2.0086. [ CrossRef ] [ Google Scholar ]
- King DT, English LD. Engineering design in the primary school: Applying STEM concepts to build an optical instrument. International Journal of Science Education. 2017; 38 :2762–2794. doi: 10.1080/09500693.2016.1262567. [ CrossRef ] [ Google Scholar ]
- Koh JHL, Chai CS, Wong B, Hong H-Y. Design thinking for education: Conceptions and applications in teaching and learning. Springer; 2015. [ Google Scholar ]
- Kollosche D. Styles of reasoning for mathematics education. Educational Studies inMathematics. 2021; 107 (3):471–486. [ Google Scholar ]
- Kollosche D, Meyerhöfer W. COVID-19, mathematics education, and the evaluation of expert knowledge. Educational Studies in Mathematics. 2021; 108 :401–417. doi: 10.1007/s10649-021-10097-2. [ PMC free article ] [ PubMed ] [ CrossRef ] [ Google Scholar ]
- Krause CM, Di Martino P, Moschkovich JN. Tales from three countries: Reflections during COVID-19 for mathematical education in the future. Educational Studies in Mathematics. 2021; 108 :87–104. doi: 10.1007/s10649-021-10066-9. [ PMC free article ] [ PubMed ] [ CrossRef ] [ Google Scholar ]
- Langman C, Zawojewski J, McNicholas P, Cinar A, Brey E, Bilgic M, Mehdizadeh H. Disciplinary learning from an authentic engineering context. Journal of Pre-College Engineering Education Research. 2019; 9 :77–94. [ Google Scholar ]
- Larson M (2017) Math education is STEM education! NCTM president’s message . Retrieved from https://www.nctm.org/News-and-Calendar/Messages-from-the-President/Archive/Matt-Larson/Math-Education-Is-STEM-Education .
- Lesh R, Caylor B. Introduction to the special issue: Modeling as application versus modeling as a way to create mathematics. International Journal of Computers for Mathematical Learning. 2007; 12 (3):173–194. doi: 10.1007/s10758-007-9121-3. [ CrossRef ] [ Google Scholar ]
- Lesh R, English LD, Riggs C, Sevis S. Problem solving in the primary school (K-2) Mathematics Enthusiast. 2013; 10 (1):35–60. doi: 10.54870/1551-3440.1259. [ CrossRef ] [ Google Scholar ]
- Lesh R, Zawojewski JS. Problem solving and modeling. In: Lester F, editor. Second handbook of research on mathematics teaching and learning. Information Age Publishing; 2007. pp. 763–804. [ Google Scholar ]
- Lester F, Cai F. Can mathematical problem solving be taught? In: Felmer P, Pehkonen E, Kilpatrick J, editors. Posing and solving mathematical problems. Springer; 2016. pp. 117–136. [ Google Scholar ]
- Li, Y, Schoenfeld A H (2019) Problematizing teaching and learning mathematics as ‘given’ in STEM education. International Journal of STEM Education, 6 , 44. 10.1186/s40594-019-0197-9.
- Lipman M. Thinking in education. 2. Cambridge University Press; 2003. [ Google Scholar ]
- Lipman M. A life teaching thinking. The Institute for the Advancement of Philosophy for Children; 2008. [ Google Scholar ]
- Lippard CN, Riley KL, Mann MH. Encouraging the development of engineering habits of mind in prekindergarten learners. In: English LD, Moore TJ, editors. Early engineering learning. Springer; 2018. pp. 19–37. [ Google Scholar ]
- Litzinger TA. Thinking about a system and systems thinking in engineering. In: Duschl RA, Bismack AS, editors. Reconceptualizing STEM education. Routledge; 2016. pp. 35–48. [ Google Scholar ]
- Lucas, B., Claxton, G., & Hanson, J. (2014). Thinking like an engineer: Implications for the education system. A report for the Royal Academy of Engineering Standing Committee for Education and Training.
- Maass K, Geiger V, Romero Ariza M, Goos M. The role of mathematics in interdisciplinary STEM education. ZDM Mathematics Education. 2019; 51 :869–884. doi: 10.1007/s11858-019-01100-5. [ CrossRef ] [ Google Scholar ]
- Mayes R. Quantitative reasoning and its role in interdisciplinarity. In: Doig B, Williams J, Swanson D, Borromeo Ferri R, Drake P, editors. Interdisciplinary Mathematics Education. ICME-13 Monographs. Springer; 2019. [ Google Scholar ]
- McKenna AF. Adaptive expertise and knowledge fluency in design and innovation. In: Johri A, Olds BM, editors. Cambridge handbook of engineering education research. Cambridge University Press; 2014. pp. 227–242. [ Google Scholar ]
- Meadows, D. (2008). Thinking in systems: A primer (Chelsea Green Publishing). https://www.chelseagreen.com/product/thinking-in-systems .
- Mukhopadhyay S, Greer B. How many deaths? Education for statistical empathy. In: Sriraman B, editor. International perspectives on social justice in mathematics education. Information Age Publishing; 2007. pp. 169–189. [ Google Scholar ]
- National Research Council (NRC) A framework for K-12 science education: Practices, crosscutting concepts, and core ideas. National Academies Press; 2012. [ Google Scholar ]
- National Science and Technological Council. (2018). Charting a course for success: America’s strategy for STEM education. Committee on STEM Education of the National Science and Technological Council . https://www.whitehouse.gov/wp-content/uploads/2018/12/STEM-Education-Strategic-Plan-2018.pdf .
- NGSS Lead States. (2013). Next generation science standards: For states, by states (The National Academic Press). http://www.nextgenscience.org/next-generation-science-standards .
- Nichols RK, Burgh G, Fynes-Clinton L. Reconstruction of thinking across the curriculum through the community of inquiry. In: Rollins Gregory M, Haynes J, Murris K, editors. The Routledge international handbook of philosophy for children. Routledge; 2019. pp. 245–252. [ Google Scholar ]
- OECD (2019). PISA 2022 Mathematics Framework . https://pisa2022-maths.oecd.org/ca/index.html .
- O’Reilly C, Devitt A, Hayes N. Critical thinking in the preschool classroom—A systematic literature review. Thinking Skills and Creativity. 2022 doi: 10.1016/j.tsc.2022.101110. [ CrossRef ] [ Google Scholar ]
- Organisation for Economic Co-operation and Development (OECD) Education at a glance 2019: OECD indicators. OECD; 2019. [ Google Scholar ]
- Organisation for Economic Co-operation and Development (OECD) Thinking Outside the box: The PISA 2022 Creative Thinking Assessment. OECD; 2022. [ Google Scholar ]
- Oxford Economics (2022). Global economic model . https://www.oxfordeconomics.com/service/subscription-services/macro/global-economic-model/ .
- P21 Partnership for 21st Century Learning (2015). http://www.p21.org/our-work/p21-framework .
- Park D-Y, Park M-H, Bates AB. Exploring young children’s understanding about the concept of volume through engineering design in a STEM activity: A case study. International Journal of Science and Mathematics Education. 2018; 16 (2):275–294. doi: 10.1007/s10763-016-9776-0. [ CrossRef ] [ Google Scholar ]
- Rhodes T, Lancaster K. Mathematical models as public troubles in COVID-19 infection control. Health Sociology Review. 2020; 29 :177–194. doi: 10.1080/14461242.2020.1764376. [ PubMed ] [ CrossRef ] [ Google Scholar ]
- Rhodes T, Lancaster K, Rosengarten M. A model society: Maths, models and expertise in viral outbreaks. Critical Public Health. 2020; 30 (3):253–256. doi: 10.1080/09581596.2020.1748310. [ CrossRef ] [ Google Scholar ]
- Roberts T, Maiorca C, Jackson C, Mohr-Schroeder M. Integrated STEM as problem-solving practices. Investigations in Mathematics Learning. 2022 doi: 10.1080/19477503.2021.2024721. [ CrossRef ] [ Google Scholar ]
- Rollins Gregory M, Haynes J, Murris K, editors. The Routledge international handbook of philosophy for children. Routledge; 2017. [ Google Scholar ]
- Shaughnessy, M. (2013). By way of introduction: Mathematics in a STEM context. Mathematics Teaching in the Middle School , 18 (6):324.
- Shin N, Bowers J, Roderick S, McIntyre C, Stephens AL, Eidin E, Krajcik J, Damelin D. A framework for supporting systems thinking and computationalthinking through constructing models. Instructional Science. 2022; 50 (6):933–960. doi: 10.1007/s11251-022-09590-9. [ CrossRef ] [ Google Scholar ]
- Slavit D, Grace E, Lesseig K. Student ways of thinking in STEM contexts: A focus on claim making and reasoning. School Science and Mathematics. 2021; 21 (8):466–480. doi: 10.1111/ssm.12501. [ CrossRef ] [ Google Scholar ]
- Slavit D, Lesseig K, Simpson A. An analytic framework for understanding student thinking in STEM contexts. Journal of Pedagogical Research. 2022; 6 (2):132–148. doi: 10.33902/JPR.202213536. [ CrossRef ] [ Google Scholar ]
- Stehle SM, Peters-Burton EE. Developing student 21st century skills in selected exemplary inclusive STEM high schools. International Journal of STEM Education. 2019; 6 :39. doi: 10.1186/s40594-019-0192-1. [ CrossRef ] [ Google Scholar ]
- Strimel GJ, Bartholomew SR, Kim E, Zhang L. An investigation of engineering design cognition and achievement in primary school. Journal for STEM Education Research. 2018 doi: 10.1007/s41979-018-0008-0. [ CrossRef ] [ Google Scholar ]
- Stroh, D. P. (2018). The systems orientation: From curiosity to courage . https://thesystemsthinker.com/the-systems-orientation-from-curiosity-to-courage/
- Svensson M. Cross-curriculum system concepts and models. In: Hallström J, Williams PJ, editors. Teaching and learning about technological systems. Springer; 2022. pp. 53–72. [ Google Scholar ]
- Teng B, Wang S, Shi Y, Sun Y, Wang W, Hu W, Shi C. Economic recovery forecasts under impacts of COVID-19. Economic Modelling. 2022; 110 :105821. doi: 10.1016/j.econmod.2022.105821. [ PMC free article ] [ PubMed ] [ CrossRef ] [ Google Scholar ]
- Tornroth S, WikbergNilsson A. Design thinking for the everyday aestheticisation of urban renewable energy. Design Studies. 2022; 79 :101096. doi: 10.1016/j.destud.2022.101096. [ CrossRef ] [ Google Scholar ]
- Turner E, Bennett A, Granillo M, et al. Authenticity of elementary teacher designed and implemented mathematical modeling tasks. Mathematical Thinking and Learning: an International Journal. 2022; 2022 :1–24. doi: 10.1080/10986065.2022.2028225. [ CrossRef ] [ Google Scholar ]
- Turner E, Roth McDuffie A, Aguirre J, Foote MQ, Chapelle C, Bennett A, Granillo M, Ponnuru N. Upcycling plastic bags to make jump ropes: Elementary students leverage experiences and funds of knowledge as they engage in a relevant, community oriented mathematical modeling task. In: Suh J, Wickstram M, English L, editors. Exploring mathematical modeling with young learners. Springer; 2021. pp. 235–266. [ Google Scholar ]
- Turner E, Varley M, Simic K, Diaz-Palomar J. “Everything is math in the Whole World!”: Integrating critical and community knowledge in authentic mathematical investigations with elementary latina/o students. Mathematical Thinking and Learning: An International Journal. 2009; 11 (3):136–157. doi: 10.1080/10986060903013382. [ CrossRef ] [ Google Scholar ]
- Tytler R (2016) Drawing to learn in STEM. Proceedings of the ACER Research Conference: Improving STEM learning: What will it take? (pp. 45-50). Australian Council for Educational Research.
- van Breukelen DHJ, de Vries MJ, Schure FA. Concept learning by direct current design challenges in secondary education. International Journal of Technology and Design Education. 2017; 27 :407–430. doi: 10.1007/s10798-016-9357-0. [ CrossRef ] [ Google Scholar ]
- York S, Lavi R, Dori YJ, Orgill M. Applications of systems thinking in STEM education. Journal of Chemical Education. 2019; 96 :2742–2751. doi: 10.1021/acs.jchemed.9b00261. [ CrossRef ] [ Google Scholar ]
- Zawojewski J, Hjalmarson M, Bowman KJ, Lesh R, editors. Models and modeling in engineering education. Brill; 2008. [ Google Scholar ]
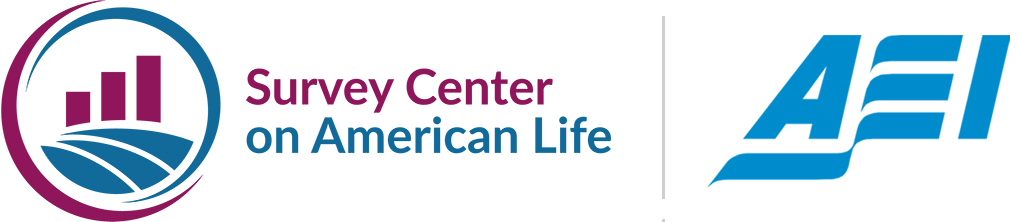
STEM Perspectives: Attitudes, Opportunities, and Barriers in America’s STEM Workforce
July 15, 2020 | Daniel A. Cox
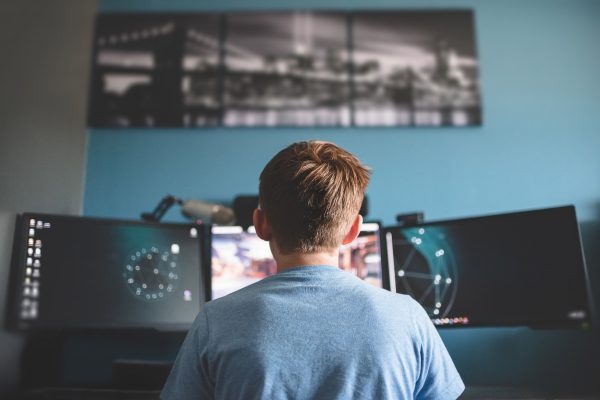
- A strong majority of STEM graduates are satisfied in their jobs, and relatively few regret their major choice. When asked if they would recommend a STEM career to a young person, 95 percent of STEM workers with a STEM degree say yes.
- At the same time, over half of STEM degree-holders say the phrase “people feel like they are generally replaceable” describes the field very or somewhat well, and over 40 percent say that STEM employers would rather hire new workers than train current ones.
- Forty percent of STEM degree-holders say women face more obstacles in STEM than they do in other industries, 30 percent say the same of African Americans, and nearly 50 percent say the same of older Americans. Further, women and associate degree-holders are significantly more likely to leave the STEM field than are their male or bachelor’s and graduate degree-holding counterparts.
- STEM workers say that noncognitive skills such as writing and communication are significantly more important on the job than high-level math, analytical, or computer skills.
Read the PDF.
Introduction
STEM disciplines are often recommended as the most direct path between education and a good, high-wage career. From public policy to student career counseling, encouraging STEM credentials and degrees has been at the center of American educational, employment, and economic strategy for decades. The question arises whether we have gone too far in promoting STEM as a pathway to career success. What do current and former STEM workers think about the field in which they work?
In a new national survey, AEI examines how people with STEM degrees perceive the working conditions, opportunities, and barriers to success in STEM occupations. For STEM-credentialed individuals who either never worked in STEM or have left the field, we also explore their reasons for opting for other career pathways.
Like most workers, the survey finds that a strong majority of STEM graduates are satisfied in their jobs. 1 Relative to other degree holders, few STEM graduates regret their major choice, 2 and most are confident that the STEM field offers about the same or better opportunities than other fields do in pay, opportunities for advancement, ability to make a meaningful contribution to society, collegiality among colleagues, and more. Although there is some variation among subgroups, most current STEM workers do not seem concerned about the impact of automation or foreign competition on their jobs, and relatively few have seriously considered leaving the field. When asked if they would recommend a STEM career to a young person, 95 percent of STEM workers with a STEM degree said yes.
Some responses, however, provide cause for concern—especially for women, minorities, and workers with less than a bachelor’s degree in STEM occupations. Over half of STEM degree holders say the statement “people feel like they are generally replaceable” describes the field very or somewhat well, and more than four in 10 say that employers would rather hire new workers than train current ones. Women are more likely than men are to regret choosing a STEM degree, and over 40 percent of STEM degree holders say women face more obstacles in STEM than they do in other fields. (Among female respondents, this number rises to over 50 percent.) Similarly, over 30 percent of all STEM degree holders say black Americans face more obstacles in STEM than they do in other industries (more than 40 percent of nonwhite respondents say the same), and nearly half of current STEM workers say older Americans face more barriers in STEM than they do in other fields.
The survey also examines who is leaving STEM and why. Among those who have left STEM during their working life, the most common reasons cited are a change in career interests or family responsibilities, while about four in 10 point to additional factors such as feeling undervalued, lack of competitive salary or benefits, and few opportunities for advancement. Women and associate degree holders are more likely to leave the STEM field than are their male or bachelor’s and graduate degree-holding counterparts. There is at least some evidence that those with less than a bachelor’s degrees are more likely to cite a lack of opportunity for advancement in the workplace and job elimination from automation as reasons for leaving the field. 3
Consistent with recent literature, the survey finds that STEM workers identify noncognitive and interpersonal skills as important in their job, especially among female STEM workers and older workers who are more advanced in their careers. Nearly half of STEM workers say good written and communication skills are extremely important in their job, and nearly 70 percent say the same of critical thinking skills. By comparison, only 36 percent of STEM workers said high-level math, analytical, or computer skills—generally thought to be core skills for STEM occupations—were extremely important in their job. Notably, the importance of noncognitive or implicit skills on the job increases as workers age, while the importance of technical skills remains relatively constant.
Findings in the survey will provide workers, employers, policymakers, and concerned citizens with a more nuanced picture of the experience and outlook of STEM graduates in the workforce. While the education and media narrative around STEM centers on the outsized influence of Big Tech and a belief that STEM training offers a sure path to career security, AEI’s survey of STEM graduates provides the reader with fresh perspective on STEM through the eyes of STEM workers. For a majority of STEM graduates, a STEM career provides a path to a fulfilling and sustainable career. For others—particularly minorities, women, and those with less education—the reality is more complex, revealing a need for greater intentionality by employers, educators, and policymakers to promote a more holistic training agenda and a more inclusive STEM workplace environment.
Read the full report.
Like most workers, the survey finds that a strong majority of STEM graduates are satisfied in their jobs. 1 Relative to other degree holders, few STEM graduates regret their major choice, 2 and most are confident that the STEM field offers about the same or better opportunities than other fields do in pay, opportunities for advancement, ability to make a meaningful contribution to society, collegiality among colleagues, and more. Although there is some variation among subgroups, most current STEM workers do not seem concerned about the impact of automation or foreign competition on their jobs, and relatively few have seriously considered leaving the field. When asked if they would recommend a STEM career to a young person, 95 percent of STEM workers with a STEM degree said yes.
- For example, a 2016 Pew Research Center survey found that nearly 80 percent of American workers were “very” or “somewhat” satisfied with their current job. Pew Research Center, “The State of American Jobs,” October 6, 2016, https://www.pewsocialtrends.org/2016/10/06/3-how-americans-view-their-jobs/ .
- A 2017 Strada-Gallup poll found that 40 percent of bachelor’s degree holders say they would have chosen a different major. By contrast, only 20 percent of STEM graduates say they would have chosen a different major. Strada Education Network and Gallup, “On Second Thought: U.S. Adults Reflect on Their Education Decisions,” June 2017, https://news.gallup.com/reports/225161/2017-strada-gallup-college-student-survey.aspx .
- There are only 80 associate degree holders who formerly worked in STEM in the sample. Results should be interpreted with caution.
Survey Reports
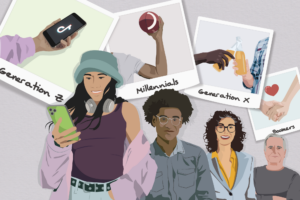
Daniel A. Cox, Kelsey Eyre Hammond, Kyle Gray November 9, 2023
Generation Z and the Transformation of American Adolescence: How Gen Z’s Formative Experiences Shape Its Politics, Priorities, and Future
This report explores the foundational differences between American generations through their formative adolescent experiences.
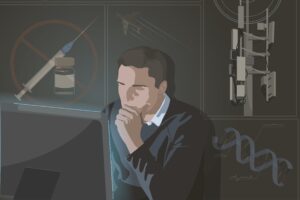
Daniel A. Cox, M. Anthony Mills, Ian R. Banks, Kelsey Eyre Hammond, Kyle Gray September 28, 2023
America’s Crisis of Confidence: Rising Mistrust, Conspiracies, and Vaccine Hesitancy After COVID-19
America is experiencing a crosscutting crisis of expertise and scientific distrust accelerated by the COVID-19 pandemic that poses significant challenges to democratic debate and public decision-making
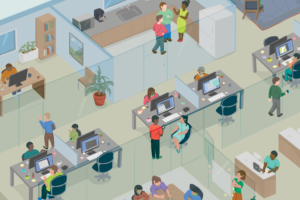
Daniel A. Cox, Brent Orrell, Kyle Gray, Jessie Wall September 14, 2023
The Social Workplace: Social Capital, Human Dignity, and Work in America, Volume II
The Social Workplace, Volume II examines Americans’ expectations and experiences surrounding work, the workplace, and key job-related priorities such as pay and interpersonal connections.
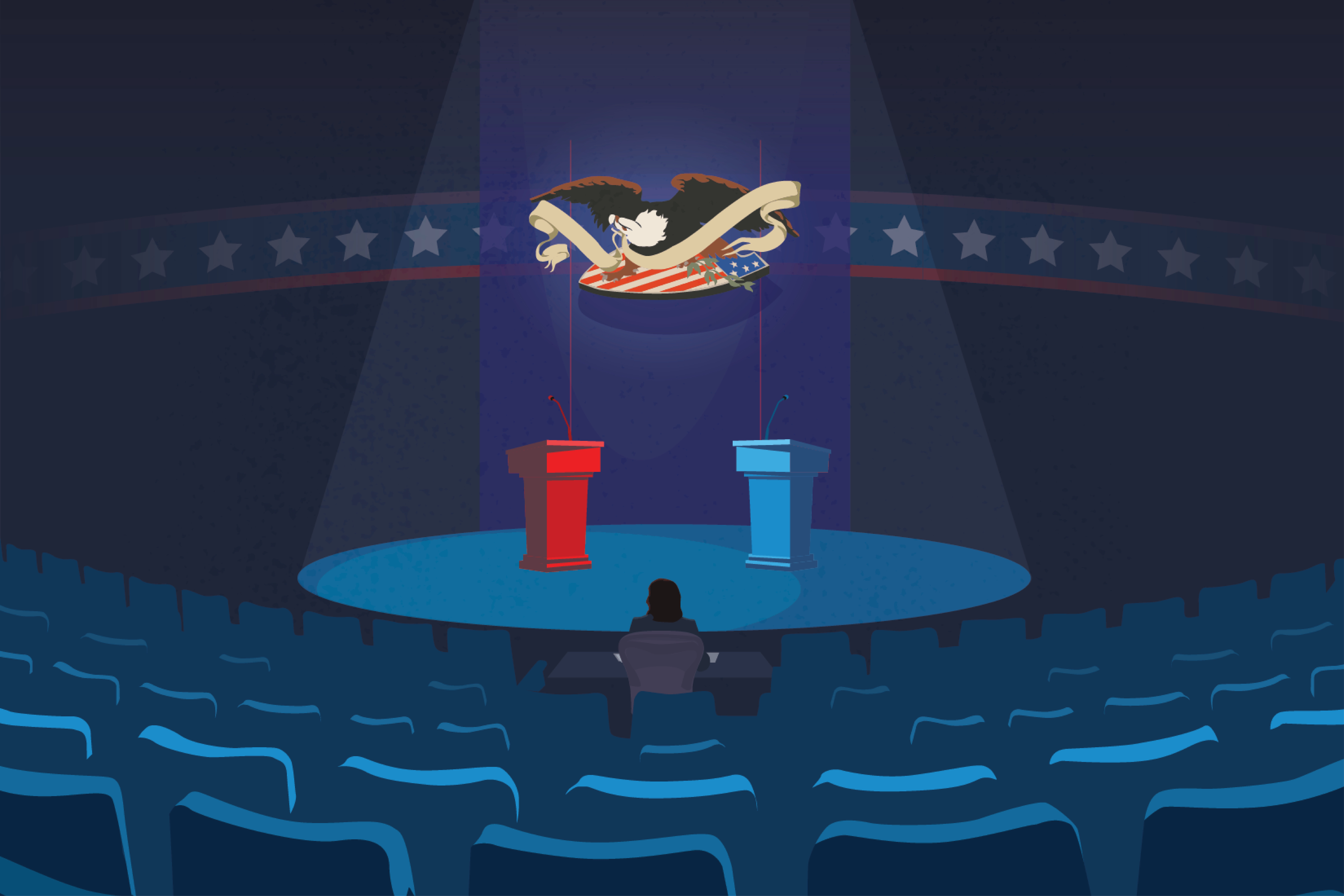
Daniel A. Cox, Ruy Teixeira June 29, 2023
The 2024 Presidential Election: Evolving Political Coalitions and Familiar Partisan Divisions
Ahead of the 2024 presidential election, the AEI Survey Center on American Life conducted a national survey of Americans that explored a wide range of political attitudes, current voting preferences, and perceptions of the political parties.
In the news
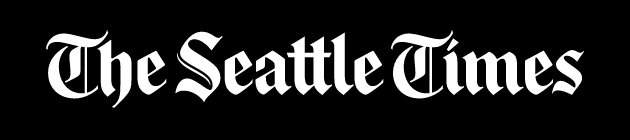
May 17, 2024
Parks Have Social Superpowers. Let’s Make More of Them
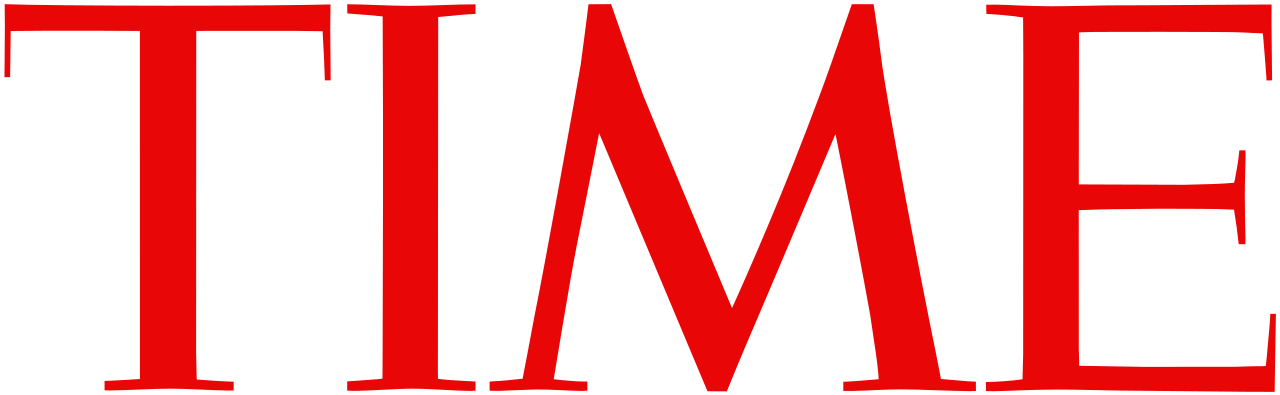
May 15, 2024
How Celibate Women Became a Threat
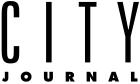
Gen Z’s Gender Stalemate

‘Tradwife’ Content Isn’t Really for Women. It’s for Men Who Want Submissive Wives.
- Open access
- Published: 17 January 2024
STEM learning opportunities and career aspirations: the interactive effect of students’ self-concept and perceptions of STEM professionals
- Yu Chen 1 ,
- Winnie Wing Mui So ORCID: orcid.org/0000-0002-9649-074X 2 , 3 ,
- Jinxin Zhu 4 &
- Stephen Wing Kai Chiu 5
International Journal of STEM Education volume 11 , Article number: 1 ( 2024 ) Cite this article
1 Citations
2 Altmetric
Metrics details
Students’ positive perceptions of scientists or engineers have been reported to be positively related to their science, technology, engineering, and mathematics (STEM) career aspirations. However, other research indicates that positive perceptions of experts in these fields might not necessarily lead to students’ pursuit of STEM careers. Self-concept, defined as one’s perceived abilities in specific academic domains, likely plays a moderating role in the relationship between perceptions and career aspirations according to the motivational theory of role modelling. Moreover, students’ perceptions of STEM professionals might be sourced from STEM-related media and school experiences. Therefore, through running a moderated mediation model, this study examined whether and how the influences of media consumption and school STEM learning opportunities on career aspirations would be mediated by perceptions of STEM professionals, and whether the mediation effect would be conditional on students’ self-concept.
Data were collected through an online survey of 608 primary and secondary school students from Hong Kong, and were analysed using structural equation modelling.
Results revealed that the students’ positive perceptions of STEM professionals were positively associated with their career aspirations, and mediated the links from media consumption and school opportunities to career aspirations. In addition, this mediated pathway depended on STEM self-concept, such that perceptions of STEM professionals were only linked with STEM career aspirations for adolescents with average or high levels of self-concept.
Conclusions
The findings of this study suggest the need to pay attention to the STEM perceptions and self-concept interaction while designing and implementing learning activities to connect a diversity of students with STEM careers. It is not only important to foster students’ self-concept, but also to enrich their knowledge of diverse occupations, so as to help diversify their perceptions that being professionals in these fields is desirable and attainable, and to eventually inspire more student engagement and participation in STEM.
Introduction
The critical role of science, technology, engineering, and mathematics (STEM) education in developing global competitiveness and prosperity has been emphasized in many regions across the world over the years. Yet, research indicates that despite multiple educational reforms and substantial resources, there are still low college enrolment rates in STEM fields and a lack interest in STEM careers (Kayan-Fadlelmula et al., 2022 ; Sahin et al., 2020 ; Vooren et al., 2022 ). A similar situation can be observed in Hong Kong. While the government and stakeholders have been stepping up efforts to promote STEM education in primary and secondary schools, there is no evidence that students have developed aspirations for STEM-related careers.
Students’ positive perceptions of STEM professionals are considered to have positive impacts on their career aspirations in the STEM fields (e.g.Chan et al., 2019 ; Scholes & Stahl, 2020 ). When students consider STEM professionals as intelligent, competent, innovative, or successful, they show respect and interest in STEM-related jobs and are likely to pursue these jobs (Chan et al., 2019 ). However, other studies have revealed that positive perceptions (e.g., scientists as smart people) may not necessarily contribute to some students’ STEM interests or aspirations (e.g.DeWitt et al., 2013 ; Jones & Hite, 2020 ; Kang et al., 2019 ). Evidence of the link between perceptions of STEM professionals and career aspirations remains inconclusive. Hence, research into the potential moderators of students’ perceptions of STEM professionals is still warranted (Gladstone & Cimpian, 2021 ).
Students’ self-concept may be one of the crucial moderators of the relationship between perceptions of STEM professionals and career aspirations. Researchers have defined self-concept as one’s beliefs about one’s personal abilities in specific academic domains (such as science or mathematics) (Bong & Skaalvik, 2003 ; Rüschenpöhler & Markic, 2019 ). The motivational theory of role modelling (Morgenroth et al., 2015 ), which was developed based on the expectancy-value theories of motivation, highlights the distinct functions of individuals’ perceptions of STEM professionals and self-concept in influencing students’ role modelling for career motivations. Specifically, students might be more inclined to pursue or select STEM careers if they perceive themselves as being capable in STEM-related subjects and able to achieve success in STEM fields like their STEM role models (match between self-concept and perceptions of STEM professionals) (Gladstone & Cimpian, 2021 ; Morgenroth et al., 2015 ; Starr & Leaper, 2019 ). By contrast, a mismatch between self-concept and perceptions of STEM professionals’ STEM abilities and success may lead students to perceive STEM professionals as unreachable or unattainable role models, resulting in decreased intentions or aspirations to pursue STEM careers. In other words, there may be an interaction between students’ perceptions of STEM professionals and STEM self-concept, or more specifically, STEM self-concept is likely to moderate the association between students’ perceptions of STEM professionals and their career choices or aspirations (Morgenroth et al., 2015 ). Not many studies have been conducted to examine this, except for one previous study by Starr and Leaper ( 2019 ). However, their study was limited to investigating the moderating role of self-concept in the association between STEM stereotypes and confidence and interest in STEM learning through using regression analyses, and only involved high school students in the United States. Our study extended their research by directly examining whether self-concept would play a moderating role in the relationship between positive perceptions and career aspirations with a sample of Hong Kong primary and secondary school students.
Moreover, while positive links from media consumption and school STEM experiences to career aspirations were found in several past studies (e.g.Archer et al., 2014 , 2015 ; Bottia et al., 2015 ; Dou et al., 2019 ; Gossen & Ivey, 2023 ; Halim et al., 2021 ; Reinhold et al., 2018 ; Tzu-Ling, 2019 ), other studies have indicated that increased STEM exposure failed to contribute to some students’ STEM interests or aspirations (e.g.Kang et al., 2019 ; Rezayat, 2020 ; Wieselmann et al., 2020 ). Hence, research is needed to include the examination of interactive effects (e.g., in this study, the interaction effect of perceptions of STEM professionals and self-concept on career aspirations) that can help reveal how and why these STEM experiences affected students differently (Cohen et al., 2021 ). Given the positive links between media consumption and school experiences with students’ perceptions of STEM professionals (Archer et al., 2014 ; Davis-Hall et al., 2023 ; Martin & Fisher-Ari, 2021 ; Halim et al., 2021 ; Martin & Moote et al., 2020 ; Starr, 2018 ), and the potential interaction of perceptions and self-concept as described above, it was hypothesized that self-concept could possibly moderate the mediating role of perceptions in the links from media consumption and school opportunities to STEM career aspirations (a moderated mediation model). To the best of our knowledge, the possible influence of the interaction between perceptions and self-concept on the relations between media consumption, school STEM opportunity, and career aspirations remains underexplored in the literature.
To address this gap, this study aimed to examine the interactive effects of perceptions of STEM professionals and self-concept on the relationships between media consumption, school opportunities, and STEM career aspirations among Hong Kong students. This study adds evidence to the existing literature on the underlying mechanisms of how these factors (media consumption, school opportunities, perceptions of STEM professionals, and self-concept) might jointly influence students’ STEM career aspirations. Knowledge of possible mediating or moderating effects is important for developing STEM experiences to foster students’ career aspirations with different characteristics (e.g., students with different levels of self-concept). Overall, there were two major research questions addressed in this study.
RQ1. Do students’ positive perceptions of STEM professionals mediate the relationships between media consumption, school opportunities, and their career aspirations in STEM?
RQ2. Does students’ STEM self-concept moderate such mediated relations?
Literature review
- STEM career aspirations
STEM career aspirations can be considered as a subset of career aspirations, defined as ‘an individual’s expressed career-related goals or choices’ (Rojewski, 2005 , p. 132), which contribute to future STEM-related career paths (Mau & Li, 2018 ). Hou and Leung ( 2011 ) explained that STEM career aspirations refer to the evolving goals, ideals, and intentions of individuals to aspire to careers in the STEM fields in the future. Aspirations for STEM careers can be understood as expressions of people's hopes or ambitions regarding further STEM studies and careers (Archer et al., 2020 ).
However, it has been a long-standing issue that our students lack interest in or aspirations to pursue careers in STEM (DeWitt et al., 2014 ; Du &Wong, 2019 ; Martin & Fisher-Ari, 2021 ). Students were found to hold persistent, low aspirations in science during the secondary school stage (Archer et al., 2020 ). Chinese students reported relatively low science career aspirations as well (Du & Wong, 2019 ). A number of factors such as learning experiences, perceptions of STEM professionals, motivational beliefs, gender, or parental educational levels and occupations have been identified as significant predictors of students’ career aspirations (Ketenci et al., 2020 ; Mau & Li, 2018 ; Mau et al., 2020 ; Rosenzweig & Chen, 2023 ; Tyler-Wood et al., 2018 ; Wang & Degol, 2017 ). For instance, students who did not show strong science ability beliefs, that is, did not strongly believe that they could do science and that they valued science, tended not to aspire to STEM careers (Aschbacher et al., 2014 ). Girls were found to be less likely to pursue an STEM-related career than boys (Ketenci et al., 2020 ; Tandrayen-Ragoobur & Gokulsing, 2021 ; Wang et al., 2023 ), and the attrition rates in STEM subjects were found to be higher for girls than for boys (Edwin et al., 2019 ), resulting in low participation of females in STEM professions. After controlling variables such as socio-economic status, school type, or school urbanicity, female students were two times less likely than male students to choose a career in the STEM fields. Particularly, girls were found to be heavily under-represented in careers in physical science, computer science, and engineering (Rosenzweig & Chen, 2023 ). Conlon et al. ( 2023 ) asked primary school students what job in the future they wanted, and reported similar results that boys showed higher aspirations to STEM careers than girls. Besides, students with non-college-educated parents were found to be significantly more likely to change from STEM to non-STEM career expectations by 11th grade (Starr et al., 2022 ). Hence, still more studies are needed to explore how to bolster or maintain students’ STEM career aspirations.
Students’ positive perceptions of STEM professionals and career aspirations
A number of studies have been conducted to investigate how students perceive STEM professionals (e.g., scientists, technicians, or engineers) and their work (Bian et al., 2017 ; Ferguson & Lezotte, 2020 ; Liu & Chiang, 2019 ; Luo & So, 2022 ; Martin & Fisher-Ari, 2021 ; So et al., 2020 ; Starr, 2018 ). Results have shown that students tend to see STEM professionals positively in terms of intelligence, competence, accuracy, or success (Chan et al., 2019 ; DeWitt et al., 2013 ), yet regard their appearance, personality, or sociability negatively (Garriott et al., 2017 ; Jones & Hite, 2020 ; Starr, 2018 ).
Students’ perceptions of scientists or engineers might be highly influential in the formation and actualization of their aspirations for STEM careers (So et al., 2020 ). Students’ positive views of innovation and entrepreneurship competencies might have positive and direct impacts on their STEM-related aptitudes, and their views of engineers’ career prestige could have positive and direct impacts on their career interest in engineering (Chan et al., 2019 ). However, negative stereotypes might decrease students’ confidence, interest, and goals regarding STEM (Garriott et al., 2017 ; Scholes & Stahl, 2020 ). A narrow understanding of scientists might exacerbate students’ inability to imagine themselves as scientists, considering science as something that is not ‘for me’, resulting in decreased desires for those careers in the future (Bøe et al., 2011 ). For instance, the perceived masculine nature and dangers of such work might increase students’ reluctance to become scientists (Scholes & Stahl, 2020 ). Through interviews with STEM undergraduates, it was found that for those who decided to leave the STEM pipeline, the reasons they mostly gave were that they perceived non-STEM jobs, especially those in finance and business, as more secure and accessible and having greater economic returns as compared to jobs in the STEM sector (Wong et al., 2022 ). Thus, promoting positive conceptions of STEM is important for increasing participation and diversity in STEM (Martin & Fisher-Ari, 2021 ).
While positive perceptions (or images) of scientists or engineers were often found to have positive impacts on STEM career aspirations, other studies have reported that there might be no relationship between the two (Kang et al., 2019 ; Mohtar et al., 2019 ), or sometimes a contrast effect might occur (DeWitt et al., 2013 ; Jones & Hite, 2020 ). For instance, students’ interest in physical sciences-based careers was found to not be influenced by their perceptions of those careers (Mohtar et al., 2019 ). It was also revealed that students’ perceptions of scientists’ work did not predict their interest in careers in biology science and physical science (Kang et al., 2019 ). Sometimes positive perceptions can be demotivating. Popular perceptions of scientists, such as ‘specialist’ and ‘clever’ might feed into students’ feelings that science is not for them, resulting in their negative science identity and low aspirations (DeWitt et al., 2013 ). When students saw high levels of STEM professionals’ competence might also be less attainable. Perceived high levels of STEM professionals’ competence or success (e.g., very competent, extremely successful) might decrease students’ motivation to pursue STEM careers, because students might feel that success is unattainable and therefore be demotivated (e.g., they can, but I cannot) (Gladstone & Cimpian, 2021 ). Jones and Hite ( 2020 ) analysed Korean students’ drawings of scientists in terms of science behaviours, science subjects, setting and appearance, skills and abilities, job satisfaction, scientist attributes, and job attributes. Their findings suggested that students might view scientists in a positive light, but as role models, students considered their jobs as being unattainable or undesirable, resulting in their rejection of the possibility of choosing science-related careers. Hence, research is still needed to address questions about why and for whom positive perceptions of STEM professionals as intelligent, and/or competent motivate their career aspiration development (Gladstone & Cimpian, 2021 ).
Interaction between self-concept and perceptions of STEM professionals
Self-concept is one of the important constructs of the expectancy-value theory (Wigfield & Eccles, 2000 ). It represents a key predictor of individuals’ motivation, emotion, and performance, and may directly influence achievement or career choices (Wigfield & Eccles, 2000 ). Self-concept is often defined as one’s perceived ability in a specific domain (e.g., science or mathematics) that is necessary to successfully perform a particular behaviour in that domain (Bong & Skaalvik, 2003 ). Thus, applied to STEM-related domains, if students believe that they can do well in learning mathematics, science, or information technology (IT), they are referred to as having a higher self-concept in those domains.
Self-concept in STEM-related domains has been shown to be related to students’ aspirations in those fields (e.g.Kang et al., 2021 ; Rosenzweig & Chen, 2023 ; Rüschenpöhler & Markic, 2019 ; Sax et al., 2015 ; Wang et al., 2017 ). For instance, students with a high science self-concept were likely to show a stronger intention to pursue science-related careers than those with a low science self-concept (Kang et al., 2021 ). In addition, students were more likely to pursue an STEM-related career if they perceived them as having high abilities in learning mathematics (Ketenci et al., 2020 ). Having a greater ability self-concept in math helped increase the likelihood of future STEM employment among students (Wang et al., 2017 ). By contrast, inadequate self-concept might lead students to consider not entering the STEM fields. For instance, there might be a link between female students’ stereotypes that women do not have natural STEM abilities and women’s under-representation in STEM careers (Clark et al., 2021 ). For some students who considered computer science or engineering as their least preferred careers, they frequently chose competence-related beliefs (e.g., by not thinking that they would do well at the career) as one main category of their reasons (Rosenzweig & Chen, 2023 ).
Self-concept was likely to moderate the association between perceptions of STEM professionals and aspirations. According to the motivation theory of role modelling, this is because students tend to evaluate how well their self-concept matches the attributes of the possible role models when they are deciding whether to pursue a particular career (Morgenroth et al., 2015 ). When students perceive a mismatch between their own abilities and those of role models (e.g., scientists or engineers), they may be demotivated to follow their role models (Morgenroth et al., 2015 ). As a result, perceptions of STEM professionals may not positively impact the development or maintenance of career aspirations for students with relatively low levels of self-concept. Starr and Leaper ( 2019 ) found that the negative relation between students’ endorsing stereotypes and motivation was only significant among those with low genius self-concept, suggesting the moderating role of self-concept. However, their study was limited to investigate the relationships between self-concept and stereotypes with students’ STEM learning. The potential moderating role of self-concept in the relationship between positive perceptions of STEM professionals and career aspirations remained unknown, which was tested in this study.
Roles of perceptions and self-concept in the relations between media consumption, school STEM experiences, and career aspirations
Increasing students’ STEM opportunities and experiences may foster their STEM interest and engagement, and contribute to increased STEM demands (Bottia et al., 2015 ; Cohen et al., 2021 ; Dou et al., 2019 ; Gossen & Ivey, 2023 ; Halim et al., 2021 ; Reinhold et al., 2018 ; Tzu-Ling, 2019 ). In addition to school experiences, Hong Kong students were found to have more media-related experiences (e.g., reading books/magazines) than other out-of-school experiences (e.g., visiting museums) (Chen et al., 2022 ). By considering the context of Hong Kong, this study placed a specific focus on investigating the links from media consumption and school experiences to career aspirations, and the potential role of perceptions of STEM professionals and self-concepts in mediating or moderating these links.
Prior research has revealed positive connections between students’ career aspirations and the instructional quantity and quality of school science education (Wang & Staver, 2001 ). Science-related classroom experiences might largely affect students’ views of their career path (Gossen & Ivey, 2023 ) and their decisions regarding whether or not to pursue STEM careers (Banerjee et al., 2018 ; Šorgo et al., 2018 ). More specifically, students' primary school science experiences seem to be a significant positive predictor of their career pursuits, indicating the importance of schooling (Cohen et al., 2021 ). Experiences of STEM extracurricular activities seem to boost students’ confidence and benefit their STEM career intentions and actions (Gossen & Ivey, 2023 ). STEM-related media consumption, as an important form of capital, is also considered to play an essential role in strengthening students’ STEM trajectories (Archer et al., 2014 ; Moote et al., 2020 ). The extent to which students consume STEM-related media, such as via TV programs, books/magazines, and online, appears to largely affect their STEM aspirations (Archer et al., 2015 ; Halim et al., 2021 ). There might be influences on career choice from popular science television channels and programmes (Cerinsek et al., 2013 ). After controlling for home environment, gender, and other relevant factors, consuming science and science-fiction media (e.g., books and television) was found to still be predictive of students’ STEM identity, which might show strong influences on their future career choice and persistence (Dou et al., 2019 ).
However, other research found that students’ experiences with science in the classroom did not directly predict their choices of STEM majors (Steenbergen-Hu & Olszewski-Kubilius, 2017 ), or their identification with STEM-related careers (Kang et al., 2019 ). Rather, early experiences might have negative impacts on career choices when students encounter some difficulties in learning science (Rezayat, 2020 ). School-based instruction may sometimes decrease students’ STEM interest and career choices due to constraints, such as time, standardized test pressures, or lack of teaching and learning resources (Rezayat, 2020 ; Wieselmann et al., 2020 ). Likewise, media influences might not guarantee students’ choice of STEM careers (e.g.Mohtar et al., 2019 ; Steinke et al., 2021 ). STEM media (e.g., STEM TV; STEM videos) were found to not directly impact students’ interest in STEM careers (Chen et al., 2023 ). Students’ preferences for STEM professionals featured in media might affect the influence of media on their career decisions to some extent by affecting their self-identification with STEM (Steinke et al., 2021 ). The findings of these studies imply that not all students seem to benefit from STEM-related media consumption or school opportunities.
In other studies, school and media were found to play important roles in shaping how students perceive STEM professionals (Davis-Hall et al., 2023 ; Jones & Hite, 2020 ). For instance, many STEM educational programmes or interventions were purposefully designed to feature experts in various STEM fields to give students a more accurate understanding of the attributes of scientists or engineers, and to improve their intentions to aspire to STEM careers (Davis-Hall et al., 2023 ; Emembolu et al., 2020 ; Farland-Smith, 2010 ; Gladstone & Cimpian, 2021 ; Shimwell et al., 2023 ; Wyss et al., 2012 ). Researchers have found that involving STEM-speaking adults who introduced their jobs and the use of STEM during robotics-based interventions had a positive impact on primary school students’ interest in learning STEM and their positive attitudes toward STEM careers (Hudson et al., 2020 ). As for media influences, televised scientist characters might affect young learners’ views of science (Whitelegg et al., 2013 ). Students who were exposed to accurate images of scientists through school (e.g., textbooks) or media resources (e.g., magazines, museums, and TV) were found to be more likely to have non-stereotyped images of scientists (Tan et al., 2017 ). Media images of STEM professionals may be used as important information for students when they consider future personal and professional identities during their adolescence (Steinke, 2017 ).
Hence, students’ perceptions of STEM professionals might potentially mediate the influence of school and media-related experiences on their career aspirations. School or media experiences might be more or less effective in partially increasing students’ career aspirations, depending on whether these experiences help develop students’ positive perceptions. Moreover, given the potential interplay between perceptions and self-concept, it is possible that the impacts of school or media experiences on career aspirations might vary among students with differing levels of STEM self-concept. When students are presented with successful, competent images of STEM professionals in diverse STEM activities, those with higher self-concept might be able to perceive the possibility of STEM careers as attainable, whereas those with lower self-concept might be demotivated by seeing STEM careers as too difficult to achieve. In short, it was hypothesized that these experiences might be more motivating for students with higher self-concept, but less motivating for those with lower self-concept to develop or maintain aspirations to STEM careers. How the interactions between perceptions and self-concept might contribute to the varying relations between school and media experiences and career aspirations was directly examined in this study.
The hypothesized model of this study
This study drew upon the motivational theory of role modelling (Morgenroth et al., 2015 ), with the aim of investigating the complex relationships among media consumption, school STEM opportunities, positive perceptions of STEM professionals, self-concept and students’ STEM career aspiration development. Previous findings have indicated that career aspirations might be significantly influenced by factors, such as gender, grade level, and parents' occupation and education level (e.g.Domenico & Jones, 2006 ; Reinhold et al., 2018 ; Sáinz & Müller, 2018 ; Starr et al., 2022 ; Wang et al., 2023 ). Given these findings, gender, school level, parental educational level, and parental STEM-related jobs were also included. The hypothesized moderated mediation model is presented in Fig. 1 . Specifically, the study was mainly concerned with the following three hypotheses:
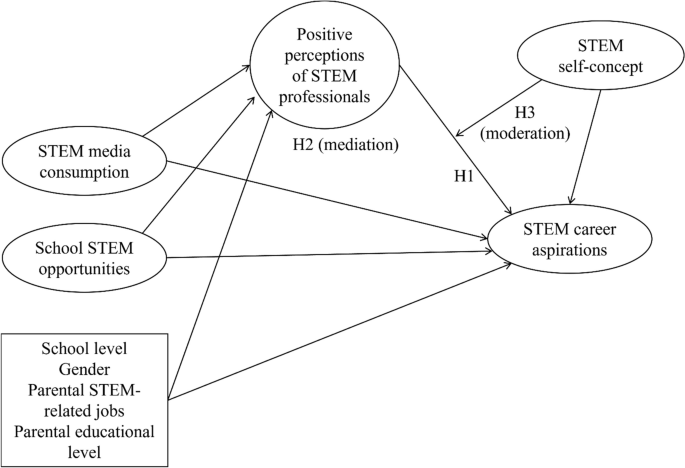
Hypothesized moderated mediation model
H1. Positive perceptions of STEM professionals are positively and significantly associated with STEM career aspirations. (To address RQ1).
H2. STEM-related media consumption and school opportunities are positively associated with positive perceptions of STEM professionals, and subsequently with career aspirations. (To address RQ1).
H3. STEM self-concept significantly moderates the direct effect of positive perceptions of STEM professionals, and the indirect effects of media consumption and school opportunities on career aspirations. (To address RQ2).
Participants
A total of 608 students from three primary schools, two junior secondary schools, and one senior secondary school in Hong Kong were involved in this study, of whom 353 were girls and 255 were boys. As for school levels, 56.6% ( n = 344) were upper primary students from Grade 4 to Grade 6, with ages ranging from 11 to 13 (years), and an average age of 11.63 (0.78); 31.9% ( n = 194) were junior secondary students from Grade 7 to Grade 9, with ages ranging from 15 to 17 and an average age of 15.25 (0.53); and 11.5% ( n = 70) were senior secondary students from Grade 10 to Grade 12, with ages ranging from 17 to 19, and an average age of 17.10 (1.024). They were all ethnic Chinese, and around 95% of the students were born in Hong Kong. Student participation was on a voluntary basis. Before participating in this study, consent forms were sent to the students, their teachers, and schools to obtain their permission. Questionnaires were anonymous and confidential.
Data collection
Data were collected through an online survey with the help of teachers in participating schools. A questionnaire was created for this online survey, and consisted of five main scales subjected to statistical analysis, namely, (1) STEM career aspirations, (2) STEM self-concept, (3) positive perceptions of STEM professionals, (4) STEM-related media consumption, and (5) school STEM-related opportunity. Overall, there were 27 items for the five scales (see Table 3 ). These items were mostly adopted and modified from the instrument developed and validated by Archer and colleagues (Archer & DeWitt, 2016 ; DeWitt & Archer, 2015 ; DeWitt et al., 2011 ) to investigate young learners’ science aspirations. The validated instruments of Chan et al. ( 2019 ) and Starr ( 2018 ) which measured students’ perceptions of engineers and scientists, respectively, were also taken into consideration when selecting items for understanding students’ positive perceptions of STEM professionals. Demographic information such as gender (girls coded as 0 and boys coded as 1), school level (primary school as 0, junior secondary as 1, senior secondary as 2; dummy coded with primary school as the reference group in the analysis), parental STEM-related jobs (parents having STEM jobs as 1 and the others as 0), and parental educational level (bachelor or above as 1 and the others as 0) was also collected. Questions were presented to students in plain Chinese language to help them understand the meaning. More details of the five scales are presented below.
Scale 1: STEM career aspirations (career aspirations)
In this scale, students were asked about their intentions to engage in STEM careers in the future. There was one item used by Archer and Dewitt ( 2016 ) to measure students’ science career aspirations. This study adopted this item and added additional items to assess students’ career aspirations for a wide range of STEM areas, not merely the science area. Overall, there were four items using a 4-point Likert scale from 1 ( strongly disagree ) to 4 ( strongly agree ). Each item measured students’ career aspirations for one STEM field. Some common job examples of each STEM area were provided to facilitate students' responses. Sample items are: ‘When I grow up, I would like to work in mathematics (e.g., actuarial, accounting, insurance)’ and ‘When I grow up, I would like to work in engineering (e.g., civil engineering, automotive engineering, or architectural design)’. The reliability and validity of these items had been confirmed in a previous study by Chen et al. ( 2022 ) with another group of Hong Kong students. Higher scores refer to stronger STEM career aspirations among the students.
Scale 2: positive perceptions of STEM professionals (perceptions)
Different instruments have been developed for assessing students’ perceptions of scientists (Archer & Dewitt, 2016 ), engineers (Chan et al., 2019 ) or more generally, people who work in STEM (Starr, 2018 ). For instance, Archer and Dewitt ( 2016 ) used items such as ‘can make a difference in the world’, ‘make a lot of money’, ‘smart’, or ‘are respected by others’ to measure students’ views of scientists. The study by Starr ( 2018 ) used items, such as ‘gifted in math’, ‘naturally very intelligent’, or ‘geniuses’ to understand students’ perceptions of STEM people. In the study by Chan et al. ( 2019 ), students’ perceptions of engineers in terms of STEM-related aptitude and career prestige were measured with items, such as ‘good at maths and science’, ‘enjoys their job’, ‘well paid’, or ‘well-respected’. It could be found that there were some overlaps in the use of items for understanding students’ views of scientists or engineers.
Items that assessed positive views of STEM professionals and were more commonly included in these instruments (Archer & Dewitt, 2016 ; Chan et al., 2019 ; Starr, 2018 ) were adopted, because this study intended to measure students’ positive perceptions of a diversity of STEM people at a more general level. Overall, eight 4-point Likert items (1 = strongly disagree to 4 = strongly agree ) were selected and utilized to understand how students perceived STEM professionals, particularly their STEM-related abilities, and the contribution and success of their work. Sample items are: good at math, good at science, have an exciting job, make a difference in the world, high income, or respected by others. Higher scores refer to more positive perceptions of STEM professionals expressed by the students.
Scale 3: STEM self-concept (self-concept)
The students’ STEM self-concept was measured with six items using a 4-point Likert scale from 1 ( strongly disagree ) to 4 ( strongly agree ). The students were asked to self-evaluate whether they were confident in their personal abilities to learn science, mathematics, and technology at school. Because engineering-related subjects are not included in most Hong Kong schools (Zhang et al., 2022 ), measures of students’ self-concept in learning engineering-related subjects were not included in this study. Sample items are ‘I do well in Math subjects’ and ‘I understand most of the things in my Science subjects’. Higher scores refer to stronger STEM self-concept among the students.
Scale 4: STEM-related media consumption (media consumption)
Past studies (e.g., Jones & Hite, 2021; Tan et al., 2017 ) have identified books or magazines, televisions, and/or websites as the main media sources of students’ perceptions of scientists or career aspirations. Hence, in this scale, students were asked to report how often they obtained STEM information from these media resources (e.g., books, magazines, TV, or websites). This scale included one guiding question ‘How often do you obtain STEM information via the following media?’ and the four items indicated the four media resources of Books, Magazines, TV, and Websites (e.g., Google). All four items were designed on a 5-point Likert scale from 0 (never), 1 ( about once a year ), 2 ( about once a half year ), 3 ( about once a month ), and 4 ( about once a week ).
Scale 5: school STEM opportunities (school opportunities)
In Hong Kong, STEM education emphasizes the integration and application of the four fields, and aims at preparing students to face the needs of the rapid technological and scientific developments in society (Educational Bureau [EDB], 2016 ). STEM education was recommended to be integrated into regular Science, Mathematics, Information Technology (IT), or other school subjects (EDB, 2016 ). In addition, many schools organize STEM activities as short-term and extracurricular activities during out-of-class time (Tam et al., 2020 ; Zhang et al., 2022 ).
Hence, in this scale, students were asked to report whether they had opportunities for integrated STEM activities in regular Science, Mathematics, IT or other subjects, and/or out-of-class time inside school. There were five items using a 4-point Likert scale from 1 ( strongly disagree ) to 4 ( strongly agree ). Sample items are ‘My school provides STEM opportunities in Science lessons’, ‘My school provides STEM opportunities in IT lessons’, and ‘My school provides STEM opportunities out of class time’. Higher scores refer to more adequate school STEM opportunities as reported by the students.
Data analysis
A confirmatory factor analysis (CFA) was used to test and confirm the fit of the measurement model for our own data set using the Mplus 8.0 software. Goodness-of-fit was evaluated with the following indices: χ 2 , Root Mean Square Error of Approximation (RMSEA) (cutoff value of the fit < 0.08), Comparative Fit Index (CFI) (adequate value > 0.90), Tucker–Lewis index (TLI) (adequate value > 0.90), and Standardized Root Mean Square Residual (SRMR) (cutoff value of the fit < 0.08) (Cangur & Ercan, 2015 ; Hu & Bentler, 1999 ). Reliability was tested via SPSS as well to ensure the internal consistency of these scales. After that, descriptive statistics (i.e., means and standard deviations) and correlations among the variables, including school STEM opportunity, media consumption, perceptions of STEM professionals, self-concept, and career aspirations, were computed. In addition, t tests and one-way ANOVAs were run to detect whether there were significant differences in these variables due to gender or school level, respectively.
To test the hypothesized moderated mediation model (see Fig. 1 ), structural equation modelling (SEM) via Mplus 8.0 was employed. Through the SEM analysis (Stride et al., 2015 ), the mediating role of students’ perceptions of STEM professionals and the moderating role of STEM self-concept in the associations between school opportunity, media consumption, and career aspirations were tested. To ensure that the hypothesized model in this study was acceptable and meaningful, three models (as shown in Appendix A) were tested.
First, a reference model (Model A) and the hypothesized model (Model B) were tested to ensure the model fit of the hypothesized model. In the reference model (Model A), there were five latent variables (STEM opportunity, media consumption, perceptions of STEM professionals, self-concept, and career aspirations) and four categorical variables (gender, school level, parental educational level, and parental STEM-related jobs). In the hypothesized SEM model (Model B), one latent interaction term (perceptions of professionals × self-concept) was added on the basis of the reference model. Self-concept was specified as the moderator. A latent variable interaction approach was used to create the latent interaction term (perceptions of professionals × self-concept) to examine the interaction between perceptions of professionals and self-concept, which were latent variables.
In Mplus, to test latent interactions, random estimation and numerical integration are needed through the use of ‘type = random’ and ‘algorithm = integration’ commands (Wang & Wang, 2012 ). When numerical integration is carried out, Mplus does not provide model fit indices, such as CFI or TLI, but provides Akaike (AIC) and Bayesian (BIC) only. Hence, a comparison of the indices of AIC and BIC between the reference model (Model A) and the hypothesized model (Model B) was conducted. The model with the smaller AIC or BIC indicates a better model fit. Before running these models, all measured items were standardized. Hence, unstandardized results are reported in this study. Non-significant paths were excluded from the finalized model. For instance, parental educational level was found to have non-significant paths to perceptions and career aspirations, and thus was excluded. For a more specific test of the interactive effect between perceptions of STEM professionals and self-concept, simple slope analyses were performed.
According to the t test and ANOVA results shown below (Table 3 and 4 ), significant differences by gender and school level were found in school STEM opportunities and/or media consumption. Gladstone and Cimpian ( 2021 ) also indicated that the impacts of students’ perceptions of STEM professionals might vary due to gender or age. It was considered that gender or school level might affect the hypothesized model. In view of this, one additional model (Model C) with gender and school level effects controlled was tested to further test the correctness of the hypothesized model. In Model C, four paths from school level to school STEM opportunity and media consumption, and one path from gender to media consumption were added on the basis of the hypothesized model. Three additional interactive latent variables (gender_x_perceptions of professionals, and school level (junior secondary)_x_perceptions of professionals, and school level (senior secondary)_x_perceptions of professionals) were also added to examine whether the moderating effects of self-concept would change with gender or school level moderation taken into consideration.
Preliminary analyses
A confirmatory factor analysis was performed to evaluate the structural validity of the scales used in this study. The CFA model included five latent variables (school opportunities, media consumption, self-concept, perceptions of professionals, and career aspirations). Results showed that the factor loadings of most items were greater than 0.5 (see Table 1 ). Model fit indices were: χ 2 = 758.485, df = 307, RMSEA = 0.049, CFI = 0.936, TLI = 0.927, SRMR = 0.049. Cronbach’s alpha was greater than 0.8. Thus, these scales demonstrated good structural validity and inter-consistency reliability.
The means, standard deviations, and correlation coefficients are presented in Table 2 . The participating students’ average STEM self-concept was 2.86 out of 4, and their average aspiration for STEM careers was 2.33 out of 4, indicating that a considerable percentage of these students might lack adequate self-concept in learning mathematics, science, or IT, and might not show strong aspirations for careers in those STEM fields. Regarding students’ perceptions of STEM professionals, the average score was 3.06 out of 4, suggesting that the students tended to perceive experts in those fields as having adequate STEM capabilities, enjoying their jobs, having high incomes, and/or being respected by others. As for STEM media consumption and school opportunities, the mean scores were 2.41 and 3.09 out of 4, respectively. The results suggested that the students sometimes obtained information about science, technology, engineering, and mathematics via mass media, and most had STEM learning opportunities in school. All variables were positively associated with each other (see Table 2 ).
In addition, t tests were performed to examine whether there were significant gender differences in these variables. As shown in Table 3 , significant gender differences were found in self-concept, career aspirations, and media consumption. Boys were likely to show significantly stronger self-concept and career aspirations and to obtain more media resources related to STEM than girls. For perceptions of STEM professionals and school experiences, no significant gender differences were found. This means that boys and girls did not differ in their views about people in those areas in terms of their science or mathematics abilities or career prestige. Nor did they differ in their views about the opportunities provided in their schools.
ANOVAs were performed to test differences by school level (senior primary vs. junior secondary vs. senior secondary) on these variables (see Table 4 ). Significant differences by school level were found in self-concept, media consumption, and school opportunities. For multiple comparisons, it was found that secondary school students’ self-concept was significantly lower than that of primary school students. In addition, they reported significantly less media consumption or school opportunities compared to their younger peers. No statistically significant differences by school level were found in career aspirations or perceptions of STEM professionals.
The moderated mediation model of STEM career aspirations
In this study, three models (the reference model, the hypothesized model, and the model with gender and grade level further controlled) were performed and compared to ensure that the hypothesized model (Fig. 1 ) had a good model fit and so was more acceptable. Model fit indices of each model and the unstandardized coefficients of the paths included in each model are listed in Appendix A.
The reference model (Model A) and the hypothesized model (Model B) were first tested and compared to ensure the model fit of the hypothesized model. After excluding non-significant paths, it was found that the reference model fitted the data well (RMSEA = 0.042, CFI = 0.927, TLI = 0.919, SRMR = 0.060). There were no big differences found in AIC or BIC between these two models, indicating that the hypothesized model was likely to have a good model fit as well. After that, an additional model (Model C) with gender and grade level effects further controlled based on the hypothesized model (Model B) was performed. It was found that Model C had larger AIC and BIC. There were no significant moderating effects of gender or school level on the relation between perceptions and career aspirations. While significant paths from school level to media consumption or school opportunities were found, the inclusion of these paths seemed not to strongly affect those paths which were also shown in the hypothesized model (Model B). Looking at the variance explained, the hypothesized model (Model B) accounted for 20.3% of the variance in STEM career aspirations, whereas the other two models explained relatively less, with 18.0% and 18.4%, respectively. In other words, the hypothesized model (Model B) showed an acceptable model fit and helped explain relatively more variance in STEM career aspirations than Model A and Model C. Hence, the hypothesized model with unstandardized coefficients (see Fig. 2 ) was finally accepted in this study.
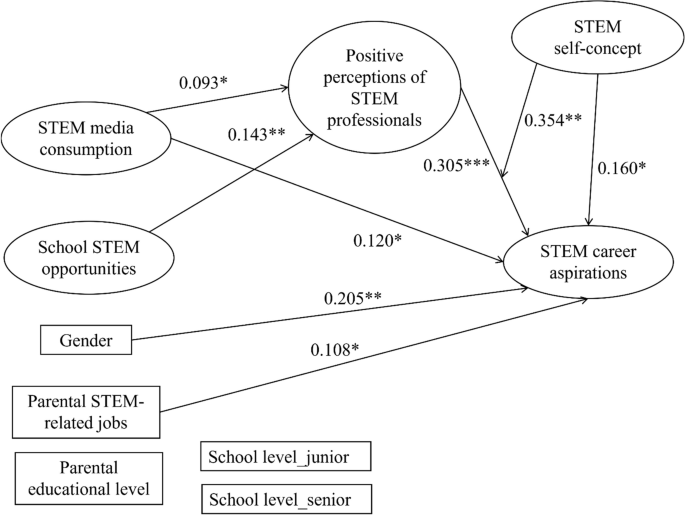
Unstandardized model of media consumption, school STEM opportunities, positive perceptions of STEM professionals, self-concept and career aspirations in STEM. Non-significant paths were excluded from the finalized model
The mediating role of students’ positive perceptions of STEM professionals
According to Fig. 2 , students’ perceptions of STEM professionals were significantly and positively associated with career aspirations ( β = 0.305, p = 0.000 < 0.001). H1 was supported. STEM media consumption was significantly and positively associated with career aspirations as well ( β = 0.120, p = 0.012 < 0.05). In addition, media consumption was found to be significantly and positively linked to students’ perceptions ( γ = 0.093, p = 0.037 < 0.05), which were subsequently linked to career aspirations. That is, media consumption was likely to influence students’ career aspirations in STEM careers positively and directly, but also positively and indirectly through enriching students’ perceptions of scientists or engineers.
School STEM opportunities were found to have no significant direct path to career aspirations ( β = 0.034, p = 0.432). However, the indirect path from school opportunities to career aspirations via perceptions of professionals was found to be statistically significant (0.042, p = 0.013 < 0.05). That is, perceptions of STEM professionals significantly mediated the relation between school opportunities and career aspirations. In other words, school opportunities might be more beneficial for students’ career aspiration development if these opportunities concurrently helped develop students’ positive views of STEM professionals. Besides, self-concept was found to be significantly and positively associated with career aspirations ( β = 0.160, p = 0.033 < 0.05). Hence, H2 was partially supported. Regarding parent-related factors, only the association between parental STEM-related jobs and career aspirations was found to be significant ( β = 0.108, p = 0.043 < 0.05).
The moderating role of STEM self-concept
In terms of the moderation effect, the results showed that the interaction (perceptions of professionals × self-concept) was significantly related to aspirations ( β = 0.354, p = 0.004 < 0.01). STEM self-concept positively moderated the impacts of perceptions of STEM professionals on career aspirations. The link between perceptions and career aspirations was much stronger for students with high STEM self-concept ( β = 0.659, p = 0.000 < 0.001), than for students with mid ( γ = 0.305, p = 0.000 < 0.001) or low ( β = − 0.049, p = 0.707) self-concept (see Fig. 3 ).
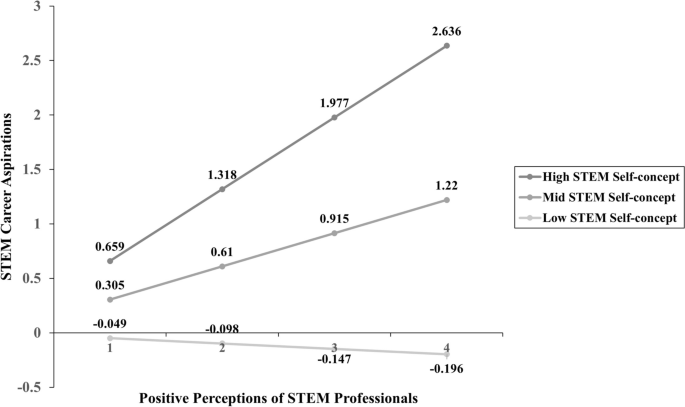
Moderated impacts of positive perceptions of STEM professionals on career aspirations by self-concept
The SEM model also demonstrated that the indirect effect of school opportunities and media consumption on aspirations via perceptions of STEM professionals was conditional, depending on self-concept. The conditional indirect effect of school opportunities and media consumption on aspirations via perceptions through adjustment of self-concept is indicated by this formula: (a1 + a2) (b1 + b2 self-concept) (Stride et al., 2015 ). Specifically, the effect of school opportunities and media consumption on perceptions was a1 and a2, the effect of perceptions on aspirations was b1, and the effect of interaction item (perceptions × self-concept) on aspirations was b2. When self-concept took the value of -1SD, 0, and 1SD, the overall conditional indirect effect of school opportunities and media consumption was -0.011 ( p = 0.721), 0.072 ( p = 0.003 < 0.01), and 0.155 ( p = 0.001 < 0.01), respectively. That is, the impacts of school opportunities and media consumption on aspirations through perceptions of STEM professionals was likely to increase as students’ self-concept increased (see Fig. 4 ). Yet, if students had low self-concept, even though they were provided with more STEM opportunities inside school or more STEM-related media resources to develop understandings of scientists or engineers, their STEM career aspirations might not be effectively enhanced. Hence, H3 was supported.
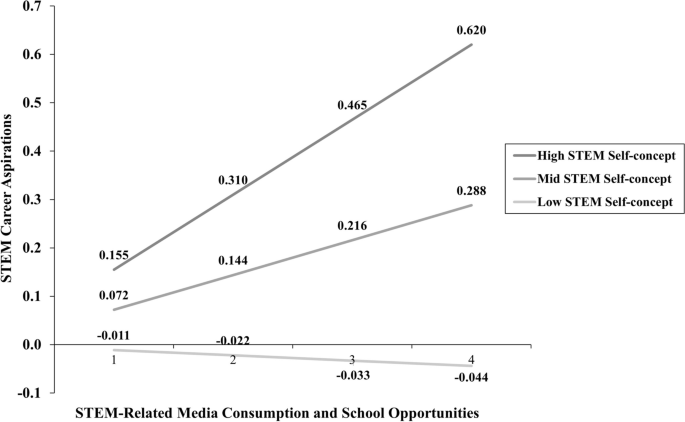
Moderated indirect effects of school opportunities, and media consumption on STEM career aspirations via positive perceptions of STEM professionals by self-concept
This study contributes new insights to help understand the complex roles of perceptions of STEM professionals and self-concept in the connections of media and school experiences with STEM career aspirations among Hong Kong students. It was found that perceptions of STEM professionals were significantly and positively associated with students’ career aspirations, and concurrently, it significantly mediated the relationships between media consumption and school STEM opportunities with career aspirations. Self-concept moderated the direct path from perceptions of STEM professionals to aspirations, and the indirect paths from media and school STEM experiences to career aspirations via perceptions. It is worth noting that the strength of the associations between STEM experiences, perceptions of STEM professionals, and career aspirations was more substantial among students with high (vs. mid or vs. low) STEM self-concept. Below, the implications of these findings are further discussed.
Media consumption, school opportunities and STEM career aspirations
The findings revealed that a considerable percentage of the students might lack adequate aspirations for STEM careers (Mean score = 2.33 out of 4). This may be related to the limited career prospects of STEM in Hong Kong (Legislative Council Secretariat, 2020 ; Tsui et al., 2019a ). It was also demonstrated that there was a significant gender difference in career aspirations (see Table 3 ). Compared to girls, boys reported significantly higher career aspirations. For the impact of school level, no significant differences were found in aspirations, suggesting that Hong Kong students’ aspirations in STEM seemed stable across school levels (see Table 4 ). This finding is consistent with those of previous studies (e.g.Archer et al., 2013 , 2020 ; Sadler et al., 2012 ) which also found persistent and low aspirations among students during their secondary school years. One explanation for this would be that the proportion of young learners specifically aspiring to STEM careers might be established fairly early and then remain stable as they grow up (Archer et al., 2020 ). It is therefore important for students to form their career aspirations in the STEM fields by age 14, or before senior secondary school (Kang et al., 2021 ; Sadler et al., 2012 ). Aligned with these prior studies, this study emphasizes the need to cultivate Hong Kong students’ science aspirations in their early learning stages.
STEM media consumption was found to be significantly and positively related to career aspirations. Students with more access to STEM media were likely to show higher aspirations for such careers. This result is consistent with those of previous studies (Chen et al., 2022 ; Dou et al., 2019 ; Halim et al., 2021 ) and supports Archer et al. and and’s ( 2014 , 2015 ) studies which emphasized the importance of media resources as one dimension of STEM capital for shaping students’ career aspirations.
However, no positive link was found between school STEM opportunities and career aspirations. This result aligns with previous research (e.g.Kang et al., 2019 ; Rezayat, 2020 ; Steenbergen-Hu & Olszewski-Kubilius, 2017 ) that indicated the absence of a direct link between school experiences and students’ attitudes toward STEM, but was inconsistent with others (Banerjee et al., 2018 ; Cohen et al., 2021 ). One reason may be that the scale used in this study mainly investigated students’ STEM opportunities in their regular mathematics, science, technology, or other subject learning in school. Hence, the primary purpose of these STEM opportunities might be to enhance students’ science, mathematics, or technology knowledge or skills that should be learned in these school subjects, rather than encouraging them to explore their possible directions for future careers. This might be due to the fact that in Hong Kong, academic performance is imperative in determining a promising future (Tsui et al. 2019b ). Moreover, there was a concern about the limitations of instructional time and personal abilities of integrating STEM by teachers who are involved in STEM education (Chen & So, 2022 ). As a result, the integration of STEM into regular school subjects might still be relatively inadequate, and therefore its effectiveness is limited. Another explanation is that the variable of school STEM-related opportunities might lack variability across students at the individual level within a single school context, because the participating students were nested within schools in this study. However, due to the small sample size at the school level (six schools involved), this study did not further examine the influence of school opportunities on students’ career aspirations at the school level. Future studies may consider addressing this by including more schools.
Students’ positive perceptions of STEM professionals and their impacts
Previous research has indicated that helping students develop positive views of scientists or engineers might effectively increase their likelihood of choosing STEM careers (e.g.Chan et al., 2019 ; DeWitt et al., 2014 ). In this study, a significant and positive link between perceptions of STEM professionals in terms of STEM abilities or career success and career aspirations was identified with the students who reported mid or high self-concept. That is, among students with mid or high self-concept, holding more positive perceptions of STEM professionals’ competence and career prestige might better motivate them to aspire to careers in STEM. According to Tsui et al. ( 2019b ), when Hong Kong students make choices about their future study and work, they place a high priority on personal public examination results, but also the stability and prospects that are offered by jobs. Therefore, for Hong Kong students with adequate self-concept in STEM, information on job satisfaction and prospects related to STEM should be disseminated to them so as to foster their positive understanding of STEM people, and so develop stronger career intentions. When they are able to perceive STEM professions as being attainable, enjoyable, and having prospects, they may be more motivated to choose STEM careers.
For most students, greater access to STEM-related media was likely to help them develop more positive perceptions of scientists or engineers. In this study, a positive path was found between media consumption and perceptions. This might be due to the fact that STEM information from media is an important source for students to develop images or perceptions of scientists or engineers (Jones & Hite, 2020 ; Steike, 2017 ; Tan et al., 2017 ). The portrayal of science, technology, or engineering in mass media as sometimes powerful, and generating hope for the future seems to promote a competitive schema related to the promise of science (Nisbet et al., 2002 ). On the other hand, school STEM opportunities were found to have a significant and positive path to perceptions. Comparing the two paths, school opportunities appeared to be potentially more relevant in shaping perceptions of STEM professionals than media experiences. There might be more direct and close exposure opportunities to STEM professionals provided in school, such as involving STEM experts to serve as role models and mentors, to arrange hands-on activities, to introduce students to career possibilities, and to boost students’ STEM participation (Gamse et al., 2017 ). Moreover, with the support of school teachers, it might be easier for students to alter their negative or stereotypical views of scientists or engineers, and this may in turn contribute to their positive attitudes toward or perceptions of STEM careers. For instance, helping students to see the connections between their school learning and STEM-related jobs might enable them to develop more positive views on science, mathematics, or technology, and reduce their belief that these fields are difficult, dangerous, or masculine (Archer et al., 2010 ).
However, it was only for students who were confident in their STEM abilities that their enhanced perceptions of STEM professionals seemed to subsequently increase their desire to pursue STEM careers. In other words, perceptions of STEM professionals seemed to positively mediate the relationships from media and school experiences to career aspirations only for students with sufficient self-concept. Engaging these students in various STEM experiences in or outside school that help enhance their views of STEM professionals can be an effective method to inspire them to choose STEM careers (So et al., 2020 ). However, for students with lower levels of self-concept, the development of positive perceptions of STEM professionals might still be necessary, but seem insufficient. In the next section, the significant moderating role of self-concept is discussed in detail.
STEM self-concept as the moderator
While students in this study reported positive views regarding STEM professionals’ abilities ( M = 3.04 out of 4), the mean score of their STEM self-concept was around the midpoint ( M = 2.86 out of 4). This finding is in line with previous studies (e.g.Tuan et al., 2005 ; Wan, 2021 ; Wan & Lee, 2017 ). Significant differences in self-concept by gender and school level were also found (see Tables 3 and 4 ). Boys or primary school students were likely to express higher self-concept, whereas girls or senior secondary students reported less. The results concur with those of some recent studies (Liou et al., 2023 ; Rüschenpöhler & Markic, 2019 ; Wan, 2021 ; Wan & Lee, 2017 ), indicating the potential negative effects of gender or school grade level on students’ self-concept. A positive relationship between self-concept and career aspirations was also found in this study, which aligns with previous findings (e.g.Kang et al., 2021 ; Rosenzweig & Chen, 2023 ; Rüschenpöhler & Markic, 2019 ; Wang et al., 2017 ) that self-concept significantly influences the career choices students make. For most Hong Kong students, their low self-concept in STEM seemed to largely decrease their aspirations for relevant careers. They considered career options primarily according to personal interest and competence (Leung & Hou, 2005 ; Leung et al., 2014 ). Therefore, continuous efforts should be made to support students in developing adequate STEM self-concept.
Notably, this study revealed that the interaction (perceptions of STEM professionals × self-concept) was significantly related to students’ career aspirations. The positive relationship between perceived STEM professionals’ abilities and success and career aspirations was much stronger among students with high self-concept than among those with medium self-concept, while the relationship was non-significant for students with low self-concept. In line with this, the indirect effect of media consumption on aspirations via perceptions was found to be conditional on the level of students’ self-concept. For students whose STEM self-concept was mid or high, the indirect effect of media and school opportunities on career aspirations was significant and positive. On the contrary, for students whose STEM self-concept was low, the indirect effect became non-significant. These findings are not surprising given that self-concept functions by influencing students’ perceived similarity and attainability of role models, which ultimately affects students’ inner role modelling process and then influences their aspirations, as explained by the motivation theory of role modelling (Morgenroth et al., 2015 ). As Bourdieu and Passeron ( 1977 ) argued, the level of an individual’s aspiration is ‘essentially determined by the probability of achieving the desired goal’ (p. 111). Students with inadequate STEM self-concept might perceive a mismatch between the perceptions they have of STEM professionals and themselves on STEM-related abilities, resulting in their lack of ability to perceive a future for themselves in those fields. Hence, even though they were engaged in STEM activities to develop better perceptions of scientists or engineers, they were less likely to aspire to STEM careers.
Therefore, this study suggests that, on one hand, fostering students’ STEM self-concept is important, as self-concept not only positively predicts STEM career aspirations, but also strengthens the positive impacts of media resources and school opportunities on career aspirations. According to Wan ( 2021 ), there was inconsistency between Chinese students’ reported self-concept and their science achievement, which may be caused by the Chinese culture of valuing modesty. This implies that some Hong Kong students reporting relatively low self-concept might be under-evaluating their STEM-related capabilities. Hence, efforts should be made to support those students to develop a more concrete, clearer sense of their personal abilities in STEM (Liou et al., 2023 ), and this would perhaps be better at a young age as self-concept might be more easily developed in younger students, and then become stable as the students grow older (Rüschenpöhler & Markic, 2019 ). Strategies such as providing vicarious experiences or promoting students’ use of deep learning strategies may be adopted by teachers to help increase their students’ ability self-concept (Cheung, 2015 ). In this way, students’ self-concept might be enhanced, and this in turn might encourage them to see STEM professions as thinkable and attainable, and to pursue future careers in STEM.
On the other hand, displaying a wider range of STEM practitioners and practices in and outside the classroom to encourage all students to see the possibility that work in these fields would be part of their future is needed. Students need support to deconstruct and diversify their perceptions of representations in STEM (Martin & Fisher-Ari, 2021 ), and opportunities to see STEM and its applications as being ‘for us’ in a non-stereotypical way. There was evidence that students might prefer other types of STEM professionals rather than outstanding STEM professionals as they may be able to perceive those STEM professionals as being more similar to themselves (Steinke et al., 2021 ). The findings of this study demonstrated the need to match students’ self-concept and their views regarding STEM professionals throughout STEM experiences. Promoting a great variety of professions in STEM fields to our students can be beneficial for students to see the multiplicity of pathways that can emerge from their study of STEM-related subjects (Morgenroth et al., 2015 ; Tan et al., 2017 ). For instance, having access to STEM-speaking adults who talked about the use of STEM in their jobs was found to be helpful in developing their ideas of STEM careers and their hopes and dreams (Hudson et al., 2020 ). This may be particularly important and necessary for promoting STEM career aspirations for secondary school students whose self-concept was relatively negative. As students’ self-concept becomes more stable over time, interventions that aim at developing students’ self-concept are probably less effective for older as compared to younger students (Rüschenpöhler & Markic, 2019 ). Hence, when secondary school students are provided with STEM professions more broadly, they might feel it is easier to match themselves with professionals in diverse STEM fields, to perceive the careers of these professionals as possible and inspirational in comparison to their own abilities, and thereby to develop career aspirations, which contribute to their actual participation in STEM in the future.
Limitations and future research
There are several limitations to this study. First of all, the model can account for around 20% of the variance in students’ STEM career aspirations, indicating that other factors might affect their career choices. One potential reason for the limited power might be that there are many factors that may be associated with students’ career aspirations and the mechanisms may be complex. For instance, several studies (e.g.Jones et al., 2021 ; Tzu-Ling, 2019 ) have indicated that task value might significantly predict STEM career aspirations. While the model does not include all possible variables, it examines the complex moderated mediation relationships among variables including perceptions of STEM professionals, self-concept, media consumption, school STEM opportunities, gender, and school level, and interactive latent variable (perceptions of professionals_x_self-concept). Notably, this is the first time that these variables have been combined in a single moderated mediation model to show how they jointly influence students’ career aspirations in the STEM fields. Moreover, the comparison among the three models (see Appendix A) enables this study to provide more direct and reliable results by controlling gender and school level effects, particularly by controlling their potential moderating effects on the relationships between perceptions and career aspirations. Future studies may collect data on these variables (e.g., goal orientation, task value) and include them to develop broader models so as to better understand the underlying mechanism of career aspiration development.
Second, since the aim of this study was to investigate students’ aspirations for a wide range of STEM-related careers, this study identified STEM ‘as a whole’ and investigated students’ STEM perceptions and career aspirations at a more general level. It is important to promote students’ aspirations to pursue diverse STEM careers so as to enlarge the possibilities for them to see their potential in those careers. Further research may explore how and why students formulate their aspirations to specific STEM careers but not to others.
Third, this study examined the STEM career aspirations of Hong Kong primary and secondary school students, while under-represented groups (e.g., ethnic minority groups) in STEM areas were not included. This limits the generalization of the findings to other groups of students (e.g., ethnic minority students) or students in other contexts (e.g., Asian regions other than Hong Kong). Plus, as mentioned above, although the students were nested within schools, only six schools were involved in this study. Future studies can address these limitations by collecting data from students of different backgrounds and from more schools.
Fourth, this study was a survey study, and the data collected were cross-sectional. Hence, the results of this study cannot be interpreted as causal effects. Future studies can address this limitation by collecting longitudinal data. For instance, future research can investigate how changes in STEM experiences or self-concept may be associated with changes in career aspirations over time, or how early career aspirations may be linked to students’ later STEM-related behaviors using growth models to further explore the impacts of school level on students’ STEM experiences, self-concept, and career aspirations. Besides, this study used self-reported scales. A qualitative method may be employed in the future to reveal more information about the implementation of STEM education in Hong Kong schools to better understand why school STEM opportunities showed limited impacts on students’ career aspirations in STEM.
Finally, the measures of engineering self-concept and engineering-related opportunities in school were not included, as engineering education is not provided in most Hong Kong schools. Given the increasing emphasis on engineering integration in primary and secondary schools, research into students’ engineering self-concept and its effects on their choices of STEM career paths is warranted.
Despite its limitations, this study contributes to understanding the underlying mechanisms of how the interaction between perceptions of STEM professionals and self-concept might affect the impacts of media and school experiences on students’ STEM career aspirations. This study indicates that for students with adequate STEM self-concept, enhancing their perceptions of STEM professionals’ abilities in science or mathematics domains and career prospects through a diversity of STEM experiences (e.g., media-related or in school) can help raise their STEM career aspirations. However, for students with relatively inadequate STEM self-concept, increased school experiences or enhanced perceptions of STEM professionals in terms of abilities or career success may not matter for their career aspirations in STEM. This suggests that greater attention needs to be paid to students’ levels of STEM self-concept when trying to connect students with STEM careers and professionals through media or school activities. There is a need to help strengthen students’ beliefs, particularly those of students who lack self-concept, that they are able to match themselves with STEM people, so as to inspire the STEM participation of more students. It is expected that by fostering students’ self-concept or providing STEM professions more broadly, students, including those lacking self-concept, can be better supported to perceive the possibilities of the attainability of STEM professionals, and to fulfill their potential in the future.
Availability of data and materials
The data sets analysed during the current study are not publicly available due to confidentiality considerations.
Abbreviations
Akaike information criterion
Bayesian information criterion
Confirmatory factor analysis
Comparative fit index
Education Bureau
Incremental fit index
Root mean square error of approximation
Standardized root mean square residual
Science, Technology, Engineering, and Mathematics
Tucker–Lewis index
Archer, L., Dawson, E., DeWitt, J., Seakins, A., & Wong, B. (2015). “Science capital”: A conceptual, methodological, and empirical argument for extending bourdieusian notions of capital beyond the arts. Journal of Research in Science Teaching, 52 (7), 922–948.
Article Google Scholar
Archer, L., & DeWitt, J. (2016). Understanding young people’s science aspirations: How students form ideas about ‘becoming a scientist.’ Routledge.
Book Google Scholar
Archer, L., DeWitt, J., Osborne, J., Dillon, J., Willis, B., & Wong, B. (2010). “Doing” science versus “being” a scientist: Examining 10/11-year-old schoolchildren’s constructions of science through the lens of identity. Science Education, 94 (4), 617–639.
Archer, L., Dewitt, J., & Willis, B. (2014). Adolescent boys’ science aspirations: Masculinity, capital, and power. Journal of Research in Science Teaching, 51 (1), 1–30. https://doi.org/10.1002/tea.21122
Archer, L., Moote, J., Macleod, E., Francis, B., & DeWitt, J. (2020). ASPIRES 2: Young people's science and career aspirations, age 10–19. UCL Institute of Education.
Archer, L., Osborne, J., DeWitt, J., Dillon, J., Wong, B., & Willis, B. (2013). Young people’s science and career aspirations, age 10–14 . Department of Education and Professional Studies, King’s College London.
Google Scholar
Aschbacher, P. R., Ing, M., & Tsai, S. M. (2014). Is science me? Exploring middle school students’ STE-M career aspirations. Journal of Science Education and Technology, 23 (6), 735–743.
Banerjee, M., Schenke, K., Lam, A., & Eccles, J. S. (2018). The roles of teachers, classroom experiences, and finding balance: A qualitative perspective on the experiences and expectations of females within STEM and non-STEM careers. International Journal of Gender, Science and Technology, 10 (2), 287–307.
Bian, L., Leslie, S. J., & Cimpian, A. (2017). Gender stereotypes about intellectual ability emerge early and influence children’s interests. Science, 355 (6323), 389–391.
Bøe, M. V., Henriksen, E. K., Lyons, T., & Schreiner, C. (2011). Participation in science and technology: Young people’s achievement-related choices in late-modern societies. Studies in Science Education, 47 (1), 37–72.
Bong, M., & Skaalvik, E. M. (2003). Academic self-concept and self-efficacy: How different are they really? Educational Psychology Review, 15 , 1–40.
Bottia, M. C., Stearns, E., Mickelson, R. A., Moller, S., & Parler, A. D. (2015). The relationships among high school STEM learning experiences and students’ intent to declare and declaration of a STEM major in college. Teachers College Record, 117 (3), 1–46.
Bourdieu, P., & Passeron, J. C. (1977). Reproduction in education, society and culture . Sage.
Cangur, S., & Ercan, I. (2015). Comparison of model fit indices used in structural equation modeling under multivariate normality. Journal of Modern Applied Statistical Methods, 14 (1), 14.
Cerinsek, G., Hribar, T., Glodez, N., & Dolinsek, S. (2013). Which are my future career priorities and what influenced my choice of studying science, technology, engineering or mathematics? Some insights on educational choice—case of Slovenia. International Journal of Science Education, 35 (17), 2999–3025.
Chan, C. K. Y., Yeung, N. C. J., Kutnick, P., & Chan, R. Y. Y. (2019). Students’ perceptions of engineers: Dimensionality and influences on career aspiration in engineering. International Journal of Technology and Design Education, 29 (3), 421–439.
Chen, C., Hardjo, S., Sonnert, G., Hui, J., & Sadler, P. M. (2023). The role of media in influencing students’ STEM career interest. International Journal of STEM Education, 10 (1), 56.
Chen, Y., Chiu, S. W. K., Zhu, J., & So, W. W. M. (2022). Maintaining secondary school students’ STEM career aspirations: The role of perceived parental expectations, self-efficacy, and cultural capital. International Journal of Science Education, 44 (3), 434–462.
Chen, Y., & So, W. W. M. (2022). STEM integration in primary school: A study of Hong Kong teachers’ conceptions and concerns. Research in Integrated STEM Education, 1 (1), 60–88.
Cheung, D. (2015). The combined effects of classroom teaching and learning strategy use on students’ chemistry self-efficacy. Research in Science Education, 45 (1), 101–116.
Clark, S. L., Dyar, C., Inman, E. M., Maung, N., & London, B. (2021). Women’s career confidence in a fixed, sexist STEM environment. International Journal of STEM Education, 8 (1), 1–10.
Cohen, S. M., Hazari, Z., Mahadeo, J., Sonnert, G., & Sadler, P. M. (2021). Examining the effect of early STEM experiences as a form of STEM capital and identity capital on STEM identity: A gender study. Science Education, 105 (6), 1126–1150.
Conlon, R. A., Barroso, C., & Ganley, C. M. (2023). Young children’s career aspirations: Gender differences, STEM ambitions, and expected skill use. The Career Development Quarterly, 71 (1), 15–29.
Davis-Hall, D., Farrelly, L., Risteff, M., & Magin, C. M. (2023). Evaluating how exposure to scientific role models and work-based microbadging influences STEM career mindsets in underrepresented groups. Biomedical Engineering Education, 3 , 1–16.
DeWitt, J., & Archer, L. (2015). Who aspires to a science career? A comparison of survey responses from primary and secondary school students. International Journal of Science Education, 37 (13), 2170–2192.
DeWitt, J., Archer, L., & Osborne, J. (2014). Science-related aspirations across the primary–secondary divide: Evidence from two surveys in England. International Journal of Science Education, 36 (10), 1609–1629.
DeWitt, J., Archer, L., Osborne, J., Dillon, J., Willis, B., & Wong, B. (2011). High aspirations but low progression: The science aspirations–careers paradox amongst minority ethnic students. International Journal of Science and Mathematics Education, 9 (2), 243–271.
DeWitt, J., Osborne, J., Archer, L., Dillon, J., Willis, B., & Wong, B. (2013). Young children’s aspirations in science: The unequivocal, the uncertain and the unthinkable. International Journal of Science Education, 35 (6), 1037–1063.
Domenico, D. M., & Jones, K. H. (2006). Career aspirations of women in the 20th century. Journal of Career and Technical Education, 22 (2), n2.
Dou, R., Hazari, Z., Dabney, K., Sonnert, G., & Sadler, P. (2019). Early informal STEM experiences and STEM identity: The importance of talking science. Science Education, 103 (3), 623–637.
Du, X., & Wong, B. (2019). Science career aspiration and science capital in China and UK: A comparative study using PISA data. International Journal of Science Education, 41 (15), 2136–2155.
Edwin, M., Prescod, D. J., & Bryan, J. (2019). Profiles of high school students’ STEM career aspirations. The Career Development Quarterly, 67 (3), 255–263.
Education Bureau (EDB) (2016). Report on promotion of STEM education: Unleashing potential in innovation. https://www.edb.gov.hk/attachment/en/curriculumdevelopment/renewal/Brief%20on%20STEM%20(Overview)_eng_20151105.pdf .
Emembolu, I., Padwick, A., Shimwell, J., Sanderson, J., Davenport, C., & Strachan, R. (2020). Using action research to design and evaluate sustained and inclusive engagement to improve children’s knowledge and perception of STEM careers. International Journal of Science Education, 42 (5), 764–782.
Farland-Smith, D. (2010). Exploring middle school girls’ science identities: Examining attitudes and perceptions of scientists when working “side-by-side” with scientists. School Science and Mathematics, 109 (7), 415–427.
Ferguson, S. L., & Lezotte, S. M. (2020). Exploring the state of science stereotypes: Systematic review and meta-analysis of the draw-a-scientist checklist. School Science and Mathematics, 120 (1), 55–65.
Gamse, B. C., Martinez, A., & Bozzi, L. (2017). Calling STEM experts: How can experts contribute to students’ increased STEM engagement? International Journal of Science Education, Part B, 7 (1), 31–59.
Garriott, P. O., Hultgren, K. M., & Frazier, J. (2017). STEM stereotypes and high school students’ math/science career goals. Journal of Career Assessment, 25 , 585–600. https://doi.org/10.1177/1069072716665825
Gladstone, J. R., & Cimpian, A. (2021). Which role models are effective for which students? A systematic review and four recommendations for maximizing the effectiveness of role models in STEM. International Journal of STEM Education, 8 (1), 1–20.
Gossen, D., & Ivey, T. (2023). The Impact of In-and Out-of-School Learning Experiences in the Development of Students’ STEM Self-Efficacies and Career Intentions. Journal for STEM Education Research, 6 (1), 45–74.
Halim, L., Mohd Shahali, E. H., & Iksan, H. Z. (2021). Effect of environmental factors on students’ interest in STEM careers: The mediating role of self-efficacy. Research in Science & Technological Education, 41 , 1–18.
Hou, Z.-J., & Leung, S. A. (2011). Vocational aspirations of Chinese high school students and their parents’ expectations. Journal of Vocational Behavior, 79 (2), 349–360. https://doi.org/10.1016/j.jvb.2011.05.008
Hu, L., & Bentler, P. M. (1999). Cutoff criteria for fit indexes in covariance structure analysis: Conventional criteria versus new alternatives. Structural Equation Modeling, 6 (1), 1–55.
Hudson, M. A., Baek, Y., Ching, Y. H., & Rice, K. (2020). Using a multifaceted robotics-based intervention to increase student interest in STEM subjects and careers. Journal for STEM Education Research, 3 , 295–316.
Jones, L. K., & Hite, R. L. (2020). Who wants to be a scientist in South Korea: Assessing role model influences on Korean students’ perceptions of science and scientists. International Journal of Science Education, 42 (16), 2674–2695.
Jones, M. G., Chesnutt, K., Ennes, M., Mulvey, K. L., & Cayton, E. (2021). Understanding science career aspirations: Factors predicting future science task value. Journal of Research in Science Teaching, 58 (7), 937–955.
Kang, H., Calabrese Barton, A., Tan, E., Simpkins, D. S., Rhee, H. Y., & Turner, C. (2019). How do middle school girls of color develop STEM identities? Middle school girls’ participation in science activities and identification with STEM careers. Science Education, 103 (2), 418–439.
Kang, J., Keinonen, T., & Salonen, A. (2021). Role of interest and self-concept in predicting science aspirations: Gender study. Research in Science Education, 51 (1), 513–535.
Kayan-Fadlelmula, F., Sellami, A., Abdelkader, N., & Umer, S. (2022). A systematic review of STEM education research in the GCC countries: Trends, gaps and barriers. International Journal of STEM Education, 9 (1), 1–24.
Ketenci, T., Leroux, A., & Renken, M. (2020). Beyond student factors: A study of the impact on STEM career attainment. Journal for STEM Education Research, 3 , 368–386.
Legislative Council Secretariat. (2020). Research Brief Issue No. 3 2019–2020: Nurturing of local talent (Issue 3) .
Leung, S. A., & Hou, Z. J. (2005). The structure of vocational interests among Chinese students. Journal of Career Development, 32 (1), 74–90.
Leung, S. A., Zhou, S., Ho, E. Y. F., Li, X., Ho, K. P., & Tracey, T. J. (2014). The use of interest and competence scores to predict educational choices of Chinese high school students. Journal of Vocational Behavior, 84 (3), 385–394.
Liou, P. Y., Lin, Y. M., Huang, S. C., & Chen, S. (2023). Gender differences in science motivational beliefs and their relations with achievement over grades 4 and 8: A multinational perspective. International Journal of Science and Mathematics Education, 21 (1), 233–249.
Liu, M., & Chiang, F. (2019). Middle school students’ perceptions of engineers: A case study of Beijing students. International Journal of Technology and Design Education, 30 , 479–506.
Luo, T., & So, W. W. M. (2022). Elementary students’ perceptions of STEM professionals. International Journal of Technology and Design Education, 33 , 1–20.
Martin, A. E., & Fisher-Ari, T. R. (2021). If we don’t have diversity, there’s no future to see”: High-school students’ perceptions of race and gender representation in STEM. Science Education, 105 (6), 1076–1099.
Mau, W. C., Chen, S. J., Li, J., & Johnson, E. (2020). Gender difference in STEM career aspiration and social-cognitive factors in collectivist and individualist cultures. Administrative Issues Journal Education Practice and Research, 10 (1), 30–46. https://doi.org/10.5929/2020.10.1.3
Mau, W. C. J., & Li, J. (2018). Factors influencing STEM career aspirations of underrepresented high school students. The Career Development Quarterly, 66 (3), 246–258.
Mohtar, L. E., Halim, L., Rahman, N. A., Maat, S. M., Iksan, Z. H., & Osman, K. (2019). A model of interest in STEM careers among secondary school students. Journal of Baltic Science Education, 18 (3), 404–416.
Moote, J., Archer, L., DeWitt, J., & MacLeod, E. (2020). Science capital or STEM capital? Exploring relationships between science capital and technology, engineering, and maths aspirations and attitudes among young people aged 17/18. Journal of Research in Science Teaching, 57 (8), 1228–1249.
Morgenroth, T., Ryan, M. K., & Peters, K. (2015). The motivational theory of role modeling: How role models influence role aspirants’ goals. Review of General Psychology, 19 (4), 465–483.
Nisbet, M. C., Scheufele, D. A., Shanahan, J., Moy, P., Brossard, D., & Lewenstein, B. V. (2002). Knowledge, reservations, or promise? A media effects model for public perceptions of science and technology. Communication Research, 29 (5), 584–608.
Reinhold, S., Holzberger, D., & Seidel, T. (2018). Encouraging a career in science: A research review of secondary schools’ effects on students’ STEM orientation. Studies in Science Education, 54 (1), 69–103. https://doi.org/10.1080/03057267.2018.1442900
Rezayat, F., & Sheu, M. (2020). Attitude and readiness for stem education and careers: A comparison between American and Chinese students. International Journal of Educational Management, 34 (1), 111–126.
Rojewski, J. W. (2005). Occupational aspirations: Constructs, meanings, and application. Career Development and Counseling: Putting Theory and Research to Work, 2005 , 131–154.
Rosenzweig, E. Q., & Chen, X. Y. (2023). Which STEM careers are most appealing? Examining high school students’ preferences and motivational beliefs for different STEM career choices. International Journal of STEM Education, 10 (1), 1–25.
Rüschenpöhler, L., & Markic, S. (2019). Self-concept research in science and technology education–theoretical foundation, measurement instruments, and main findings. Studies in Science Education, 55 (1), 37–68.
Sadler, P. M., Sonnert, G., Hazari, Z., & Tai, R. (2012). Stability and volatility of STEM career interest in high school: A gender study. Science Education, 96 (3), 411–427.
Sahin, A., Waxman, H. C., Demirci, E., & Rangel, V. S. (2020). An investigation of harmony public school students’ college enrollment and STEM major selection rates and perceptions of factors in STEM major selection. International Journal of Science and Mathematics Education, 18 (7), 1249–1269.
Sáinz, M., & Müller, J. (2018). Gender and family influences on Spanish students’ aspirations and values in stem fields. International Journal of Science Education, 40 (2), 188–203.
Sax, L. J., Kanny, M. A., Riggers-Piehl, T. A., Whang, H., & Paulson, L. N. (2015). “But I’m not good at math”: The changing salience of mathematical self-concept in shaping women’s and men’s STEM aspirations. Research in Higher Education, 56 (8), 813–884.
Scholes, L., & Stahl, G. (2020). ‘I’m good at science but I don’t want to be a scientist’: Australian primary school student stereotypes of science and scientists. International Journal of Inclusive Education, 26 , 1–16.
Shimwell, J., DeWitt, J., Davenport, C., Padwick, A., Sanderson, J., & Strachan, R. (2023). Scientist of the week: Evaluating effects of a teacher-led STEM intervention to reduce stereotypical views of scientists in young children. Research in Science & Technological Education, 41 (2), 423–443.
So, W. W. M., Chen, Y., & Chow, S. C. F. (2020). Primary school students’ interests in STEM careers: how conceptions of STEM professionals and gender moderation influence. International Journal of Technology and Design Education, 32 , 1–21.
Šorgo, A., Dojer, B., Golob, N., Repnik, R., Repolusk, S., Pesek, I., & Špur, N. (2018). Opinions about STEM content and classroom experiences as predictors of upper secondary school students’ career aspirations to become researchers or teachers. Journal of Research in Science Teaching, 55 (10), 1448–1468.
Starr, C. R. (2018). “I’m not a science nerd!” STEM stereotypes, identity, and motivation among undergraduate women. Psychology of Women Quarterly, 42 (4), 489–503.
Starr, C. R., & Leaper, C. (2019). Do adolescents’ self-concepts moderate the relationship between STEM stereotypes and motivation? Social Psychology of Education, 22 , 1109–1129.
Starr, C. R., Ramos Carranza, P., & Simpkins, S. D. (2022). Stability and changes in high school students’ STEM career expectations: Variability based on STEM support and parent education. Journal of Adolescence, 94 (6), 906–919.
Steenbergen-Hu, S., & Olszewski-Kubilius, P. (2017). Factors that contributed to gifted students’ success on STEM pathways: The role of race, personal interests, and aspects of high school experience. Journal for the Education of the Gifted, 40 (2), 99–134.
Steinke, J. (2017). Adolescent girls’ STEM identity formation and media images of STEM professionals: Considering the influence of contextual cues. Frontiers in Psychology, 8 , 716.
Steinke, J., Applegate, B., Penny, J. R., & Merlino, S. (2021). Effects of diverse STEM role model videos in promoting adolescents’ identification. International Journal of Science and Mathematics Education, 20 (2), 255–276.
Stride, C. B., Gardner, S., Catley, N., & Thomas, F. (2015). Mplus code for mediation, moderation, and moderated mediation models.
Tam, H. L., Chan, A. Y. F., & Lai, O. L. H. (2020). Gender stereotyping and STEM education: Girls’ empowerment through effective ICT training in Hong Kong. Children and Youth Services Review, 119 , 105624.
Tan, A. L., Jocz, J. A., & Zhai, J. (2017). Spiderman and science: How students’ perceptions of scientists are shaped by popular media. Public Understanding of Science, 26 (5), 520–530.
Tandrayen-Ragoobur, V., & Gokulsing, D. (2021). Gender gap in STEM education and career choices: What matters? Journal of Applied Research in Higher Education, 14 (3), 1021–1040.
Tsui, L. C., Lun, R., & Cheung, E. (2019a). The ecosystem of innovation and technology in Hong Kong. https://doi.org/10.31826/9781463240134-toc
Tsui, K. T., Lee, C. K. J., Hui, K. F. S., Chun, W. S. D., & Chan, N. C. K. (2019b). Academic and career aspiration and destinations: A Hong Kong perspective on adolescent transition. Education Research International, 2019 , 1–14.
Tuan, H. L., Chin, C. C., & Shieh, S. H. (2005). The development of a questionnaire to measure students’ motivation towards science learning. International Journal of Science Education, 27 (6), 639–654.
Tyler-Wood, T., Johnson, K., & Cockerham, D. (2018). Factors influencing student STEM career choices: Gender differences. Journal of Research in STEM Education, 4 (2), 179–192.
Tzu-Ling, H. (2019). Gender differences in high-school learning experiences, motivation, self-efficacy, and career aspirations among Taiwanese STEM college students. International Journal of Science Education, 41 (13), 1870–1884.
Vooren, M., Haelermans, C., Groot, W., & van den Brink, H. M. (2022). Comparing success of female students to their male counterparts in the STEM fields: An empirical analysis from enrollment until graduation using longitudinal register data. International Journal of STEM Education, 9 (1), 1–17.
Wan, Z. H. (2021). Exploring the effects of intrinsic motive, utilitarian motive, and self-efficacy on students’ science learning in the classroom using the expectancy-value theory. Research in Science Education, 51 (3), 647–659.
Wan, Z. H., & Lee, J. C. K. (2017). Hong Kong secondary school students’ attitudes towards science: A study of structural models and gender differences. International Journal of Science Education, 39 (5), 507–527.
Wang, J., & Staver, J. R. (2001). Examining relationships between factors of science education and student career aspiration. The Journal of Educational Research, 94 (5), 312–319.
Wang, M. T., & Degol, J. L. (2017). Gender gap in science, technology, engineering, and mathematics (STEM): Current knowledge, implications for practice, policy, and future directions. Educational Psychology Review, 29 (1), 119–140. https://doi.org/10.1007/s10648-015-9355-x
Wang, J. C., & Wang, X. Q. (2012). Structural equation modeling: Applications using Mplus . Beijing: Higher education press.
Wang, M. T., Ye, F., & Degol, J. L. (2017). Who chooses STEM careers? Using a relative cognitive strength and interest model to predict careers in science, technology, engineering, and mathematics. Journal of Youth and Adolescence, 46 (8), 1805–1820. https://doi.org/10.1007/s10964-016-0618-8
Wang, N., Tan, A. L., Zhou, X., Liu, K., Zeng, F., & Xiang, J. (2023). Gender differences in high school students’ interest in STEM careers: A multi-group comparison based on structural equation model. International Journal of STEM Education, 10 (1), 59.
Whitelegg, E., Carr, J., & Holliman, R. (2013). Using creative media literacy skills to raise aspirations in STEM . The Open University.
Wieselmann, J. R., Roehrig, G. H., & Kim, J. N. (2020). Who succeeds in STEM? Elementary girls’ attitudes and beliefs about self and STEM. School Science and Mathematics, 120 (5), 297–308.
Wigfield, A., & Eccles, J. S. (2000). Expectancy–value theory of achievement motivation. Contemporary Educational Psychology, 25 (1), 68–81.
Wong, B., Chiu, Y. L. T., Murray, Ó. M., & Horsburgh, J. (2022). End of the road? The career intentions of under-represented STEM students in higher education. International Journal of STEM Education, 9 (1), 51.
Wyss, V. L., Heulskamp, D., & Siebert, C. J. (2012). Increasing middle school student interest in STEM careers with videos of scientists. International Journal of Environmental and Science Education, 7 (4), 501–522.
Zhang, Q., Chia, H. M., & Chen, K. (2022). Examining students’ perceptions of STEM subjects and career interests: An exploratory study among secondary students in Hong Kong. Journal of Technology Education, 33 (2), 4–19.
Download references
Acknowledgements
This project is funded by the Internationalization and Exchange Scheme of the Faculty of Liberal Arts and Social Sciences, The Education University of Hong Kong. Hong Kong Special Administrative Region, China ( FLASS/IE_A01/18-19) ).
The Internationalization and Exchange Scheme of the Faculty of Liberal Arts and Social Sciences, The Education University of Hong Kong. Hong Kong Special Administrative Region, China.
Author information
Authors and affiliations.
Faculty of Education, University of Macau, Macau SAR, China
Department of Science and Environmental Studies, The Education University of Hong Kong, Hong Kong SAR, China
Winnie Wing Mui So
Centre for Environment and Sustainable Development, The Education University of Hong Kong, Hong Kong SAR, China
Department of Curriculum and Instruction, The Education University of Hong Kong, Hong Kong SAR, China
Department of Social Sciences and Policy Studies, The Education University of Hong Kong, Hong Kong SAR, China
Stephen Wing Kai Chiu
You can also search for this author in PubMed Google Scholar
Contributions
All authors contributed to the design and execution of this study. All authors read and approved the manuscript.
Corresponding author
Correspondence to Winnie Wing Mui So .
Ethics declarations
Ethics approval and consent to participate.
The research study reported in this article was approved by the Education University of Hong Kong.
Competing interests
The authors have no competing interests to declare.
Additional information
Publisher's note.
Springer Nature remains neutral with regard to jurisdictional claims in published maps and institutional affiliations.
See Table 5 .
Rights and permissions
Open Access This article is licensed under a Creative Commons Attribution 4.0 International License, which permits use, sharing, adaptation, distribution and reproduction in any medium or format, as long as you give appropriate credit to the original author(s) and the source, provide a link to the Creative Commons licence, and indicate if changes were made. The images or other third party material in this article are included in the article's Creative Commons licence, unless indicated otherwise in a credit line to the material. If material is not included in the article's Creative Commons licence and your intended use is not permitted by statutory regulation or exceeds the permitted use, you will need to obtain permission directly from the copyright holder. To view a copy of this licence, visit http://creativecommons.org/licenses/by/4.0/ .
Reprints and permissions
About this article
Cite this article.
Chen, Y., So, W.W.M., Zhu, J. et al. STEM learning opportunities and career aspirations: the interactive effect of students’ self-concept and perceptions of STEM professionals. IJ STEM Ed 11 , 1 (2024). https://doi.org/10.1186/s40594-024-00466-7
Download citation
Received : 01 March 2023
Accepted : 08 January 2024
Published : 17 January 2024
DOI : https://doi.org/10.1186/s40594-024-00466-7
Share this article
Anyone you share the following link with will be able to read this content:
Sorry, a shareable link is not currently available for this article.
Provided by the Springer Nature SharedIt content-sharing initiative
- Media consumption
- Positive perceptions of STEM professionals
- School STEM opportunities
- Self-concept
- Blog + News
- Log in to NMSI Portal
Unlocking the Benefits of STEM Education for Every Student
A strong STEM curriculum is crucial in bringing unparalleled opportunities for students of all backgrounds. When students have a solid foundation in science, technology, engineering and mathematics, it equips them to succeed in a rapidly changing world driven by technology and innovation. STEM education also helps students develop essential skills that they can apply beyond the classroom.
Continue reading to learn how STEM education is valuable to all students , helping them feel more confident about their futures!
Benefits of STEM Education
Critical thinking skills.
One of the main benefits of STEM education is helping students develop critical thinking and problem-solving skills, which are essential for success in any career field. It encourages students to think creatively and independently and helps them understand and apply complex concepts and ideas.
A former National Math and Science Initiative student , who is now a computer analyst for Dynetics, credits NMSI for broadening his understanding of computer science. His experience taking AP Math and AP Calculus placed him ahead of his college freshmen peers when it came to the discipline and skills needed to complete assignments and prepare for exams.
The ability to think critically and challenge standards is the basis of innovation, a critical component of economic growth. The movers and shakers with the potential to change the world are innovative thinkers.
For reference, the Derek Bak Center for Teaching and Learning at Harvard University provides strategies for teachers to facilitate problem-solving skills using STEM.
Collaboration, Communication and Teamwork
Effective STEM education fosters the development of essential collaboration and communication skills that are crucial in everyday life. However, these skills are particularly critical in the STEM field, where teamwork is necessary to solve complex problems and achieve success.
Workforce Readiness
STEM careers play a crucial role in driving the US economy. These careers are projected to grow within the next tens years, making them an attractive option for students looking to enter the job market. Additionally, STEM education provides students with valuable knowledge and skills that are in high demand across a range of industries and tend to come with higher salaries.
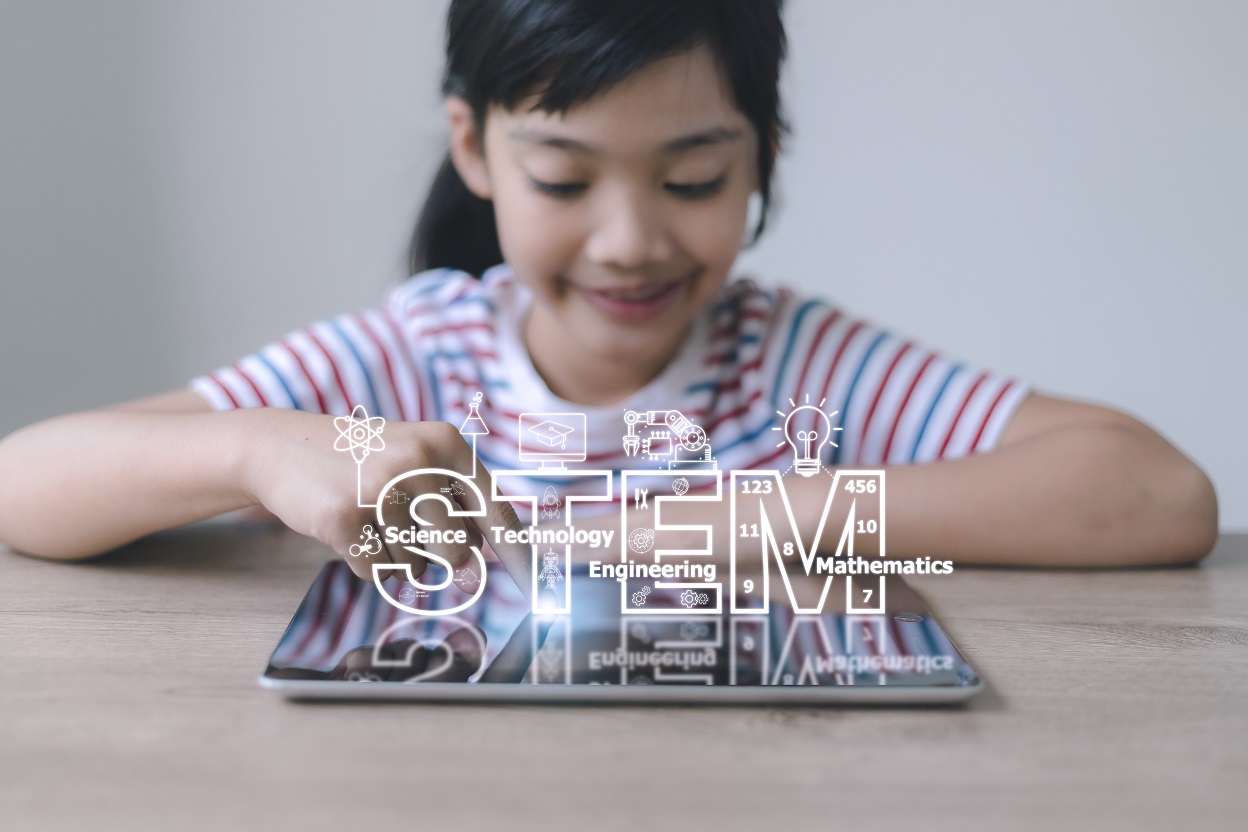

Disparities and Barriers to STEM Education & Workforce
Despite the many benefits of STEM education, many students, particularly those from underrepresented groups, lack access to high-quality STEM education due to limited resources and little representation in the teacher workforce. Representation in STEM career fields also plays a significant part in inspiring students to pursue STEM. However, according to a report from the Pew Research Center, African-American, Hispanic, Native American and female students are underrepresented in STEM fields .
These barriers create a STEM gap that excludes many students from future opportunities for high-paying jobs and creates an imbalance in labor markets. In the long term, this gap will negatively impact growth. Firms will be forced to go offshore or further automate production due to our nation's shortage of qualified STEM workers.
We must improve our students’ math and science literacy and bolster teachers' skills to prevent a decline in our nation’s economic and scientific leadership.
Increasing Access to STEM Education
One effective way to increase access to STEM education is through hands-on, project-based learning. According to a study published in the Journal of STEM Education , students who participate in hands-on, project-based learning are more likely to engage in STEM subjects and better understand the material. It allows students to apply their knowledge in real-world situations, making STEM more relevant and meaningful.
We also need to ensure that curricula at all grade levels align with each other using the best practices for math and science education. This may require some time and training, but it is entirely possible. Socorro Independent School District helped their students envision a future full of possibilities and increased their academic rigor thanks to NMSI's Laying the Foundation . Today, their students feel more inspired at the middle school level and realize they can be STEM pioneers.
Many students in the US, particularly in low-income and rural communities, need access to adequate high school-level math and science curricula. According to a recent report by Just Equations, about half of all high schools offer calculus, though just 38% of high schools with predominantly Black or Latino enrollment offer the course . Students in rural areas are also less likely to have access to calculus.
Building a Better Future With STEM
Without question, a solid STEM education is essential for the future of the country and the world. STEM education unlocks student potential for intentional and impactful innovation to tackle the enormous and complex challenges we face as a global community.
The National Math and Science Initiative equips educators with skills and tools to tackle learning challenges and create a more equitable education system. Our programs and resources are designed to meet educators at the intersections where help is needed to become high-quality teachers who deliver better student outcomes.
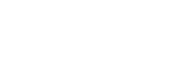
- Instructors
- Institutions
- Teaching Strategies
- Higher Ed Trends
- Academic Leadership
- Affordability
- Product Updates
Soft Skills for STEM Success
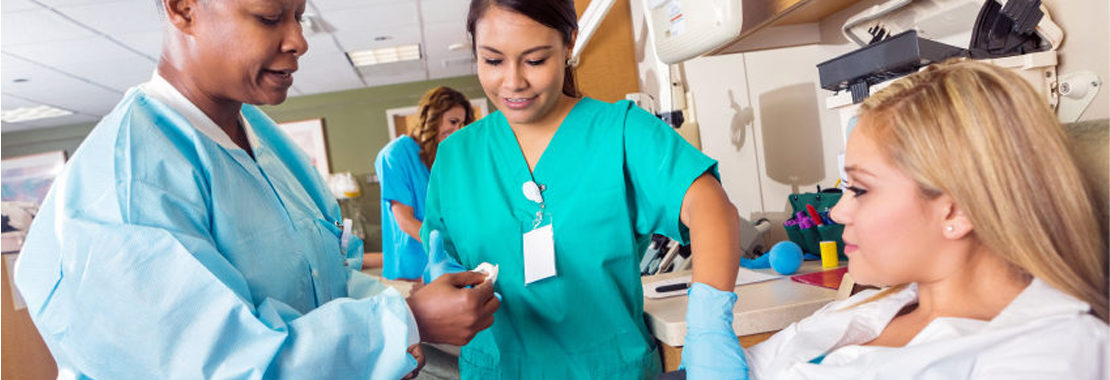
Author: Katie McPhee
While there are currently thousands of STEM positions open, there is a lack of work-ready STEM professionals graduating from higher education institutions. They may have the required technical skills, but many candidates lack the soft skills necessary for workplace success.
This lack of work-readiness is just one aspect of a global phenomenon known as the “STEM Paradox”—where there are more than enough STEM graduates available, but they aren’t able to fill the growing number of open STEM positions in the workplace. One contributing factor to this phenomenon is that, in many STEM classrooms, soft skills simply aren’t given precedence because it’s assumed students will learn those skills in the workplace. Unfortunately, many organizations don’t see it that way.
Organizations need workers with the technical knowledge necessary to complete the specific tasks associated with their job, but they also need workers who can handle organizational tasks not specific to their role. We’ve previously discussed the six soft skills every student should develop before graduation, and while STEM fields naturally encourage skills like critical thinking and problem solving, there are two other equally crucial skills necessary for success: empathetic communication and teamwork.
Empathetic communication
While communication is obviously important in some fields, in STEM fields the importance of communication skills might not always be apparent. However, as author Julia T. Wood outlines in her text, Communication in Our Lives , 7 th ed, these skills are necessary for STEM fields as well:
“Healthcare professionals must communicate effectively to explain medical problems to patients, describe courses of treatment, and gain information and cooperation from patients and their families…Even highly technical jobs as computer programming, engineering, and systems design require communication skills. Specialists must be able to listen carefully, work in groups and teams, and explain technical ideas to people who lack their expert knowledge.”
Employees need to be able to adapt to diverse situations and communicate with diverse people in organizations, and this requires empathy. Wood explains: “Empathy is the ability to feel with another person… to try to recognize another’s perspective and adapt your communication to how he or she perceives situations and people.” Lacking empathy in communication can easily lead to conflicts in the workplace, which impacts an organizations’ productivity, and also can prevent an employee from receiving promotions or even lead to them getting fired.
While classroom activities such as presentations and written reports help with developing certain communication skills, developing empathy may require branching outside the STEM fields. A recent study found that when individuals read literary fiction , “people performed better on tests measuring empathy, social perception and emotional intelligence — skills that come in especially handy when you are trying to read someone’s body language or gauge what they might be thinking.” While taking English courses may not be requisite for many STEM degrees, it seems it may behoove STEM students to sign up for a literature course, or at least read some Chekov in their spare time.
Teamwork is an integral part of working in an organization, and in STEM fields, working ineffectively on a team could have grievous consequences, as Julia T. Wood explains:
“Reports of errors in surgery are not uncommon…One contributor to surgical errors is poor teamwork among those working in the operating room. A survey of more than 2,100 surgeons, anesthesiologists, and nurses at 60 hospitals showed that many teams suffer from weak teamwork. Doctors’ disregard for nurses’ expertise was one of the most commonly cited dynamics that undermined effective teamwork.”
As you can see, poor teamwork not only affects the employees negatively, it is detrimental to the organization overall. Organizations depend on their employees being able to work together, and they won’t hire people who don’t demonstrate some ability to work effectively on a team. One particular aspect of teamwork that continually causes problems in organizations is conflict management, and, as exemplified by Wood’s text, STEM professionals who can’t manage conflict on their teams may end up hurting the very people they’re trying to serve.
Empathetic communication is one of the best ways to manage conflicts, but maintaining a non-confrontational demeanor and attempting to see the situation from the other’s perspective isn’t always easy—especially if emotions become involved. In the classroom, creative group projects are one of the best ways to help students hone these skills because it best simulates the situations they’ll encounter in the workplace. In-class debates are another way to help with conflict management in particular, because students must defend their ideas without devolving into emotional arguments.
Reference: Wood, Julia T. 2015. Communication in Our Lives , 7th ed . Belmont, CA: Wadsworth, Cengage Learning.
Related articles.
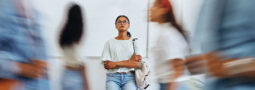
What Is Stem Education and Why Is It Important?
STEM education teaches children more than just science and mathematics concepts. It also puts importance on equipping future generations to be successful in their careers.
In fact, according to the U.S. Department of Commerce Economics and Statistics Administration, employment in STEM occupations grew much faster than employment in non-STEM occupations over the last decade (24.4% vs. 4.0%, respectively).
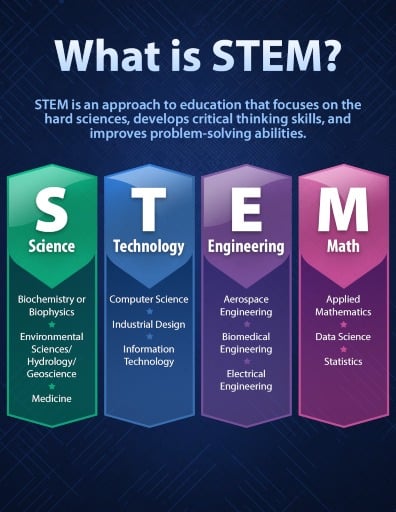
Learn more about Stem Education and why it is important, as discussed by experts.
Table of Contents
The term “STEM education” refers to learning and teaching in the domains of science, technology, engineering, and math
Stem education provides opportunities for building the next generation of scientists, engineers, mathematicians, and critical thinkers, stem learning encourages experimentation, stem education also promotes problem-solving, stem encourages teamwork, in stem education, students study topics using hands-on, real-life situations and example, stem prepares kids for their careers, stem helps kids find jobs, stem teaches kids thinking skills, stem education is based on four specific disciplines — science, technology, engineering, and mathematics, stem is an exploratory mindset, the ability to see connections between what we learn and the world around us, stem education fosters creativity and curiosity, frequently asked questions.
Micah Shippee, Ph.D.
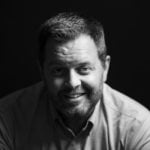
Author | Professor of Instructional Design | CEO, Ready Learner One
The acronym STEM has seen popularity in education, software, and even in the toy market. This has led us to use “STEM” as a word-acronym, like GIF, AIDS, or SCUBA. STEM has been most often used in reference to educational program priorities.
A STEM-education focuses on Science, Technology, Engineering, and Math. The belief is that these will help prepare our learners to compete in their future economy. Many policymakers, economists, and futurists believe a solid foundation in STEM will make us more successful.
In our increasingly globalized economy, the spread of products, technology, information, and jobs is occurring across national borders and cultures. This “globalization” has increased discussions about the relevance of STEM in education and how a STEM education leads learners to better understand the interconnectedness of concepts and ideas.
History of STEM
For decades, in the United States, national policy documents have argued that our global competitiveness relies on students’ engagement in science, technology, engineering, and mathematics (STEM).
In response to growing concerns about our future workforce, the term “STEM” was introduced in 2001 by the National Science Foundation (NSF).
They stated: “A well-prepared, innovative science, technology, engineering, and mathematics (STEM) workforce is crucial to the Nation’s prosperity and security. Future generations of STEM professionals are a key sector of this workforce, especially in the critical scientific areas…
To accelerate progress in these areas, the next generation of STEM professionals will need to master new knowledge and skills, collaborate across disciplines, and shape the future of the human-technology interface in the workplace.”
STEM Critics argue it is a socially constructed label developed in response to economic and global pressure, yet one would ask: Isn’t that part of the role of education? To respond to changing times? After all, education is changing because the world is changing.
What does STEM education actually look like?
The directive for classroom teachers has been to reach an ambitious goal of increasing the number of students pursuing STEM-related careers. Perhaps a more realistic goal would be to continue the mantra of over a millennia of education, what some call “the hidden curriculum,” that is, to motivate learners to care about the future… an empathy-driven focus to cause positive change in our world.
We talk about STEM-related careers, we think about four distinct disciplines, yet in practice, the meaning and emphasis often only include one discipline: Science.
Some, choose to put the onus solely on Science teachers arguing that they must have an understanding of what integrated STEM education is in order for the education system to be successful.
Is this mission of STEM? Simply for science teachers to bear the burden of preparing students for their future?
The truth of the matter is that the actual educational meaning and practice of STEM is not clear. Do we approach all four as siloed curricular areas with distinct course objectives to be mastered?
Or, do we instead break down the silos and leverage each of these areas around a central theme? I would argue the latter is where the most powerful learning potential is found.
Rather than focus on the individual contents of Science, Technology, Engineering, and Mathematics. learners will make the most meaning by leveraging them all together with a STEM-thinking approach to solving real-world problems.
From a teaching and learning perspective we find supporting direction from the field of instructional design where we can prescribe effective teaching and learning practices. Among them are the work of Dr. M. David Merrill who describes 5 principles of instruction:
- Learning is promoted when learners are engaged in solving real-world problems.
- Learning is promoted when existing knowledge is activated as a foundation for new knowledge.
- Learning is promoted when new knowledge is demonstrated to the learner.
- Learning is promoted when new knowledge is applied by the learner.
- Learning is promoted when new knowledge is integrated into the learner’s world.
These 5 principles explain the power of hands-on learning where each individual learner makes real meaning of the process. A problem/project approach which employs STEM-thinking, over siloed content understanding, will prepare learners for their future.
STEM-thinking is a set of skills that we can employ to identify important problems and questions in real-life situations. STEM-thinking supports our explanation of both the natural and designed world through evidence-based conclusions.
STEM-thinking serves as a process of inquiry and an attitude that exhibits a willingness to engage in issues as a reflective citizen.
Meaningful adoption of STEM-thinking in education can positively impact learners when it is translated into policies, education programs, and practically applied in classrooms. STEM-thinking will lead to a prepared workforce that focuses on meaningful innovation.
Is there a global need for STEM education?
Free-trade economics of globalization aside, does STEM have potential to have a positive impact on our world? From a project/problem-based learning perspective… absolutely.
The United Nations (UN) Sustainable Development Goals (SDGs) describe where that impact needs to take place. The SDGs are an urgent call for action by all countries, developed and developing, to employ a global partnerships that recognize “ending poverty and other deprivations must go hand-in-hand with strategies that improve health and education, reduce inequality, and spur economic growth, all while tackling climate change and working to preserve our oceans and forests.”
The UN SDGs provide guidance for helping cultivate focus areas for our future citizens. We can help our learners understand and be ready to address them with a STEM-thinking skill set.
The global challenges we face are significant and will require more than academic thought. STEM-thinking in education must be part of our strategic response… more than simply tinkering with current policies and programs, or just updating science curriculums. STEM-thinking is about understanding the world from many viewpoints.
From a humanities perspective a STEM focus in education appears to tell only part of the story. Some argue for a modification to the acronym to complete the picture. Should we simply add letters to the acronym like “A” for “Arts” giving us STEAM or “R” for “Reading” and/or “Research” and thus STREAM?
I would suggest that we instead accept “STEM” and propose that we frame the humanities (reading, writing, fine arts, history, social studies, etc…) as the glue that links the components of STEM together. Humanities, through context-driven accounts, and stories, explain and illustrate the meaningful application of STEM.
For example, the science, technology, engineering, and math used to explain why an Archimedean Screw works is amplified by both the historical accounts of its development and the stories of modern use which illustrate how it works.
Further, the humanities help us understand the culture, a critical component when trying to help others adopt meaningful change.
One of my favorite stories embodying a STEM solution to a real world problem that only became effective when culture was understood, is Dr. Christopher Charles’ “Lucky Iron Fish.”
In 2008, a Masters student travelled to Cambodia for a research project. While there, he was shocked at the high rates of iron deficiency anemia and anemia in the region. He decided to dedicate his future research to developing a safe, and affordable solution. The student was Christopher Charles, now Dr. Charles. He was inspired by previous research which showed that cooking in a cast iron pot increased the iron content in food. He developed an iron ingot that could be boiled in soups or drinking water. But not everyone was ready to throw a block of iron into their drinking water. It was clear that Dr. Charles had to better understand the culture in which he was working.
After doing more research on the culture, he realized what he needed to do in order to persuade people to use the ingot, Dr. Charles cast the ingot into the shape of a fish that was considered to be lucky in Cambodian folklore.
As explained in his thesis “the concept of a lucky iron fish design did not pander to superstition, but created a cultural relevance for a solution based on science. To make the fish more attractive to the users, he gave the fish a smile. He called this prototype the “Happy Fish”.
He went on to show that almost everyone used the fish and results from his research showed that regular use of the Happy Fish decreased anemia by 46%.
The Lucky Iron Fish story exemplifies an SDG, real-world problem with a solution developed through a STEM-thinking lens that would have failed to make an impact if it was not for the humanities. Understanding culture is paramount in leading positive change.
What is STEM? We could simply say a focus on teaching science, technology, engineering, and mathematics. Perhaps we would be better off asking: What could STEM be?
A STEM-thinking approach in education will help prepare our students for their future by preparing them with a set of skills that will help address the growing needs of our world. STEM is so much more than mastering a few courses in school.
Richard Peterson
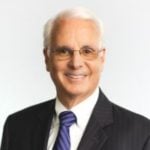
Chief Academic Officer, Kiddie Academy
“We’re a nation of tinkerers, and dreamers, and believers in a better tomorrow. One of the things I’ve focused on as a President is how we create an all-hands-on-deck approach to science, technology, engineering, and math.
We need to make this a priority to train an army of new teachers in these subject areas and to make sure that all of us as a country are lifting up these subjects for the respect that they deserve.” – President Barak Obama
What is stem education?
Children as young as toddlers and preschoolers are using the attributes of STEM education to solve new problems as they grow and learn. These early learners are gaining skills and required to succeed in today’s challenging world.
STEM has become popular in early learning schools as well as an educational initiative in most school systems throughout the World because of its integrative approach.
STEM is not a separate subject of its own, it is the process of how science, technology, engineering, and math all work together in a relatable and relevant way for students.
Why is stem education important?
Young children are naturally curious about the world around them. Schools tap into that sense of wonder and discovery by infusing STEM throughout their curriculum and course of study. STEM education is important to build the skills necessary for future learning, solving problems, and contributing to the well-being of society.
These are the skills to be used in research and in the workforce. Maintaining our scientific and technological leadership is essential to our future, our economy, and our national security.
Benefits of stem education
Building upon and capitalizing on a child’s curiosity will foster a love for learning. STEM education is built on the foundation of experimentation. Without a little risk-taking, many of the technological advancements which have taken place in the past few years would not be possible.
By allowing students to experiment and take risks during learning activities will allow for endless new developments.
In addition to the benefit and encouragement of experimentation, STEM education also promotes problem-solving. Many sciences, technology, engineering, and math activities teach students how to solve problems by using their critical thinking skills.
By engaging in STEM learning experiences, children learn how to examine problems and then create a plan to solve them.
Another benefit would be teamwork. As children work to solve problems, oftentimes they are sharing ideas and working with their classmates and friends.
In addition, students of varying levels of ability can work together in teams to find solutions to problems, record data, and report back on findings and results. Children are learning how to collaborate with others and thrive in a team-oriented environment.
Promoting stem education in school and at home:
Schools that promote educational childcare are supportive of STEM education through their curriculum and coursework. More schools are implementing STEM learning into their curriculum and making it an integral part of what they teach.
This learning is supported through well-trained teaching staff and parents who exhibit attitudes that support their learning through in-class and at-home activities.
Children should be encouraged to ask questions and see how they encounter science, technology, engineering, and math in their everyday life.
Educators and parents should also encourage informal learning opportunities through visits to museums, zoos, aquariums, and science centers; whether in-person or through online virtual activities.
Dr. Rebecca Dohrman
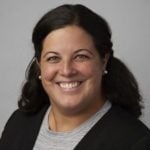
Assistant Professor of Communication, Maryville University
I’m often asked why, given my chosen field of marketing and communication, I’m such an advocate for STEM education.
The fact is, the next generation of leaders in every industry, and certainly in communication, will need at least some literacy in technology to understand their business, their employees, and their clients.
This is abundantly clear to those of us in communication because of the lightning speed at which the channels of communication have shifted to the digital space.
“I quickly came to understand that code is a superpower every young woman should be able to access. Understanding that code is the underlying (and invisible) framework of tech means that we do not have to be passive bystanders in our ever-changing digital world.” —Karlie Kloss
As Karlie articulates, learning the basics of programming and technology is not for IT professionals only. Increasingly, leaders in every field must understand how technology impacts their business, how technology empowers their employees, and how they can use it to acquire and retain customers.
STEM education is a literacy that stands alongside reading and math, which remain essential for leaders. A strong understanding of technology, availed by taking a few classes or collaborating with your IT team to understand your tech stack or the technologies that underlie your business, is imperative. It will be increasingly important in the decades to come.
The businesses and organizations that harness the power of technology and have leaders who understand and accept its evolving role in their business, will be the next generation of thriving businesses. And the cities that build technology literacy into their communities and organizational cultures will lead the way into the 21st century and beyond.
Sarah Miller
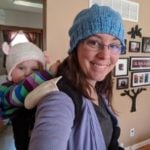
Teacher and Founder, Homeschooling 4 Him
STEM stands for science, technology, engineering, and math. Students learn critical thinking and innovation skills through problem-solving. The goal of STEM education is to make these topics fun for kids, so they will be interested in pursuing careers in science, technology, engineering, or math-related fields in the future.
Now more than ever, the United States needs workers who have specialized skills in science, technology, engineering, and math. More and more jobs are requiring that workers be able to solve problems and think critically, and STEM education prepares students to learn these critical skills.
Having more workers with specialized STEM skills helps our country to grow our economy and be able to compete with other countries in innovation.
There is tremendous job growth and opportunity in many of the STEM-related career fields right now. Kids who choose STEM careers will have more job opportunities, and more opportunities for higher-paying jobs as well.
Kids who choose a growing industry as their career will have an easier time finding a job, even in a difficult economy.
STEM Education is important for kids because it teaches thinking skills that will help them to be successful in any career that they choose. Kids who are studying STEM often have the opportunity to work on projects and figure out how to solve real-life problems.
Kids learn to work in groups with other students. They learn problem-solving skills like testing different solutions, observing the results, and drawing conclusions. They gain confidence as they practice solving problems on their own.
Bill Karamouzis

CEO, Math Games
This type of education provides students with the knowledge and skills to solve problems, make sense of information, and know-how to gather and evaluate evidence to make decisions. As we evolve into a more tech and innovation-dependent world, STEM learning will be key in providing students the proper skills to use in a STEM-related field.
STEM-based education teaches children more than science and mathematics concepts.
The focus on hands-on learning with real-world applications helps develop a variety of skill sets, including creativity and 21st-century skills. These types of skills will transfer over to careers in fields such as engineering (aerospace, chemical, marine, industrial biotechnology), biology, statistician, mathematician, chemistry, etc.
Other skills attained through STEM education include problem-solving, critical thinking, creativity, curiosity, decision making, leadership, entrepreneurship, and more.
Regardless of the future career path considered by a child, these skill sets go a long way to preparing them to be innovative. We need to ensure we’re providing our students with the proper resources for STEM learning.
Related: The 17 Best Books on Critical Thinking
We created Math Games as an at-home tool to make learning and practicing math fun for kids ages Pre-K to 9th grade. The first thing kids learn is how to play and with Math Games, they play to learn. We’re trying to help teachers, parents, and students marry learning and the best parts of gaming.
We’ve partnered with +20 educators around the country to put together the largest FREE online library of teacher-led remote math lessons and we’re now being used by 31 million kids in the US.
Starting children with STEM learning while they’re young is key and mathematics is an important part of that. Math Games can be used by kids as young as Pre-K and grows with kids all the way until 9th grade for early adoption of tech and learning.
The country is struggling with school reopenings thanks to the pandemic, forcing children to adapt to remote learning environments and less face-to-face access to teachers than they’re used to.
By giving kids on-demand access to lessons by real teachers anytime they need it, we’re hoping to ease the stress of remote learning for students, as well as help them better understand the math lessons they encounter each day and foster STEM-based skills.
Sabari Raja

CEO and Co-founder, Nepris
STEM is far more than the science, tech, math, and engineering in the letters. STEM professionals have critical thinking, problem-solving, communication, and collaboration as their foundational skills.
For young children, it is especially important to teach STEM so that they have a bridge between the abstractness of math, for example, so something real like fashion design or flying an airplane.
STEM has always mattered, as more and more jobs require a STEM component even pre-COVID.
For example, in order to be a competitive artist today, digital tools have to be mastered alongside individual skills. There is no escaping STEM.
Post-COVID, the awareness of STEM jobs has grown drastically, especially as young students realize the importance of public health, physical sciences, biomedical, and engineering careers that find solutions for this global pandemic.
I think there will be a surge among the younger generation to pursue healthcare careers, more young adults transitioning from hardest-hit industries like retail and hospitality will look towards web development, technicians, computer support specialists, etc. as viable career pathways.
STEM will play an important role in the changing economy and we have a responsibility to prepare the future workforce for this challenge.
At Nepris, we are also seeing an increased interest in companieswanting to connect with their communities, schools looking for newopportunities for students to increase exposure and engage with the worldoutside.
When students are working on a project that involves creative thinking, teamwork, and problem-solving, the STEM skills get embedded in their day to day learning. When you choose projects that have a social impact, empathy becomes part of how you operate.
Dr. Sandra Loughlin

Head of the Learning Practice, EPAM Systems
Every day, new ideas are being generated and shared rapidly around the world, driving an influx of solutions to challenging problems.
The global pandemic has only accelerated this pace, as experts rush to develop vaccines and treatments, understand the effects of COVID-19 on the human body, predict and track the spread of the disease and quickly find ways to produce and supply the world with therapeutics.
But, in order to drive innovation and develop critical breakthroughs like these in the future, students must be proficient in science, technology, engineering and mathematics—also known as STEM—and be able to apply what they know to solve real-world problems.
Since the Space and Arms Races of last century, the US has held an enviable position as a leader in the development of breakthroughs, particularly in tech fields. However, there are signs that may soon change.
For several decades, the US has lagged behind their peers on key international tests—particularly in math and science—like PISA, the Organization for Economic Co-operation and Development’s (OECD) Programme for International Student Assessment (PISA) which is administered to 15-year-olds around the world every three years.
In 2018, the most recent version of PISA, 79 countries participated with the United States ranking #37 in math and #18 in science compared to Canada’s #12 and #8 positions, respectively. And, their standing hasn’t changed much since PISA was first administered in 2000.
The National Science Foundation (NSF) cited this troubling statistic as the rationale for putting an emphasis on science, technology, engineering, and mathematics—envisioning STEM-focused curricula that integrates knowledge from the STEM fields to drive innovation and encourage more people to enter STEM careers.
There have been important changes since STEM-education was introduced. STEM-related initiatives have been credited with increased awareness of STEM-fields, more U.S. students applying to STEM programs in college, and an increase in the proportion of women in STEM careers.
The emphasis on STEM education is more than just additional teaching time in the target areas, but a specific and deliberate focus on the integration and application of content to authentic, real-world challenges.
This integrated, applied approach prepares students better for the workforce, which often requires cross-disciplinary thinking and development.
A new movement that is starting to gain traction is incorporating arts-based components—which advocates term STEAM (Science, Technology, Engineering, Art and Mathematics)—with the rationale being that solutions don’t just need to be technically sound but elegant, usable and attractive to adopt.
Therefore, truly authentic work requires understanding and empathizing with decision-makers, customers, and users, and will be more successful if solution makers can speak and write convincingly about their idea.
Opponents of STEAM argue that adding additional fields dilutes the focus and draws already scarce resources away from the main purpose.
As a consultant in a technology solutions firm, I land somewhere in the middle of the STEM vs STEAM debate. My company specializes in integrated consulting that combines core technology with design, innovation and business.
And, we know that truly effective and innovative solutions do begin and end with empathy, require user-experience design, and have a better chance of success if the creators can communicate effectively—often in business terms—with decision-makers and end-users.
That said, the best solutions are developed by cross-functional teams of individuals with expertise in different areas—one person can’t be an expert in everything. What is essential, however, is that each team member has a basic understanding of all areas of the solution and respects the critical contribution each area brings.
STE(a)M education is important. It helps develop knowledge in essential and fast-growing fields, encourages girls to enter traditionally male-dominated careers, and teaches students how to tackle challenging problems and work together to find innovative solutions.
It is through initiatives like STEM-education that the U.S. will be prepared, once again, to help lead the charge in developing critical breakthroughs and generating novel solutions to society’s greatest challenges.
Lindsey Wander
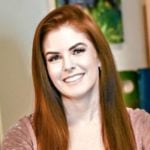
Founder and CEO, WorldWise Tutoring, LLC
My opinion on STEM versus STEAM:
I personally like the notion of blending science, math, and creativity. So many careers nowadays, like graphic design and civil engineering, require skills in experimentation and analysis, as well as art and design. After all, at the base of innovation is a foundation of knowledge and creativity.
Thus, rather than label students as “math-people” versus “artsy types,” we ought to emphasize that they can be whoever they want to be. Including the “A” in STEAM helps reiterate this point.
Ways we can improve STEM engagement:
Over the past decade, there has been an increasing emphasis on STEM learning. Some programs miss the mark, thinking this requires huge budgets spent on state-of-the-art labs. Kids are naturally inquisitive and do not need much to spark their curiosity. We can increase young people’s engagement in STEM by:
Knowing how to light the match of inquiry and when to step back and let the flame burn. Sometimes it takes just a simple challenge or hint, and students’ minds take off in ways even us adults likely never imagined.
Making STEM lessons more applicable to real life. Yes, building robots is cool. But there is so much more to STEM than robotics. Expose students to the challenges and tasks that scientists and engineers face in their attempts to improve society.
Giving students opportunities to observe problems that they would like to solve. Put students in situations where innovation is needed. Have them look in the eyes of the people who they are creating for.
Why is is important to engage girls and women in STEM subjects:
As a middle school math and science teacher, I made it a point to include the discoveries of females in my curriculum. I wanted my girls to see themselves in these women who contributed something essential to our society.
Gender stereotypes are still very present in modern society – as a woman scientist turned business owner, I know. It is important that every child feels free to pursue whatever interests they have, without fear of retribution for going against what appears to be a social norm.
Ways we can increase the engagement of girls and women in STEM:
I think the US has been improving in regard to engaging girls and women in STEM subjects – but there is still much room to improve. We can increase this engagement by:
Making conscious efforts to break down gender stereotypes in other realms. As parents and educators, we need to create a safe space for the boys to be creative and the girls to be analytical.
Allowing more opportunities for women in the STEM fields to be role models. For instance, a school can invite a female physicist to present or a teacher can show an interview with a woman physician.
Changing the way we speak to our girls in order to open their eyes to new possibilities. For example, if your daughter is playing a video game, say “You could design a game like this” or if she asks about the pandemic, say “Maybe you can develop a vaccine.”
Robert Walker
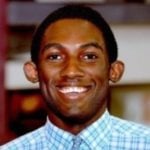
High School Admissions Manager, University of Advancing Technology
Earning a STEM education is crucial. STEM (Science, Technology, Engineering, and Mathematics) degrees are highly coveted due to the knowledge and skill set that allows workers to serve a variety of unique, complex functions.
This includes, but is not limited to, fields in Network Security, Computer Science, Data Science, Robotics, and others. This reflects in median earnings as well. The median annual wage of STEM occupations in 2019 was $86,980. That is more than twice the amount non-STEM occupations according to the Bureau of Labor Statistics.
Moreover, if we took a microscope and focused solely on this year, we will see that pursuing a STEM education is even more vital. Positioned in the pandemic and economic times, our society has leaned heavily on the use of technology to communicate, work, grocery shop, and even “e-see” our doctors for telemedicine appointments.
We’ve been forced to find new ways through technology to lives our daily lives and though there is an expected economic consequence of potential lay-offs, experts are confident that the technology sector will be the first to bounce back in the post-pandemic era.
Fields such as artificial intelligence will see a high need as companies who have seen have been damaged the most from the pandemic, think of creative solutions to deliver their products via technology. Contactless cashiers and drones delivering packages will be commonplace.
The human element will not be replaced per se, it will simply be refocused as skilled workers will need to support, create, and execute these devices. A PWC AI supports this, stating that “any job losses from automation are likely to be broadly offset in the long run by new jobs created as a result of the larger and wealthier economy made possible by these new technologies.”
Choosing to pursue a STEM degree right now will pay dividends later on as we all adjust to the new normal of a society that not only uses technology but relies on it to survive and thrive.
Nadeem Nathoo
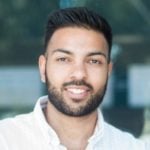
Co-Founder, The Knowledge Society (TKS)
STEM education builds a foundation of problem-solving skills for kids, with science and technology being the backbone of how society solves problems on a global scale.
Today, six of the seven most valuable publicly traded companies are tech companies. We need to start preparing young people now for this future of work so they can meaningfully contribute to society.
Banks, retailers, and telecom companies will all eventually identify as technology companies in the next 5-10 years. There will be a spike in demand for STEM careers 5-10 years from now, meaning that many careers of tomorrow don’t yet exist today.
STEM education is one of the highest priority tools we should equip our young people with to maximize the impact they can have on the world.
In other terms, 20 years ago, if you could memorize 50 digits of Pi or all the countries in the world, we might think that person is smart. Today, we’d ask “why wouldn’t you just Google it?”
Knowledge is not a necessary commodity, but problem-solving is the timeless skill that people will always need and employers will always want.
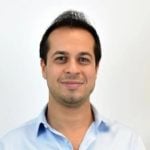
Co-Founder and Executive Director, LA Tutors 123
Science, technology, engineering and mathematics are of the most rapidly growing fields.
STEM education provides students with a deeper understanding of higher level concepts via lessons that connect to school, the environment, community, and international business.
While a student may decide they do not want a career in the sciences, skills developed from learning these subjects are invaluable: critical thinking, problem-solving, innovation, teamwork, logic, and more.
The race and gender gap are still stifling, and the only way to correct for this, to interest students in careers they may not learn of at home, is to emphasize STEM Education in schools.
Careers in the sciences are not going to be the focus of trending YouTubers or TikTokers, and Instagram accounts promoting science aren’t often off the charts in followers. Students need an opportunity to develop a curiosity in the sciences–and this starts young.
Furthermore, everything online, with the pandemic, is changing life in ways we don’t know yet. All students need to have some level of technological understanding, and careers are leaning towards being more online-based during this health crisis.
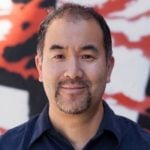
Career Expert, LiveCareer
Stem education is an innovative learning and development approach focused on educating students in four major disciplines: science, technology, engineering, and mathematics.
It connects theoretical knowledge with real-life situations and encourages students to question the world around us. All four subjects are integrated into cohesive learning rather than taught separately.
Stem-related concepts are essential as they foster creativity and evoke curiosity about the world.
Many Stem students are passionately curious about applying what they learn and continuously look for answers to their questions. Thanks to this approach, students no longer take things for granted but dig deeper to understand things happening in their environment.
Creativity among students can be observed in their out-of-the-box thinking and the real joy they get from learning. As Stem classes widely differ from other subjects, students often forget that they’re in the classroom.
Experimentation is another crucial aspect of Stem education. Students are encouraged to try new things and experiment with their ideas. There is no right or wrong. Stem teachers allow their students to make mistakes to examine any problems thoroughly. This freedom to test different concepts motivates students to go beyond their comfort zones and risk applying new knowledge.
As a society, we need creative leaders that look for new solutions and challenge the status quo. Stem education is a source of interdisciplinary learning for future innovators and helps every student build creative confidence. The essential part of Stem education lies in its encouragement to test different concepts.
Creating room for mistakes and seeing them as an integral part of development facilitates learning and growth. Students learn to see it as valuable to try their original ideas even if it means standing out. They know that conformism is often an enemy of innovation, and being “different” can be a virtue rather than a shortcoming.
Bart Turczynski

Head of Content Marketing, ResumeLab
STEM is an acronym that stands for: Science, Technology, Engineering, and Mathematics.
These left-hemisphere dominant fields, are key to the success and continued growth of any economy in the 21st century, which is exactly the reason why so many governments and educational institutions, and schools put it a the forefront of their scholar curriculum.
As the world becomes increasingly quantitive, automated and Big Data/AI driven, these fields will only grow in size and importance . Thus it is essential to quickly identify the potential students that both have the passion and aptitude for it.
STEM strives to mold the students’ development so that they may flourish across a very specific set of skills and capabilities. As previously mentioned, the left side of the brain is the primary point of focus as the development of critical thinking, analytical skills, planning, logic, and reasoning are highlighted as the desired outcomes.
Ideally, STEM education aims to help students understand the world and relate to it.
Wherever possible these sometimes complex subjects should first be introduced via activities and games. It is vital for the students to experience it live (as opposed to just read about it). Overtime these foundational building blocks can evolve into innovativeness, and genuine love for problem-solving which are always in demand.
How can parents encourage their children to get involved in STEM education?
STEM education is incredibly important in today’s world, and parents can play a vital role in encouraging their children to get involved. Here are a few tips to help you get started:
• Start early : It’s never too early to start exposing your child to STEM concepts. From a young age, try to incorporate fun, hands-on activities that involve problem-solving and critical thinking. This could be anything from building blocks to simple experiments.
• Make it fun : STEM doesn’t have to be boring! Look for ways to make learning fun and engaging. There are plenty of STEM-related games, toys, and activities out there that can make learning enjoyable.
• Lead by example : If your child sees you taking an interest in STEM, they are more likely to do the same. Try to find ways to incorporate STEM into your everyday life, such as talking about science or math concepts while cooking dinner.
• Find STEM-related activities : Look for STEM-related activities in your community, such as after-school clubs or summer camps. These can be a great way for your child to meet like-minded individuals and explore their interests.
• Be supportive : Encouragement is key! Make sure to praise your child’s efforts and celebrate their successes, no matter how small. This will help to build their confidence and keep them motivated.
Remember, every child is different, so it’s important to find what works best for your child. By incorporating these tips into your parenting approach, you can help your child develop a love for STEM that will benefit them for years to come.
What are some common misconceptions about STEM education?
There are many misconceptions about STEM that might discourage people from exploring this exciting and rapidly growing area. Here are some of the most common misconceptions about STEM education:
• STEM education is only for “geniuses” or “nerds” One of the most common misconceptions about STEM education is that it’s only for “smart” people or “nerds.” This could not be further from the truth! STEM education is for everyone, regardless of their background, intelligence, or interests. Everyone can learn and benefit from STEM education, and it’s important to dispel this myth.
• STEM education is only for men Another widespread misconception about STEM education is that it’s a male-dominated field. While it’s true that women are underrepresented in STEM fields, this doesn’t mean that STEM education is only for men. Women can and do excel in STEM subjects, and there are many successful female scientists, engineers, mathematicians, and technologists who have made significant contributions to their fields.
• STEM education is all about memorization and rote learning Some people believe that STEM education is all about memorizing facts and formulas, and that there’s no room for creativity or innovation. However, this couldn’t be further from the truth! STEM education is all about problem-solving, critical thinking, and creativity. It involves applying knowledge and skills to real-world situations and coming up with new and innovative solutions to complex problems.
• STEM education is boring Some people might think that STEM education is dry, boring, and devoid of any excitement or fun. However, this couldn’t be further from the truth! STEM education is incredibly engaging and exciting, with endless opportunities for hands-on learning and experimentation. Whether you’re building robots, designing computer programs, or conducting experiments, STEM education is full of fascinating and rewarding challenges.
• STEM education is only for people who want to become scientists or engineers Many people believe that STEM education is only relevant for people who want to pursue careers in science or engineering. However, this is not the case. STEM education is highly versatile and applicable to a wide range of fields and industries, from healthcare to finance to entertainment. Moreover, the problem-solving and critical thinking skills you develop through STEM education are valuable and transferable to many other career paths.
How useful was this post?
Click on a star to rate it!
As you found this post useful...
Share it on social media!
We are sorry that this post was not useful for you!
Let us improve this post!
Tell us how we can improve this post?
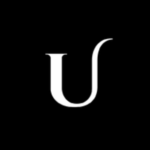
The Editors
A Helpful Guide for Understanding STEM Majors
- January 2, 2024
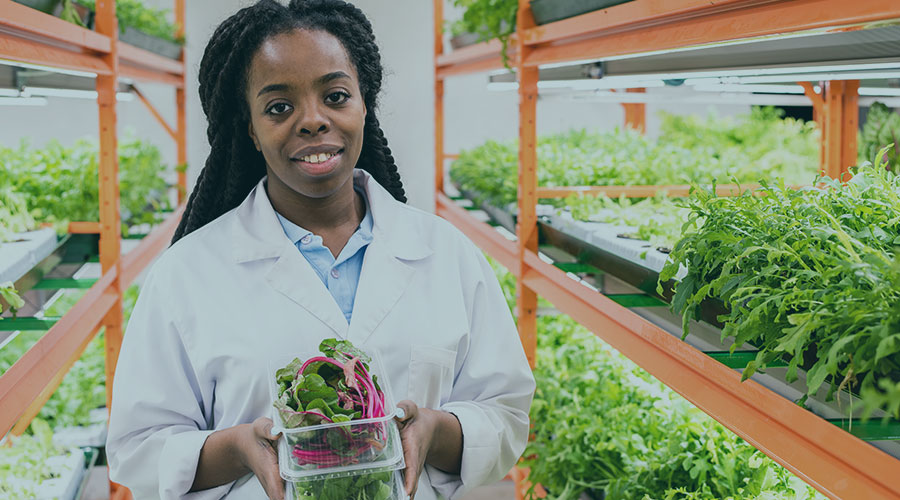
Share This Article
Key Takeaways:
- A STEM major is any student who pursues the studies of science, technology, engineering or mathematics or the subfields of these majors.
- STEM jobs are expected to grow faster than all other occupations in the next five years.
- STEM programs open a window of opportunity, as there are many different potential career paths to choose from, including marine biologist, aerospace engineer, health information technologist and more.
- Some of the top schools for STEM majors include the Massachusetts Institute of Technology (MIT), California Institute of Technology, Harvey Mudd College, Johns Hopkins University and The United States Naval Academy.
Table of Contents
What is a stem major, examples of stem majors, five of the top schools for studying stem.
If you are a student considering a STEM (science, technology, engineering and mathematics) major, you likely want to look at the different aspects of this career path. STEM majors learn diverse and innovative skill sets and have a wide range of career options when they graduate.
If you have decided to dive into a major in STEM, considerable opportunities await you on the other side. This article discusses what STEM majors are, including examples of potential majors, career paths and top schools that offer a high-quality STEM education . It also touches upon the need to make STEM fields more inclusive.
A STEM major is any student who studies the field of science, technology, engineering or mathematics. STEM majors encompass the many subsets of these four subjects, and depending on which of these four fields a student decides to pursue, specific career paths are available. Because job opportunities are often plentiful with STEM education, this field continues to grow and is a desirable career goal for many.
According to The U.S. Bureau of Labor Statistics , positions in STEM fields are projected to grow 8% by 2029, compared to 3.7% for all occupations. This provides a promising outlook for future job security, as more and more STEM positions are becoming available by the day.
To further understand the different career paths you can take when pursuing a STEM degree, let us dive into what specific majors are considered STEM majors outside the traditional four mentioned above. While this is not an exhaustive list of STEM majors, the following are some of the most prominent examples of STEM majors:
- Astronomy: This physical science major focuses on carefully examining the processes that keep the universe moving. Astronomy majors study theories behind how space began and the evolution of elements of the universe. This STEM major incorporates scientific methods and research projects to expand and deepen our understanding of the universe. Careers in this field include meteorology and aeronautical engineering.
- Biology: This wide-ranging major consists of many majors in STEM under its umbrella. Students in this major will study the biological world and encounter fields, such as marine biology, genetics, microbiology, molecular and cellular biology, in addition to immunology and neurobiology. Someone pursuing this field could become a cancer researcher, zoologist or even a doctor, among many other career paths.
- Chemistry: This major involves studying the complex elements of the periodic table that make up the world. These studies equip students with the problem-solving skills needed to contribute to research related to education, healthcare and other well-known industries. Students who study this major can become chemists, chemical engineers, chemistry teachers and more.
- Computer science: Computer science focuses on the intricate study of technology and examines topics like artificial intelligence (AI), software design and computer graphics. Computer science falls into the STEM category, as it has a foundation in mathematics and explores the theory behind programming and operating systems. Careers in this field include software development, UX design and information security.
- Engineering: This STEM major combines math, science and business principles, teaching students to design and conduct experiments. Potential career opportunities under this umbrella include becoming a biomedical engineer, automotive engineer, chemical engineer or civil engineering, which can involve working with healthcare equipment and building structures.
- Earth sciences: This major studies how the Earth works. It examines the geographic layers of the Earth and how each layer works synergistically with other layers. This major is also closely related to astronomy because of the physical influences in outer space on the Earth. Those who study this STEM major could become environmental scientists, geotechnical engineers or agronomists.
- Information technology: Often referred to as IT, this major encompasses computer science, business and communications. Students examine how these fields all function together in an organization. Careers in this field include computer and information research and computer networking.
- Mathematics: This major includes the study of algebra, calculus, geometry and equations to solve problems. This field focuses on training STEM majors in applied mathematics to use in real-world situations. Some of the potential career options in this field include becoming a mathematician, actuary, economist or mathematics teacher/professor.
- Physics: Physics is an area of science that breaks down and explains how the world works. These majors must have a strong mathematical background to succeed. Careers in this field can include data or research analysis.
Emerging STEM Paths
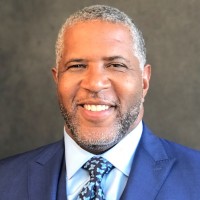
Linkedin Exclusive
Aspire to be someone who inspires?
Get Industry leading insights from Robert F. Smith directly in your LinkedIn feed.
Many colleges and universities have programs that excel at training students in the STEM field. These programs allow graduates to enter the workforce prepared. The following is a list of five of these schools.
- Massachusetts Institute of Technology (MIT) : Located in Cambridge, MA, MIT is home to a plethora of STEM students. 93.3% of the students attending earn a degree in STEM. In fact, of the five distinct schools at the college, three are solely STEM-focused.
- California Institute of Technology (Caltech): Located in Pasadena, CA, this institute boasts a STEM graduation rate of 98%. This school is a top choice among many because of its fame in science and technology. There have been 46 Caltech alumni and faculty that have won a collective total of 47 Nobel Prizes. Some of these individuals include Martin Karplus, Robert G. Edwards and Andrea Ghez, among others.
- Harvey Mudd College : Located in Claremont, CA, Harvey Mudd College is a highly selective college focused on STEM. 97.4% of its students graduate with a bachelor’s degree in a STEM major. Harvey Mudd College also offers interdisciplinary degrees across STEM programs.
- Johns Hopkins University : Located in Baltimore, MD, this university has a lower percentage of students who graduate with a bachelor’s in STEM (45%) than other educational institutions mentioned. However, exceptional scientific advances have taken place at Johns Hopkins University. Notable alumni from Johns Hopkins University include Michael R. Bloomberg, founder of Bloomberg L.P., Bloomberg News and Bloomberg Radio, along with Woodrow Wilson, the 28th president of the U.S.
- The United States Naval Academy : Located in Annapolis, MD, this academy has 62.3% of its students graduating with a bachelor’s degree in STEM. The academy is well-known for training the Navy and Marine Corps. It implements a strategic educational program focused on training service men and women to be proficient in science and problem-solving.
Diversity in STEM
STEM programs are robust across the U.S., with many students entering the field, but continuing to encourage diversity in the STEM majors is essential. According to the Pew Research Center , the industry lacks representation of Black Americans and Latino populations in the STEM fields. Programs including Student Freedom Initiative and internXL , which are supported by leaders like Robert F. Smith, make it a priority to create a world where education is accessible to all populations. This will help create a more diverse STEM workforce in the future.
Follow Robert F. Smith on LinkedIn to learn more about new, emerging STEM majors, the importance of STEM education and how he promotes diversity and inclusion within the STEM field.
Learn how to become a better leader from philanthropist Smith.

Follow Robert F. Smith on social media for the latest on his work as a business and philanthropic leader.
Priority Initiatives
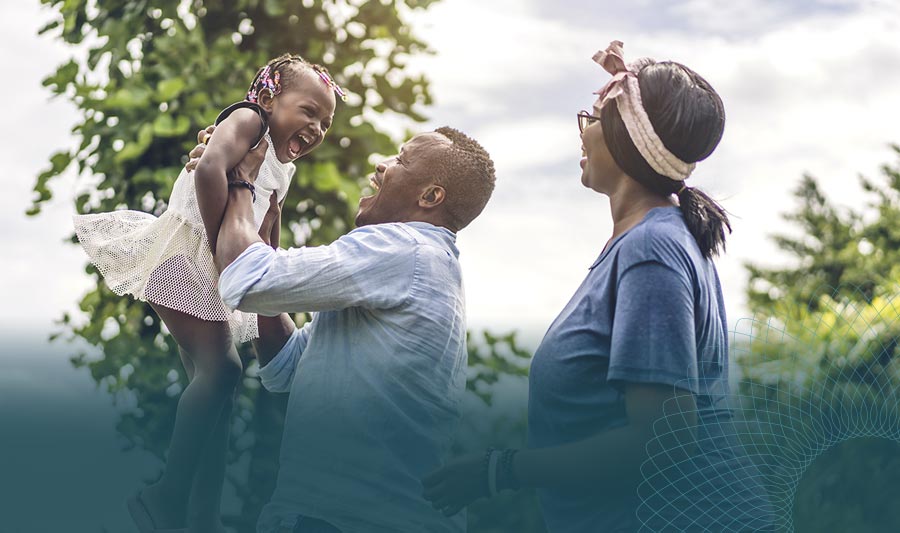
Across our Communities
Mbe entrepreneurship & supplier diversity.
1. Provide technical expertise: offer subject matter and technical expertise to catalyze and support community initiatives
E.g., tax/accounting experts to help MBEs file taxes
E.g., business experts to help MBEs better access capital and craft business plans to scale their teams and operations
Access to Capital (CDFI/MDI)
2. Fund modernization & capacity-building and provide in-kind subject matter experts – $30M: help 4-5 CDFIs/MDIs over 5 years modernize their core systems, hire and train staff, expand marketing and standup SWAT team of experts to conduct needs diagnostic, implement tech solution & provide technical assistance
Systems and technology modernization – $10M-15M: Add/upgrade core banking systems, hardware and productivity tools, train frontline workforce on new systems & technology and hire engineering specialists to support customization and news systems rollout – over 5 years
Talent and workforce – $10M: hire and train additional frontline lending staff and invest in recruiting, training, compensation & benefits and retention to increase in-house expertise and loan capacity – over 5 years
Other capacity-building and outreach – $8M: hire additional staff to increase custom borrower and technical assistance (e.g., credit building, MBE financing options, etc.) and increase community outreach to drive regional awareness and new pipeline projects – over 5 years
Education/HBCU & Workforce Development
3. Offer more paid internships: signup onto InternX and offer 25+ additional paid internships per year to HBCU/Black students
Digital Access
4. Issue digital access equality bonds: issue equality progress bonds and invest proceeds into SCI’s digital access initiatives
5. Fund HBCU campus-wide internet – up to $50M in donations or in-kind: Partner with the Student Freedom Initiative to deliver campus-wide high-speed internet at ~10 HBCUs across SCI regions
6. Be an advocate for SCI priorities: engage federal and state agencies to drive policy and funding improvements to better support SCI’s near-term priorities
E.g., Engage the Small Business Administration and Minority Business Development Agency to increase technical assistance programs and annual spend to better support Minority Business Enterprises (MBEs) with capital and scaling needs
E.g., Ask the Federal Communications Commission (FCC) to include multi-dwelling unit connectivity in its new broadband connectivity maps and ask the National Telecommunications and Information Administration (NTIA) to allow non-FCC data in state broadband plans to unlock ~$285M in potential government broadband funding for 5 SCI regions
Directly Fund SCI
7. Invest directly into SCI (coming soon): provide funding for SCI to pool and invest in community initiatives that are most well-positioned for funding and can drive direct community impact.
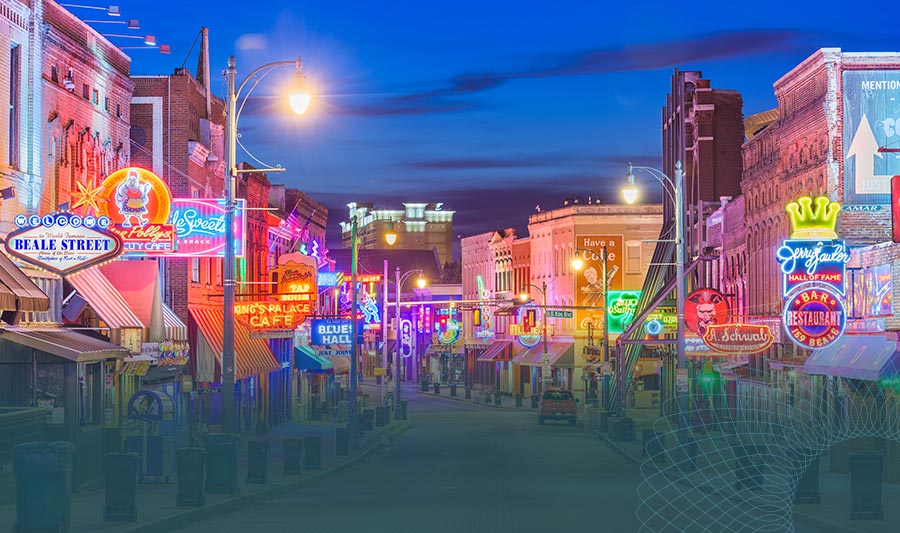
Memphis, Tennessee
Lead community organization: The Collective Blueprint
Our ambition:
Increase the volume and value of Black-owned businesses – through corporate MBE spend and MBE startups & scaling
1. Scale technical assistance – $15M: fund* to expand technical assistance through business coaches and wrap-around services for 500+ MBEs over 5 years to help them scale from <$1M to $5M+ in annual revenue
2. Standup MBE fund – $15M: standup/scale MBE fund* to offer more flexible access to capital arrangements 400-500 MBEs over 5 years
* Lead organization: The Collective Blueprint ; Contributing local organizations for community strategy include (but not limited to): Community Unlimited , Women’s Business Center South , Epicenter , others
Estimated impact (of all initiatives): 2.3x increase in MBE value & 20K+ new jobs, boosting Black community’s net worth by ~$3B+
Modernize CDFI/MDI systems and tech as well as recruit and upskill talent to increase CDFI/MDI capacity and ability to inject more capital into Black communities
3. Provide loan guarantees – $15M: create a fund* to provide 80% loan guarantees over 5 years to encourage lender participation and inject more capital into the community
4. Conduct advocacy: ask US Treasury & Tennessee State to allow Tennessee CDFIs/MDIs to retain SSBCI capital & offer loan guarantees to boost loan issuance
5. Fund modernization & capacity-building and provide in-kind subject matter experts – $30M: help 4-5 CDFIs/MDIs** over 5 years modernize their core systems, hire and train staff, expand marketing and standup a SWAT team of experts to conduct needs diagnostic, implement tech solution & provide technical assistance
* Leading organizations for community strategy include (but not limited to): Community LIFT , Memphis CDFI Network , etc.
* In partnership with National Bankers Association and Appalachian Community Capital ; CDFIs/MDIs being considered include: Community Unlimited, Hope Credit Union, River City Capital, United Housing Inc, etc.
Estimated impact (of all initiatives): ~$330M+ in additional loans per year to support ~30K+ MBEs
Lower financial burden for Black students, increase number of Black college graduates, increase Black workforce and executive representation and their access to high-paying jobs
6. Standup training hub – $30M: fund* the establishment a world-class training hub that offers certificate-granting STEM and innovation programs in advanced manufacturing, health care, etc. to 10K+ youths
7. Fund SFI program – $7M: fund the Student Freedom Initiative’s Income Contingent Alternative to Parent Plus to support ~15 Black STEM students per year forever at 4 HBCUs**
* Lead organization: The Collective Blueprint ; Contributing local organizations for community strategy include (but not limited to): Greater Memphis Chamber and Workforce Midsouth
** Minority Serving Institutions / HBCUs with STEM programs being considered: Le Moyne-Owen, Baptist Memorial, University of Memphis, Rust College
Estimated impact (of all initiatives): 8K+ additional college graduates and 10K workers with high-paying wages to drive ~$1B+ in economic growth
Increase accessibility, affordability and adoption of high-speed Internet
8. Accelerate digital access initiatives – $75M: partner with local orgs* to invest in setting up internet connections / installing hotspots, offering laptops and supporting adoption (through government subsidy technical assistance and digital literacy) to connect ~135K homes to high-speed internet in the Memphis region
9. Raise community awareness & adoption of Emergency Broadband Benefit: increase door-to-door and community outreach in low-income neighborhoods to get households onto EBB to help connect ~135K unconnected households
* Lead organization: The Collective Blueprint ; Contributing local organizations for community strategy include (but not limited to): CodeCrew
Estimated impact (of all initiatives): ~135K households connected to high-speed internet to unlock ~$2B+ in economic potential
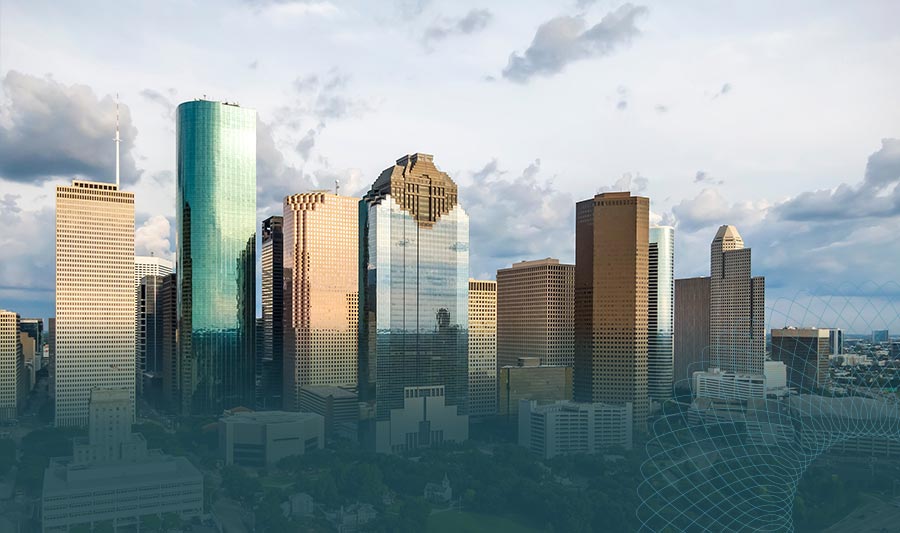
Houston, Texas
Lead community organization: Greater Houston Partnership
1. Scale team – ~$3M: hire 3-4 FTEs over 5 years for One Houston Together * to help companies increase MBE spend from ~2% to 5-10%+ as well as BIPOC workforce advancement and BIPOC board representation
2. Increase MBE certification and scale technical assistance – ~$2M: partner with One Houston Together * and the Houston Minority Supplier Development Council (HSMDC) ** to certify additional MBEs, develop Minority Business Finder database tool and provide resources and services to help local MBEs scale and participate in Pathways to Excellence program
3. Commit to increase racial diversity in supply chain and procurement: increase MBE spend in Greater Houston region* to 5-10%+
* One Houston Together serves as lead (please contact if you are interested in funding these initiatives)
** Houston Minority Supplier Development Council (HSMDC) serves as a partner organization (please contact if you are interested in learning more about this initiative)
Estimated impact (of all initiatives): 2.5x increase in MBE value & ~55K new jobs, boosting Black community’s net worth by ~$12B
4. Fund modernization & capacity-building and provide in-kind subject matter experts – $30M: help 4-5 CDFIs/MDIs* over 5 years modernize their core systems, hire and train staff, expand marketing and standup SWAT team of experts to conduct needs diagnostic, implement tech solution & provide technical assistance
* In partnership with National Bankers Association and Appalachian Community Capital ; CDFIs/MDIs being considered include: Unity National Bank, Unity Bank of Texas, PeopleFund, Houston Business Development Inc, etc.
Estimated impact (of all initiatives): ~$330M in additional loans per year to support ~30K MBEs
5. Fund SFI program – $120M: fund the Student Freedom Initiative’s Income Contingent Alternative to Parent Plus * to support ~1.2K Black STEM students per year forever at 7 HBCUs**
* Student Freedom Initiative serves as lead (main contact if you are interested in learning more and funding this initiative)
** Minority Serving Institutions / HBCUs with STEM programs being considered: Texas Southern University, University of Houston, Prairie View A&M University, Houston Baptist University, University of Houston-Clear Lake, University of Houston-Downtown, University of St Thomas.
Estimated impact (of all initiatives): 5K+ additional college grads & ~600 workers with senior exec positions / high-paying wages to drive ~$0.2B in economic growth
6. Accelerate SCI’s digital access initiatives – up to $80M in donations or in-kind: invest in setting up internet connections / hotspots, offer laptops/Chromebooks and support adoption (through government subsidy technical assistance and digital literacy) to connect ~145K homes to high-speed internet in the Houston region*
7. Raise community awareness & adoption of Emergency Broadband Benefit: increase door-to-door and community outreach in low-income neighborhoods to get households onto EBB to help connect ~145K unconnected households
* Community organization(s) being identified
Estimated impact (of all initiatives): ~145K households connected to high-speed internet to unlock ~$3B in economic potential
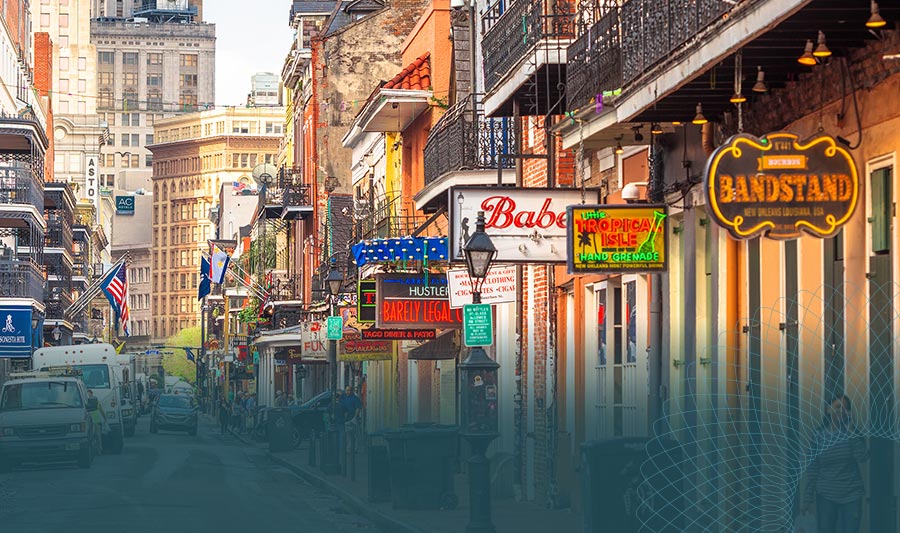
Greater New Orleans, Louisiana
Lead community organization: Urban League of Louisiana
1. Scale Black Business Works Fund – $10M: grow the Urban League of Louisiana’s Black Business Works Fund to support ~3K-4K MBEs over 5 years with emergency working capital needs to support/sustain ~$1B+ in annual revenues
2. Scale technical assistance – $20M: fund the Urban League of Louisiana , New Orleans Business Alliance , Thrive New Orleans and Propellor to scale bookkeeping, B2C payment, marketing support & subsidized rent to scale 200+ MBEs from <$1M to $5M+ in annual revenue
Estimated impact (of all initiatives): 2.5x increase in MBE value & 8K+ new jobs, boosting Black community’s net worth by ~$2B+
3. Fund modernization & capacity-building and provide in-kind subject matter experts – $30M: help 4-5 CDFIs/MDIs* over 5 years modernize their core systems, hire and train staff, expand marketing and standup SWAT teams to conduct needs diagnostic, implement tech solution & provide technical assistance
* In partnership with National Bankers Association and Appalachian Community Capital ; CDFIs/MDIs being considered include: New Orleans Business Alliance (community convener), Liberty, TruFund, LiftFund, NewCorp, etc.
4. Subsidize internships & apprenticeships – $40M: fund the New Orleans Youth Alliance , YouthForce NOLA and the Urban League of Louisiana to place and help subsidize apprenticeships, internships and other work-based learning experiences for ~20K young adults in high-pay sectors (e.g., energy)
5. Fund SFI program – $12M: fund the Student Freedom Initiative’s Income Contingent Alternative to Parent Plus to support ~120 Black STEM students per year forever at 3 HBCUs*
* Minority Serving Institutions / HBCUs being considered: Dillard University, Southern University – New Orleans and Xavier University of Louisiana
6. Scale career prep – ~$10M: scale the New Orleans Youth Alliance and YouthForce NOLA with 15-20 coaches over 5 years to equip ~20K young adults with skills for high-paying industries, job search & prep and subsidized transportation
Estimated impact (of all initiatives): ~2K additional college graduates and ~20K workers with high-paying wages to drive ~$1B in economic growth
7. Accelerate SCI’s digital access initiatives – up to $35M in donations or in-kind: partner with New Orleans’s Office of Information Technology & Innovation and Education SuperHighway to invest in setting up internet connections / hotspots, offering laptops/Chromebook and supporting adoption (through government subsidy technical assistance and digital literacy) to connect ~55K homes to high-speed internet in Greater New Orleans region
8. Raise community awareness & adoption of Emergency Broadband Benefit: increase door-to-door and community outreach in low-income neighborhoods to get households onto EBB to help connect ~55K unconnected households
Estimated impact (of all initiatives): 55K households connected to high-speed internet to unlock ~$1B in economic potential
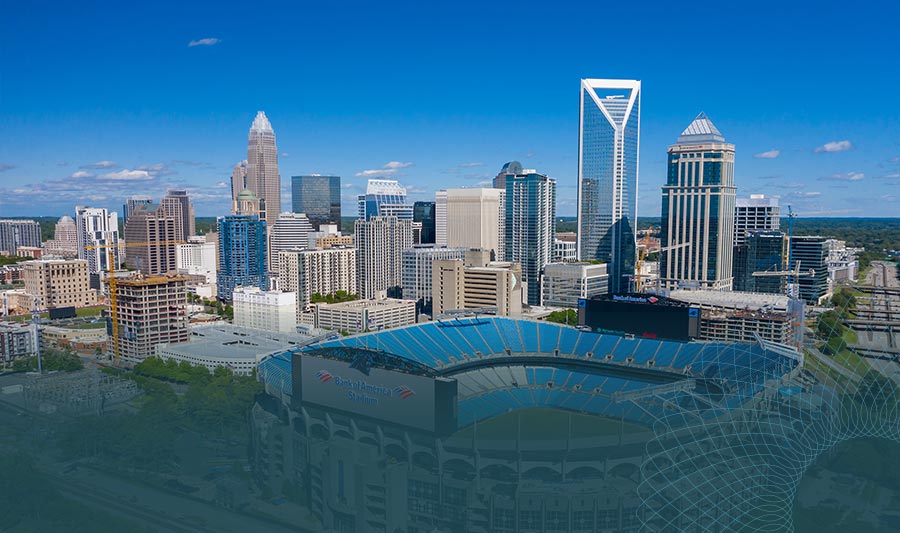
Charlotte, North Carolina
Lead community organization: Charlotte Regional Business Alliance
1. Offer in-kind FTEs: provide 2-5 in-kind FTEs to the Charlotte Regional Business Alliance (CRBA) over 5 years to convene corporate partners, assess their MBE spend, develop pipeline to increase MBE spend to 5-10%+
2. Offer technical assistance expertise: partner with the Charlotte Regional Business Alliance (CRBA) to advise/mentor ~200 MBEs on capital/loan access to help them scale from <$10M to $50M+
3. Commit to supplier diversity: increase MBE spend in Charlotte region to 5-10%+
Estimated impact (of all initiatives): 3x increase in MBE value & ~13K new jobs, boosting Black community’s net worth by ~$2B+
4. Fund modernization & capacity-building and provide in-kind subject matter experts – $30M : help 4-5 CDFIs/MDIs* over 5 years modernize their core systems, hire and train staff, expand marketing and standup SWAT team of experts to conduct needs diagnostic, implement tech solution & provide technical assistance; in-kind experts to also help build out the MBE ecosystem through CDFIs/MDIs, market CDFI/MDI offerings and programs and help draft final loan agreements to qualify borrowers between investment fund(s) and CDFIs/MDIs
* CDFIs/MDIs being considered (examples and not exhaustive): Security Federal Bank, Institute / North Carolina Community Development Initiative, Sequoyah Fund Inc, Self-Help Credit Union, BEFCOR, Aspire Community Capital, etc.
* In partnership with National Bankers Association and Appalachian Community Capital ; CDFIs/MDIs being considered include: Security Federal Bank, Institute / North Carolina Community Development Initiative, Sequoyah Fund Inc, etc.
5. Fund SFI program – up to $10M: fund the Student Freedom Initiative’s HELPS program to support ~1.5K+ students per year at HBCUs* with emergency expenses – e.g., unexpected health costs, late rent payments, etc.
* Minority Serving Institutions / HBCUs in Charlotte that are being considered: Johnson C. Smith University, Johnson & Wales University – Charlotte, Charlotte Christian College
6. Provide in-kind staff: offer 2-5 FTEs to the Charlotte Regional Business Alliance (CRBA) over 5 years to track Black-/Brown-executive representation, convene corporate partners to develop executive pipeline and hiring plans and support corporate partners to increase representation from ~10% to 30%+
Estimated impact (of all initiatives): 2.5K+ additional college graduates and 2.5K workers with high-paying wages to drive ~$0.2B in economic growth
7. Raise community awareness & adoption of Emergency Broadband Benefit: increase door-to-door and community outreach in low-income neighborhoods to get households onto EBB to help connect ~35K unconnected households
Estimated impact (of all initiatives): ~35K households get connected to high-speed internet to unlock ~$700M in economic potential for Charlotte
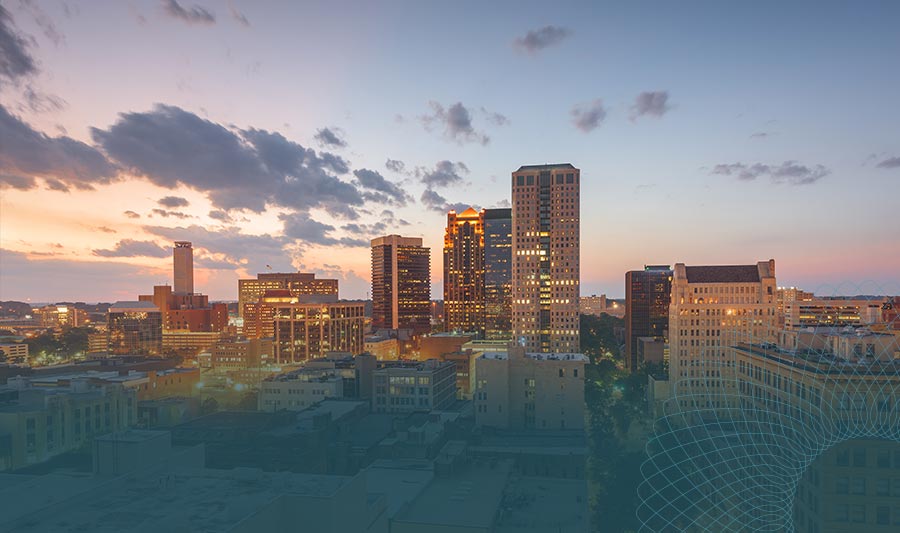
Birmingham, Alabama
Lead community organization: Prosper Birmingham
1. Fund startups and give access to investor network – $70M: grow the Prosper Health Tech Fund – powered by Gener8tor – and offer venture capital technical assistance to scale 50+ startups from <$1M to $5M+ in annual revenue; near-term priority is to secure $4M in venture investment by end of May 2022
2. Fund technical assistance – $25M: fund Prosper Birmingham , Magic City Match , and Birmingham Business Alliance to establish/expand business advisory programs, renovate and subsidize retail/office space for MBEs and scale coaches & support services (e.g., digital footprint, B2C platforms, accounting & bookkeeping, recruitment, etc.) to help 100+ MBEs scale from <$1M to $5M+ in annual revenue
Estimated impact (of all initiatives): 3x increase in annual MBE revenue & 8K+ new jobs, boosting Black community’s net worth by ~$2B+
3. Fund modernization & capacity-building and provide in-kind subject matter experts – $30M: help 4-5 CDFIs/MDIs* over 5 years modernize their core systems, hire and train staff, expand marketing and standup SWAT team of experts to conduct needs diagnostic, implement tech solution & provide technical assistance
* In partnership with National Bankers Association and Appalachian Community Capital ; CDFIs/MDIs being considered include: First Bancshares, Commonwealth National Bank, TruFund, Sabre Finance, Bronze Valley, etc.
4. Fund scholarships and hire coaches – ~$35M: scale Birmingham Promise fund to financially support 200-250 students per year over 4 years to increase college retention and graduation rates
5. Fund endowment – $2M: support 50 University of Alabama at Birmingham college students per year with housing to reduce their financial burden and increase college retention and graduation rates
Estimated impact (of all initiatives): 6.5K+ additional college graduates & 35K workers with high-paying wages to drive ~$1.2B in economic growth
6. Raise community awareness & adoption of Emergency Broadband Benefit: increase door-to-door and community outreach in low-income neighborhoods to get households onto EBB to help connect ~35K unconnected households
Estimated impact (of all initiatives): 48K households get connected to high-speed internet to unlock ~$700M in economic potential for Jefferson County

- DEALER LOG-IN
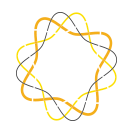
4 Ways STEM Education Preps Students for 21st-Century Careers
Do you know what a doctor, engineer and IT administrator all have in common?
You guessed it: a strong background in STEM education.
While many professionals are already pursuing a STEM career, it may be surprising to hear there’s still a huge gap that needs to be filled. According to the U.S. Bureau of Labor Statistics (BLS) 2019–29 employment projections , STEM jobs are expected to grow 8% by 2029, compared with 3.7% for all other occupations.
This is the main reason why STEM education is crucial in today’s educational setting. To keep up with advancements in STEM (science, technology, engineering and math), we need future generations to have a strong foundation in those areas.
Even if students decide to not pursue a career in STEM, STEM education is still a useful tool to prepare students for 21-century careers. Check out the top ways STEM education gives students the core skills they need to be successful in the modern workplace.
1. STEM education develops problem-solving skills.
Problem-solving is a must for a variety of different career paths but is even more important for STEM careers where solving problems is the entire purpose of the work. Through STEM education, students learn how to identify problems and think of possible solutions all while sharpening their critical thinking skills.
2. STEM encourages collaboration and teamwork.
In the real world, people are usually required to work together on projects and solve problems. With STEM education, students are introduced to collaborating with one another from an early age. As a result, these students end up thriving throughout their careers in team-oriented environments.
3. STEM promotes creativity and out-of-the-box thinking.
Without creativity and out-of-the-box thinking, there would be many everyday inventions that wouldn’t exist. Or, think of future inventions that could be brought to life with someone with a diverse education in STEM.
One of the many ways STEM teaches students to be innovative and creative is by making them think outside of the box. This is done by showing students different models so they get a range of ideas of how bringing together one idea might look, or by asking open-ended questions so students can come up with a solution on their own.
4. STEM ignites curiosity.
Another great skill students learn through STEM is curiosity. To be successful in a 21st-century career, people need to do more than just master the content. They need to look across disciplines and ask questions. They need to be innovative and learn from failures. With STEM education, students learn how to ask more intuitive questions, learn from mistakes and make educated decisions. Their passion may build, making them more likely to learn and even pursue a STEM career later on in life.
Incorporating STEM Education Into Your School Curriculum
As you can see, STEM education is a beneficial tool to help students thrive in 21-century careers, whether they pursue a STEM career or not. Integrating important STEM concepts into your school curriculum will turn students into tomorrow’s innovators, problem solvers and critical thinkers.
But how do you incorporate STEM education into your school curriculum? Well, it all starts by setting up learning spaces for success. At Diversified Spaces, we have a variety of classroom furniture that will help students build these valuable STEM skills. Reach out today to discover which type of furniture is right for your STEM classroom.
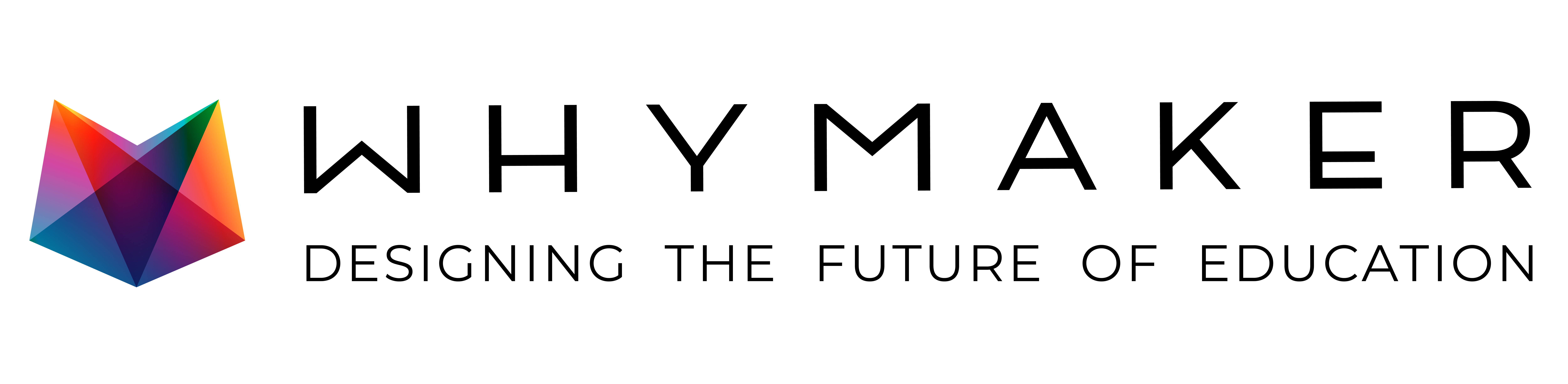
5 Ways To Successfully Teach STEM Education and Design Thinking
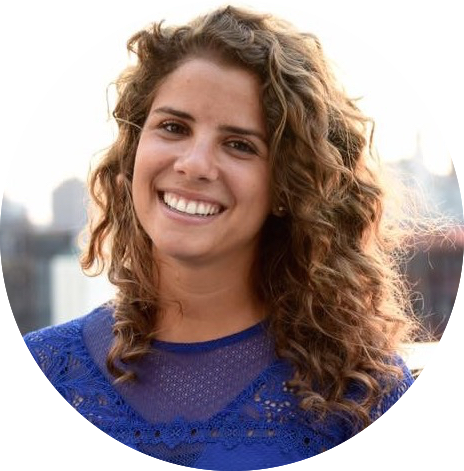
Kids are naturally excited about STEM. They enjoy hands-on learning and have an innate curiosity to understand what makes things work and how parts are connected to the whole. As educators, to be successful teachers of STEM, design thinking and project-based learning, it’s important for us to foster that excitement that our students exude. We, too, must be excited. Our students will feed off our energy and their love for all things STEM will grow.
First, What is STEM Education and Why Is It Important?
If students are not familiar with STEM learning (and some may not be), it can help to start with defining the term STEM as an acronym for Science, Technology, Engineering and Math. Then explain the importance of these cornerstone subjects (i.e., how they touch every area of our lives and offer real-life applications). This is an appealing concept to students who don’t see the point in studying science or math perhaps. Very practically, students can use what they learn from STEM to understand the world around them and prepare for their future careers.
In a recent article in The Harvard Gazette, Bridget Long, Dean of the Harvard Graduate School of Education, cited the U.S. Bureau of Labor Statistics, which shows employment in STEM occupations has grown 79% in the past three decades with STEM jobs projected to grow an additional 11% from 2020 to 2030. To be clear though, she adds “the importance of STEM education is about so much more than just jobs. STEM fields demand curious individuals eager to solve the world’s most pressing problems. The study of STEM subjects teaches critical-thinking skills, and instills a mindset that will help students find success across numerous areas and disciplines.“
What role does design thinking play in K-12 STEM education? Design thinking is a process for creative problem solving, using innovative ideas to come up with solutions for problems that may not be well-defined. Design thinking and STEM education go hand-in-hand to solve problems by focusing on the people for whom they are designing. Learn more about the WhyMaker 7-stage process for incorporating design thinking into K-12 STEM education.
Get Parents/Guardians on Board with STEM for Students
The role of parents or guardians is very important when fostering students’ excitement about STEM. Having enthusiastic partners in their children’s STEM education will contribute to a better experience for all. Share with parents what students are doing in class and how it improves students' learning such as practical knowledge, as well as innovative and critical thinking, problem-solving, and personal growth.
Inviting role models working in different STEM professions to speak to classes has been shown to encourage students and get them excited about their futures. Parents and guardians will be influenced positively by their children’s reactions to STEM professionals taking an interest in their education and supporting them.
Give parents activities to do with their children at home that connect to the STEM projects they are doing in class. Check out this list of unplugged activities families can do together at home.
Embrace the Benefits of STEM and Design Thinking
Here are 5 ways to set yourself up for success when teaching t STEM education:
- Provide a dedicated space for STEM learning – At WhyMaker, we have lots of resources and we are here to support you in creating an exciting Makerspace for teaching STEM. Here’s a guide for creating your own Makerspace.
- Offer hands-on learning experiences that encourage students to overcome challenges and solve real problems. Create learning goals and friendly competitions for team building. Read more about engaging students in hands-on learning.
- Do skill-building projects with students – teach them how to use and allow them to practice with materials and techniques like cardboard attachments , different types of adhesives and building sturdy triangles or a wheel and axle. These skill builders will allow students to have success and build confidence in their abilities.
- Enlist adults or high school students to mentor younger students – this not only benefits the younger students but provides a mentoring role for older students. Adult mentors may be STEM professionals/retirees or other interested volunteers. With support and mentoring, all students will benefit, even students who don’t feel they have an aptitude for STEM.
- Teach your students about careers in STEM – the workforce needs students with STEM backgrounds. Check out this post .
STEM and the Important of Educational Equity
Increasing access and opportunity in schools is vital and this includes equal access to STEM education.
A recent Pew Research Center article references a 2021 analysis of U.S. government data showing “Black and Hispanic workers remain underrepresented in STEM jobs compared with their share of the U.S. workforce.” Women are also vastly underrepresented in some STEM fields. Guess what helps? “The prospects for increasing diversity in the STEM workforce are closely tied to the educational system.”
According to Graduate School of Education Academic Dean Martin West in The Harvard Gazette article , more can be done to ensure students from all backgrounds are exposed to a wide variety of opportunities. Companies offering access to digital platforms and free online learning tools are attempting to bridge the gap. There is also a need for supporting teachers through increased funding.
At WhyMaker, we believe all students should be engaged in purposeful, project-based learning experiences. Check out our workshops that help schools build a culture of Maker Education.
Not ready for a workshop? Check out our teachers pay teachers page for some resources you can use in your classroom right now. Can’t afford our teacher pay teachers products? Send us an email and we will send the documents you are curious about in return for giving us feedback.
Latest Posts

Latest Articles
.png)
Finding Energy in Professional Development as an Educator in 2024
Gone are the days of simple content delivered in video format to watch on a loop before taking a simple quiz. Digital learning in 2024, thankfully, has gotten over the on-demand, individual, asynchronous, peer-less, or instructor-less learning modules. Instead, you can now find many new programs, peer-learning, collaborative networking, and impactful storytelling in a variety of personalize formats, such as social learning LMS platforms, podcasts, or hybrid options. In short, there is more direction now for you to direct your own professional development with increased flexibility and customization. And, the best news, is that many are now low-cost or even free.
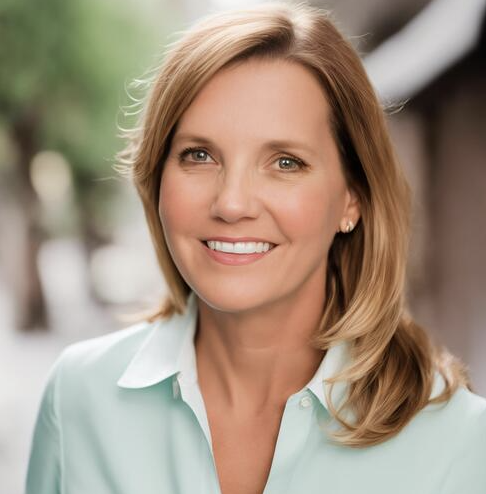
Classroom Essentials For Teachers On Amazon
Are you a teacher who loves being up-to-date and looking for classroom essentials so you can engagingly teach your students?
Celebrate CTE Month With Actionable Tools You Can Use in Your Classroom
February is national Career and Technical Education (CTE) month and is recognized nationally and regionally by educators, legislators and students.
How Teachers Build Confidence to Motivate Middle Schoolers in STEM

- Share article
Middle schoolers embody a messy no-man’s land: young enough to get excited by stickers and play-acting, but mature enough to crave autonomy and opportunities to improve their world.
Younger adolescents have shed much of their early-childhood curiosity and confidence that they can do anything. They’re hyper-focused on peers, social standing, and extracurriculars, right when the academic rigor of their math and science classes ratchets up.
Good luck getting this difficult-to-inspire bunch excited about the electromagnetic spectrum or fractional coefficients.
Sixth through eighth grade marks a shift in what motivates students, presenting a big challenge for science and math teachers.
“For many [middle school] students, their motivation will decline” starting in adolescence, said Emily Rosenzweig, an assistant professor of educational psychology at the University of Georgia who studies adolescent motivation in STEM subjects.
There are different reasons why this happens, Rosenzweig said, but it surfaces some new problems for middle school teachers: “A big one is competence perceptions: struggling to help students feel like they can learn in STEM,” she said.
Key to motivating this group academically, experts say, is giving them the chance to solve real problems in the adult world, while nurturing their confidence in their abilities and potential. Educators must also appeal to both sides of a middle schooler: the adult they are becoming and the child they very much still are.
In this developmental stage, kids want to know why they’re learning something and not just be told to do it, said Rosenzweig. That desire to understand the “why” is rooted in adolescents’ growing need for independence, she said.
What motivates middle school students?
Connecting what students are learning in STEM subjects to problems they might have to tackle in the real world is an important strategy for motivating this age group.
In Dani Boepple’s 8th grade science class at McDonald Middle School in Mesquite, Texas—located in the Dallas metro area—students learn how human activity in their land-locked city impacts ocean ecosystems.
Boepple teaches them that what goes down drains and runs off lawns at home and in school eventually ends up in the ocean. The same is true of litter, which makes its way into local streams and rivers.
Students then develop plans for action they can take in their homes and neighborhoods to reduce ocean pollution from chemicals and plastics.
“Their plans are really simple but effective,” Boepple said. “They will say, ‘I need to tell my parents to not spray pesticides on the garden and we need to pull weeds. Or I can help my neighborhood by cleaning up the trash that I see in the parking lot.’ It’s things they can actually do to make an impact.”
Projects like these tap into this current generation’s interest in fixing their world, she said.
Connecting things to the real world that they can relate to is a great way to engage them. [...] You don’t have to teach straight from the book.
Boepple also takes her students on virtual field trips to see the inner workings of NASA’s Johnson Space Center in Houston and the technology behind Amazon fulfillment facilities in different states. Students can see how what they’re learning now about the physics behind the movement of objects in our solar system, for example, can be applied to real careers. Hands-on experiments—such as using kinetic sand to make 3D models of topographic maps or mixing chemicals to create reactions—can also be a powerful motivator, said Boepple.
A big challenge to motivating her students, Boepple said, is that many are so afraid of failing that they would rather not try, something she attributes to students internalizing the message that they’re behind after the pandemic and a weakening focus on growth mindsets in their schools.
To help students get past their fear of failure, Boepple focuses on something outside the traditional science curriculum: trust.
“If they trust you, they are more willing to try,” Boepple said. “Just like if they like you, they are more willing to try. Like is a big thing, especially for middle school. They are not going to work for someone they don’t like.”
Boosting her students’ confidence is a focus for Kristyna Mosqueda-Rogers in her 5th and 6th grade math classes as well. Mosqueda-Rogers teaches at Carr Middle School in Hale Center, a rural community in northwest Texas. Her students struggle with a lack of belief in their capabilities, which leads to disengagement.
Her answer? Food trucks.

Mosqueda-Rogers’ 6th grade students are designing their own food trucks, tackling all the math that comes with launching and running a small business, from making decisions about menus and hours of operation to calculating the cost of food, fuel, and wages.
Students build shoebox-sized cardboard models of their food trucks and present their final projects in a classroom food truck festival.
Mosqueda-Rogers’ 5th graders engage in a similar exercise: They design their own zoos, including researching and calculating the size of the enclosures for different types of animals.
“It brings in a lot of the concepts that we teach into one realistic scenario,” she said. “We’re looking at decimals, geometry, finance. ”
While Mosqueda-Rogers has found project-based learning to be very motivating for her students, talking about STEM careers or big, societal problems that can be solved through the STEM disciplines can be a little too abstract for her 5th and 6th grade students. So, she said, “I have to do stuff that’s related to them right now.”
But there are many barriers to teaching students math and science from a problem-solving perspective, according to educators in a recent EdWeek Research Center poll.
There are other strategies for motivating this age group beyond project-based learning.
Teachers can find ways to give students more say in their education, said Rosenzweig, the psychologist, to help meet their developmental need for independence. For example, teachers can offer students a choice between an essay or a presentation for an assignment, or consult students in creating classroom rules.
In Boepple’s 8th grade science classroom, students can earn tickets for meeting academic goals to spend in the “college store” she has created. She stocks her store with apparel and accessories from different colleges that she buys second-hand or has received through donations. Students in her school are allowed to not wear their required uniforms as long as they are sporting clothing with college names on it, and they go wild for the items, Boepple said.
“That really helps motivate them because they can’t afford to go buy a nice college sweatshirt,” she said. “They are so grateful, and they wear them every day with pride.”
Both Boepple and Mosqueda-Rogers say that stickers that recognize small or big achievements—the currency of many elementary school classrooms—remain powerful motivators for middle school students too.
Motivation is multifaceted, and so are the ways to inspire it
Mosqueda-Rogers also believes that the example she sets, as a Latina math teacher , is helping motivate her students by challenging stereotypes about who can be good at math.
“I have so many little Hispanic girls who have a deeper love for [math] now, and they’re just growing tremendously because they’re seeing that it can be done by anyone,” she said.
Even if middle school students—especially the younger ones—aren’t thinking too deeply, yet, about what they want to do when they grow up, it’s important that they see it as plausible that they could join a STEM field, said John Dedeaux Davis Jr., a STEM education specialist at NASA. The U.S. space agency employs him to develop resources for educators to spark kids’ interest in STEM subjects, so NASA has the scientists it needs in the future.
“That connection is very important because we know early exposure to STEM can help drive people to those careers,” said Davis, a former science teacher who taught middle school in Chicago and Texas for 16 years before joining NASA. “Middle school is when students really start to form those ideas of what they might do as a career.”

When he was in the classroom, Davis encouraged his students to tap into the inner child they were quickly growing out of and play make believe—acting like a surgeon or astronaut. The idea, he said, was simply to get students to imagine themselves in these roles.
When it came time to dissect animals, Davis decorated his classroom like a lab or operating room and gave students white coats to wear so they could pretend to be scientists or doctors.
When the class studied space, Davis set up different stations around the classroom. For instance, he turned the underside of a table into a rocket cockpit. Students laid on their backs beneath it, similar to how professional astronauts position themselves during take off.
“Connecting things to the real world that they can relate to is a great way to engage them,” Davis said. “You don’t have to teach straight from the book.”
Some students may seem very difficult to get engaged in STEM. But educators should not give up on them too quickly, Rosenzweig recommends.
There’s a common misconception that motivation comes solely from within students, when really their environments do a lot to shape motivation—such as classrooms that give students more autonomy and opportunities to see the real world application of what they’re learning.
Another big misconception: If a student seems disinterested, then they’re probably not motivated to learn about STEM subjects.
“If a student seems to be not paying attention, seems to be kind of disengaged, it could be because that student doesn’t like the subject and doesn’t want do it,” Rosenzweig said. “But it could also be that the student has a more complex profile. Like, they really think this is important, but they’re struggling with their confidence for it. Or they feel like this is something they can do, but they were up late doing work for a different class.”
And even if a student doesn’t seem destined for a career in a STEM field, competency—and confidence—in STEM subjects is still important for them, too, Rosenzweig said.
The critical thinking and problem-solving skills developed in STEM subjects apply to virtually all other fields and pursuits. And you can never really know when or how a student might find their way into a STEM field. A student may not follow a straight trajectory from high school, to college, to a career in engineering, said Rosenzweig. They might find their STEM calling later in life.
“In my opinion, the more we can do to cultivate that interest and confidence early, so students continue to see it as for them and continue to develop the basic competencies that leave the door open to lots of paths that they might discover later, the better,” she said.
Coverage of problem solving and student motivation is supported in part by a grant from The Lemelson Foundation, at www.lemelson.org . Education Week retains sole editorial control over the content of this coverage.
Sign Up for The Savvy Principal
Edweek top school jobs.

Sign Up & Sign In

The Development, Testing, and Refinement of Eccles, Wigfield, and Colleagues’ Situated Expectancy-Value Model of Achievement Performance and Choice
- REVIEW ARTICLE
- Open access
- Published: 17 May 2024
- Volume 36 , article number 51 , ( 2024 )
Cite this article
You have full access to this open access article
- Jacquelynne S. Eccles 1 , 4 &
- Allan Wigfield ORCID: orcid.org/0000-0001-5195-7900 2 , 3
2 Altmetric
To address the seven guiding questions posed for authors of articles in this special issue, we begin by discussing the development (in the late 1970s-early 1980s) of Eccles’ expectancy-value theory of achievement choice (EEVT), a theory developed to explain the cultural phenomenon of why girls were less likely to participate in STEM courses and careers. We then discuss how we tested key predictions from the theory, notably how expectancies and values relate to achievement choices and performance and how socialization practices at home and in school influence them. Next, we discuss three main refinements: addressing developmental aspects of the theory, refining construct definitions, and renaming the theory situated expectancy value theory. We discuss reasons for that change, and their implications. To illustrate the theory’s practicality, we discuss intervention projects based in the model, and what next steps should be in SEVT-based intervention research. We close with suggestions for future research, emphasizing attaining consensus on how to measure the central constructs, expanding the model to capture better motivation of diverse groups, and the challenges of testing the increasingly complex predictions stemming from the model. Throughout the manuscript, we make suggestions for early career researchers to provide guidance for their own development of theories.
Similar content being viewed by others
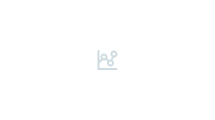
A New Look at Multiple Goal Pursuit: the Promise of a Person-Centered Approach
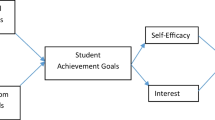
A multifactorial model of intrinsic / environmental motivators, personal traits and their combined influences on math performance in elementary school
Cross-Cultural Differences in Motivation
Avoid common mistakes on your manuscript.
We Footnote 1 are pleased to have the opportunity to discuss the development of what is now called situated expectancy-value theory (SEVT). We trace the early development of the model from Parsons et al.’s ( 1976 ) early work, discuss some of the studies testing different aspects of the model, describe theoretical refinements (notably the renaming to situated expectancy-value theory), and close with suggestions for future research. We organize our article around the seven questions Editor Greene asked us to answer.
What Inspired the Theory and What Observations and Phenomena Were You Trying to Describe, Understand, and/or Explain
During graduate school, two major forces influenced or inspired Eccles’ (then Parsons’) growing interest in both gender and individual differences in achievement motivation: (1) the excitement at UCLA in social cognitive influences on behavior, particularly those linked to attribution theory and the role of human agency in human development, and (2) the women’s movement. These two influences sparked Parsons’ interest in studying and understanding gendered life achievement-related choices. Working together with a terrific set of women graduate colleagues, Parsons coedited a special issue of the Journal of Social Issues entitled Sex roles: Persistence and Change (Ruble et al., 1976 ) that provided a new look at differences in women and men’s motivation and choice of different activities/occupations to pursue.
Developing the Theory
Parsons was Bernard Weiner’s Ph. D. student in the social psychology department at UCLA and also worked primarily with other social psychology students and faculty; thus, she was well versed in the field of social psychology. Weiner was Jack Atkinson’s student at the University of Michigan and so his research was on human motivation. Thus, Parsons had many opportunities to discuss the motivational theories of Atkinson, McClelland, and Weiner as well as the related work by social and developmental psychologist like Bandura, Kelley, and Maccoby. Interestingly, although Weiner was Atkinson’s student, he had gotten disillusioned with aspects of Atkinson’s ( 1957 ) theory, and the use of the thematic apperception test (TAT) as a primary measuring instrument (Weiner, personal communication, May 2019). He became interested in attribution theory and was developing his own version of it while Parsons was at UCLA. This sparked her interest in cognitive aspects of motivation, such as attributions and ability-expectancy beliefs (e.g., Parsons & Ruble, 1977 ), both of which are included in the model.
Parsons began to develop an expectancy-value theory based theory of choice during these projects in conversation with her social psychology peers. They (Parsons et al., 1976 ) published the first version of what was to become the model in the 1976 Journal of Social Issues mentioned above. Their initial version of the model had four boxes: cultural norms and expectations, current situational factors (e.g., discrimination), socialization in home and schools, and personal attitudes and values, and behaviors. Their focus was on gender differences in college women, so sample constructs in this preliminary model referred primarily to gender.
In 1973, Parsons moved to Smith College as a developmental psychology faculty member; this of course led her to be well-versed in developmental theories and research on motivation and other subjects. In her thinking on motivation, she was particularly influenced by Battle’s ( 1965 , 1966 ) and the Crandall’s’ (Crandall, 1969 ; Crandall et al., 1965 ) work on attainment value in relation to task choice. As a developmental psychologist interested in the first two decades of life, Parsons became increasingly interested in school and schooling and their differential effects on boys and girls. This brought her in contact with the field of educational psychology.
During her time at Smith, Parsons began having many conversations with students, notably Susan Goff, on the broader topic of incentive values and their impact on achievement choices. Parsons and Goff ( 1980 ) is the publication resulting from these conversations. This chapter was foundational for the development what would become the full model. Parsons and Goff critiqued Atkinson’s ( 1957 ) theory (arguably the dominant motivation theory of the time) in two primary ways. First, they noted that Atkinson’s model had not been particularly successful in predicting women’s outcomes, indicating that some re-conceptualization was in order. Second, they critiqued and expanded Atkinson’s view of incentive value. In the mathematical formulation of his model of resultant achievement motivation, Atkinson defined incentive value as 1–Ps (probability or expectancy for success). Parsons and Goff stated that this essentially eliminated incentive value as its own construct in the model and consequently researchers focused primarily on expectancies rather than value (see Wigfield & Eccles, 1992 , for full discussion of this issue). Rather than simply being an inverse of Ps, Parsons and Goff proposed that task or incentive value has different components and is a distinct from expectancies/probability of success. They provided the first discussion of different potential components of task value, mentioning attainment value, utility value, and whether rewards were seen as intrinsic or extrinsic. They also mentioned costs, particularly with respect to women’s choices regarding family and career. They also made the first mention of the idea that people have a hierarchy of values and that these can differ across individuals and groups, such as men and women.
Three and one-half years later, Eccles moved to The University of Michigan and began to elaborate the model with her graduate students. They did so to provide the theoretical rationale for a study of why females are less likely to go into mathematics than males; as discussed later, this study was funded by the National Institute of Education. In 1983, Parsons (now Eccles-Parsons) published the first chapter devoted to the model; her graduate students were co-authors Adler, Futterman, Goff, Kaczala, Meece, and Midgley. At that time, they referred to it as “a general model of achievement choice… which is most directly influenced by theories in which the constructs of expectancies and values are prominent” (p. 76). In later publications, Eccles began calling the model the Eccles et al. expectancy-value theory (EEVT; for example, see Eccles, 2005 ).
The model builds on Atkinson’s ( 1957 ) earlier work with its focus on expectancies and values. However, building on Parsons and Goff’s ( 1980 ) analysis, Eccles-Parsons et al.’s ( 1983 ) chapter defined the expectancy for success and task value constructs in much richer ways than Atkinson did, and linked them to a much broader array of more distal psychological, social, and cultural influences grounded in both developmental and feminist psychology. Their initial goal was to identify the major categories of social and psychological influences on people’s achievement-related behavioral choices that could aggregate across individuals to yield mean level differences at the population level in choices of females and males. Each of the boxes in the model in Fig. 1 stands for one major category of influence. They included specific examples of each category within each box. These examples were never intended to be exhaustive of the range of constructs that fit within each box, in part because this chapter focused heavily on gender differences. Second, they set out to specify the most probable connections between these categories as mechanisms for how socialization and enactment play out in individuals’ lives both over time and at each specific moment of action. Interestingly, given its impact in educational psychology, its roots and many of its early publications are in social, motivational, and developmental psychology—not educational psychology. The impact in educational psychology likely has occurred because of the focus on children and adolescents’ choices regarding different academic tasks to pursue, and the impact of schooling on the development of students’ expectancies and values.
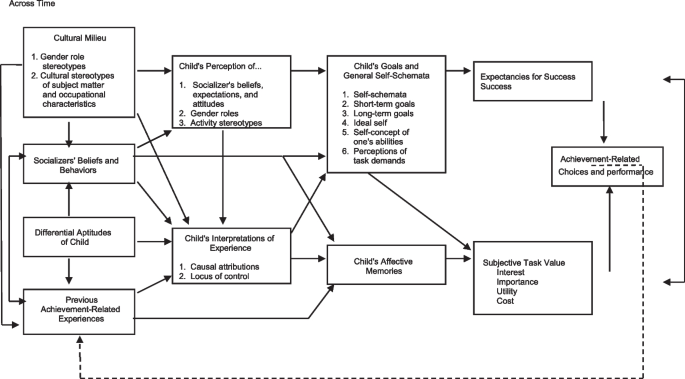
Eccles and colleagues’ expectancy-value theory (SEVT) of achievement performance and choice
Eccles-Parsons et al. ( 1983 ) divided their presentation of the model in two parts, the psychological component of the model and the socialization component. We discuss each in turn. Because the key belief and value constructs have been defined in numerous other publications, we define them briefly here. Figure 1 presents the current version of the model.
Psychological Component of the Model (Agency)
Eccles-Parsons et al. ( 1983 ) postulated that both achievement-related performance and task choice were influenced most directly and immediately by individuals’ relative expectations for success at and the subjective task value (STV) of the action chosen compared to expectations for success at and the STV of the readily available alternatives. They assumed that both expectancies and STVs themselves were influenced by individuals’ task-specific beliefs such as their self-concepts of various abilities, and their goals and self-schema, along with their affective memories for different achievement-related activities. Furthermore, they assumed that these beliefs, goals, and affective memories had been formed developmentally via individuals’ perceptions of other peoples’ attitudes and expectations for them, and by their own interpretations of their previous achievement outcomes. As discussed in more detail below, they predicted that these perceptions and interpretations had been influenced by a broad array of social and cultural factors, including socializers’ (especially parents and teachers) beliefs and behaviors, children’s prior achievement experiences and aptitudes, and the cultural milieu in which they lived.
Defining Performance Expectancies, Self-Concept of Abilities, and Subjective Task Values
Beginning with Eccles-Parsons et al. ( 1983 ) and moving to our own writings (e.g., Eccles & Wigfield, 1985 , 2023 ; Wigfield & Eccles, 2000 , 2020 ), we define expectancies for success as individuals’ estimates of how well they would do in the near or far future on any specific task or activity; for example, this or next year’s math class. In contrast, we define SCA as a more general stable estimate of one’s global but domain specific ability. In the model, Eccles-Parsons et al. postulated that task specific success expectations derived from the individuals’ domain specific SCAs. However, as measured, the items tend to factor together; we will use SCA to refer to both in the remainder of this article.
Eccles-Parsons et al. ( 1983 ) defined STVs with respect to the qualities of different achievement tasks and how those qualities might influence the individual’s desire to do the tasks (Eccles, 2005 ; Eccles-Parsons et al., 1983 ; Wigfield & Eccles, 1992 ). They proposed that one’s overall STV for any given activity or task is a function of four components: intrinsic value or enjoyment, utility value or usefulness of the task for other goals, attainment value, and perceived cost. As just noted, intrinsic value means finding a given task or activity enjoyable. Utility value, or usefulness, refers to how a task fits into an individual’s current or future plans, for instance, taking a math class to fulfill a requirement for a science degree. However, the activity also can reflect some important goals that the person holds quite deeply, such as attaining a certain occupation. Eccles-Parsons et al. ( 1983 ) defined attainment value as the importance of doing well on a given task. Over time, Eccles ( 2005 , 2009 ) connected attainment value more and more closely to the individual’s personal and social identities, so it became a broader construct than the importance of a task.
Eccles-Parsons et al. ( 1983 ) described cost in terms of cost/benefit ratio for different activities. If an activity “costs” too much, the individual will not do it (see also Eccles, 2005 ). They described different kinds or types of costs: individuals’ perceptions of how much effort they would need to exert to complete a task and whether it is worth doing so (effort cost), how much engaging in one activity means that other valued activities cannot be done (opportunity cost), and the emotional or psychological costs of pursuing the task, particularly the cost of failure (e.g., psychological cost). As discussed later, we and other researchers now have proposed other possible dimensions of cost.
We postulate that one’s overall STV for an activity is a function of a complex, not necessarily conscious, weighting scheme of the different aspects of task value just discussed. What is crucial in our view is that STV as a continuous higher order construct that could vary from very negative to very positive. Its level would derive from the algorithms used by the individual to aggregate across the multiple of more simple unidimensional constructs mentioned above.
Within Individual Influences on Expectancies, Ability Self-Concepts, and Subjective Task Values
The boxes in the middle of the model contain various psychological variables/constructs that impact individuals’ expectancies for success and STVs: for example, their goals and self-schemata, their perceptions of how hard the task or activity would be, their affect towards the activity based on previous experiences with similar activities, and their interpretations/understanding of how well they have been doing on the activity. Also critical are their understandings and interpretations of the messages they receive from different socializers. Eccles-Parsons et al. ( 1983 ) took these predictions from work in social psychology being done at the time regarding how perceptions and interpretations of experiences were the main pathways through which objective experiences in the real world influenced individuals’ beliefs and behaviors. As the field changed to emphasize the influences of culture, context, and the particular situations in which individuals find themselves, we have softened our stance on this point that it is primarily the individual as key.
Social Structure and Socialization Components of the Model
The left side of the model is focused on the social structure component of our agency and social structure general framework. Eccles-Parsons et al. ( 1983 ) originally limited this aspect of the model to two boxes, one specifying the larger cultural setting in which the individual is living (and emphasized gendered aspects of culture) and the other specifying the role of socializers. We now have elaborated what we think are the aspects of parents, teachers, and broader characteristics of school environment that impact expectancies and values; see Eccles ( 1993 ), Eccles and Wigfield ( 1985 ), Eccles et al. ( 1998 ), and Wigfield et al. ( 2006 ). Figure 2 illustrates such an elaboration regarding parental influences. Similarly, we characterize school influences as several different types of teacher in-class behaviors (e.g., differential use of praise and criticism across individuals and groups of individuals, classroom supportive climate, quality of teaching the subject matter, classroom support for student autonomy, and differential support for autonomy).
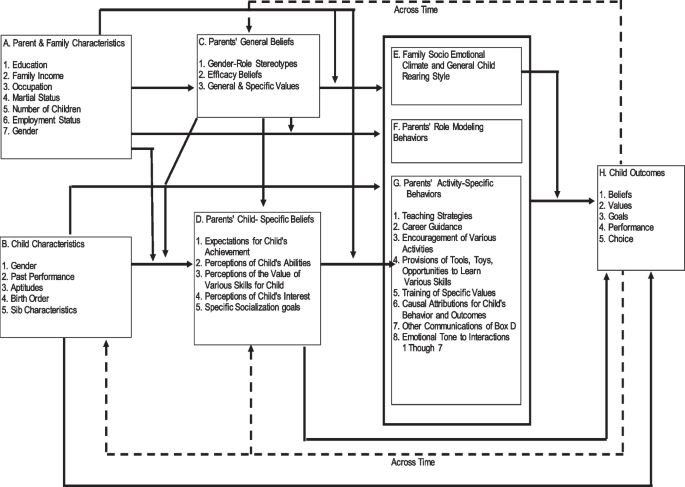
Model of parent socialization
In the reviews of the original submission of our manuscript, one reviewer asked for our perspective on whether theories should be well-formed at the beginning (because of previous research and/or a well-defined problem space that allows for that) or built as the research progresses. The reviewer viewed EEVT as well-formed at the beginning, and then tested and tweaked, rather than being built along the way, a view with which we for the most part agree. Our answer to the question of which approach to take is that it is situated! With well-defined problem spaces and a reasonable amount of research, theories can be built from the start. In other circumstances, the theory can be built as one goes along, as much design-based research does. Another part of the answer to this question is that one’s approach depends on how one views science and scientific progress. This would be an intriguing topic for fuller consideration in another article. Even with what we consider to be a relatively “fully formed” theory, we hope this section makes clear that there was a “developmental process” over several years before Eccles-Parsons and colleagues presented the first full version of the model in 1983.
Did Findings Lead to Some Changes in the Theory? Or Did Unexpected Findings Lead to New Directions?
The first study Footnote 2 testing certain predictions from the model was the NIE funded study mentioned earlier, that started in 1978. It was the first study of its kind conducted anywhere in the world, in that it used both survey and observational methods to study both social and psychological influences of gendered life choices and included students, parents, and teachers. As such it laid the groundwork for all subsequent research on the model.
We tested some key predictions from the model and explored many other questions. Regarding the proposed expectancy and value constructs themselves, Eccles and Wigfield ( 1995 ) demonstrated (using confirmatory factor analysis - CFA) that students’ expectancies for success factored separately from their subjective task values, providing support for the distinctions between the constructs. Students’ expectancies and values were positively correlated, suggesting that students value more the subjects at which they expect to do well and vice versa. The CFAs also showed that the three subjective task components measured in the study (interest, importance, and utility) each formed a separate factor. Thus, these components not only could be distinguished theoretically but also empirically. However, the items assessing expectancies for success and SCAs factored together, suggesting that although they can be distinguished theoretically, they are closely related empirically. Perhaps a more elaborate set of items would provide the differentiation.
We tested several predictions regarding expectancies, values, and different outcomes. Meece et al. ( 1990 ) examined the predictive links of students’ expectancies and STVs in math to their performance and intentions to continue taking math, looking at these relations over a 1-year period. They found that students’ expectancies for success were the strongest direct predictor of their subsequent math performance, and the importance they attached to math was the strongest direct predictor of their intentions to continue taking it in high school. Each had both direct and indirect effects on the outcome variables of performance and intentions.
Turning to gender differences, we will mention three key sets of findings. First, girls and boys themselves hold different views of their math, reading, and sports abilities that conform to American gender-role stereotypes and make different attributions for their performance that reinforce gendered stereotypes about abilities in these fields (Eccles et al., 1984 ; Eccles-Parsons et al., 1982c ). Second, math teachers interacted differently with girls and boys despite quite similar performances by these two groups of students (Eccles-Parsons et al., 1982b ; Heller & Parsons, 1981 ). For example, teachers called on boys more than girls, which reflected the fact that boys actually demanded more attention than the girls by raising their hands and shouting out answers. Third, parents provide their boy and girl children with different feedback regarding their math talents and performances (Eccles & Jacobs, 1986 ; Eccles-Parsons et al., 1982a ; Jacobs & Eccles, 1986 ). For example, parents were more likely to attribute their son’s math success to talent and their daughters’ math success to hard work.
These results supported our hypotheses regarding the origins and enactments of gendered stereotypes about math, reading and sports abilities in the USA (see Eccles, 1987 ). Furthermore, our studies demonstrated the power of a wholistic, psychological, and sociocultural model for guiding a comprehensive investigation of questions around broad topics like why are their fewer females entering fields based in STEM than males?
Pursuing Explanations for Unexpected Findings
Wigfield joined the research group in 1981 as a postdoctoral fellow; he was particularly interested in the development of motivation. The research team at Michigan next conducted two large-scale longitudinal studies to get at how children’s motivational beliefs and values change over time and understand at least some of the factors in the home and school environments that contribute to those changes.
It is important to say at this juncture that developmental questions were not initially a major focus for the development of the EEVT model. Furthermore, no one had generated specific predictions about what the course of the development of motivational beliefs and values would be, or which factors at home and school would most influence the changes. The developmental studies came about, first, because of an unexpected finding in the NIE funded study, and second, because Eccles and Wigfield became increasingly interested in the question of when the key motivational beliefs and values proposed in the model emerged. Both studies are examples of how a large, overarching framework can lead to studies that were not necessarily a part of the original plan for the model. They also reflect the importance of a project director who always was interested in answering new questions, the presence of a new post doc who was eager to take the team in new research directions, and an immediate and quickly growing bond between Eccles and Wigfield.
One of the major unexpected findings in the NIE project was a general cross-sectional downward shift in students’ motivational beliefs related specifically to math between grades 5 and 9. When we investigated this decline more carefully, we found that the differences was quite marked between grades 6 and 7, exactly the time when the students in our study were making the transition to junior high school. At the same time, Simmons and Blyth ( 1987 ) were finding a similar negative shift in other indicators of adolescent well-being occurring in conjunction with the junior high school transition.
What explains these developmental differences? Many scholars would be tempted to attribute these declines loosely in terms of some biological aspects of pubertal development linked to either hormonal changes or brain development. In contrast, drawing on the work by Simmons and Blyth ( 1987 ) and the theoretical work by Higgins and Eccles-Parsons ( 1983 ), we predicted that these declines could result from systematic negative changes in processes linked to the shift in educational social experiences associated with the transition to junior high school. We also expected there to changes at the level of the classroom that could undermine the students’ academic motivation.
Given our central goal, we designed a study to gather within-classroom data two times each year on the same students ( N = 3000) as they went through their last year in elementary school and then into and through their first year of junior high school. We chose this design because we wanted to be able to separate within and across year change in students’ expectancies, values, and other beliefs. As we had predicted, the strongest declines in students’ self-esteem and beliefs about ability/expectancies for success occurred between spring of the pre-transition year to fall of the post-transition year (Wigfield et al., 1991 ). Students “self-esteem recovered some during seventh grade but students” ability beliefs for math and English continued to decline, as did their liking of English and math. Boys reported higher self-esteem at each measurement point, and gender differences in beliefs about specific abilities followed gender stereotyping, just as we found in the NIE study.
To test our hypotheses regarding school level by classroom level by student level developmental processes, we assessed the extent to which changes in the students’ pre- and post-transition teachers’ beliefs explained the nature of the changes in the math motivational beliefs of our sample of students as they developed across these four waves of data. Some key findings were that junior high math teachers were more controlling, less trusting of their students, and used more social comparative grading strategies than did elementary school teachers. They were less likely to have confidence in their ability to teach all of their students and to be seen by their students as supportive and caring (Midgley et al., 1988 , 1989a , b ). In classrooms where these changes were strongest, it was more likely that students showed a decline in their math related expectancies for success, ability self-concepts, and subjective task values (Wigfield et al., 1991 ).
To explain these findings, Eccles and Midgley ( 1989 ) and Midgley et al. ( 1989a , b ) proposed the notion of stage-environment fit as key to healthy development. Students’ emotional adjustment and motivational beliefs had been undermined by a developmentally inappropriate shift in the educational environments to which they were exposed. This new notion was not a change in EEVT per se but represented an extension of it into a new area. It is perhaps analogous to the notion of a “mini-theory” within the larger theory along the lines that Ryan and Deci ( 2017 ) discuss in self-determination theory.
In part, based on our results and other work by researchers such as Simmons and Blyth ( 1987 ), the Carnegie Council on Adolescent Development ( 1989 ) produced a report on middle grades education that recommended that the structure of traditional junior high schools (which essentially mimicked high school structure) be changed into what are now called middle schools. The new school structure, at its best, is designed to produce stage-environment fit rather than misfit with respect to the developmental needs of early adolescents and their school structures. The interested reader can learn more about this change in Eccles’ ( 1993 ) discussion of how middle schools and traditional junior high schools vary in their structure, organization, and approach to the education of early adolescents. This recommendation was followed in many districts throughout the USA.
When Do Expectancies and Values Emerge During Childhood? The Childhood and Beyond (CAB) Study
Given our new developmental focus, we next decided to look at the early emergence of the key motivational beliefs and values in the model, developmental changes in the associations among these constructs, and their predictive role in explaining both individual and groups differences in achievement in academic, sports, and instrumental music domains. In order to carry out these goals, we first needed to create good measures of our psychological constructs that were appropriate for students across the kindergarten through twelfth grade years of schooling, which was a daunting task. The project had a cohort-sequential design in which we started measuring children’s their expectancies and subjective task values in different activity domains, and a variety of other constructs yearly, starting when children were in first, second, and fourth grades. Ultimately, we measured these variables until the oldest cohort was in twelfth grade, and then followed a subset of the sample until they were in their early 30s, in order to obtain information about their career choices. The sample was 95% white.
The key findings of this study were (1) children’s SCAs and STVs were differentiated from each other in the first grade and become more strongly differentiated with age (Eccles et al., 1993 ); (2) children’s SCAs and STVs formed distinct factors in each domain. Thus, children did not have a general belief representing their expectancies, or values, across domain, but differentiated beliefs and subjective values in each domain. In contrast, children’s anxieties did not differentiate by domain, suggesting that anxiety about one’s performance in skill-based domains reflects a more general response than do ability SCAs and STVs; (3) the strength of the association of ability self-concepts and expectancies for success, although always positive, become much stronger over age, as do the associations between expectancies, ability self-concepts and various aspects of subjective task values; (4) regarding change over time, early articles (Jacobs et al., 2002 ; Wigfield et al., 1997 ) reported decreases in students’ beliefs and STVs in the different domains studied. More recently researchers report many different patterns of change in students’ SCAs and STVs (e.g., Archambault et al., 2010 ; Gaspard et al., 2020 ; Musu-Gillette et al., 2014 ); and (5) gender differences in children’s SCAs and STVS generally corresponded to gender role stereotypes. Interestingly, however, in math, the gender differences disappeared over time.
Turning to hypothesized predictive links of children’s expectancies/ability beliefs and subjective task values to performance and choice, both Musu-Gillette et al. ( 2014 ) and Gaspard et al. ( 2020 ) investigated the long-term linkages of children’s beliefs and subjective task values to their course enrollments, choices, and career choice. Both reported different trajectories of change in children’s SCAs and STVs from first through twelfth grade. They found that membership in a different trajectory, or class, predicted later course enrollments, aspirations for different careers, and career choices. For example, children in a class characterized by strong declines in beliefs and subjective values for math were much less likely to be in a math-related career than children with more positive math beliefs and values.
How do we explain these changes? One way is to look at how change in children’s SCAs, STVs, and activity engagement is predicted by parents’ beliefs. Utilizing the elaborate CAB data obtained from parents, Simpkins et al. ( 2015 ) found that parents can have strong influences on their children’s continuing engagement in different domains even when child competence and participation are controlled. They also found that children’s competence beliefs often predicted change in parents’ beliefs about their children’s abilities, indicating the complex bi-directional relations between parent and child beliefs.
With respect to theoretical “advances,” Wigfield ( 1994 ) presented a developmental analysis of the theory, based in part on the results from CAB and also from other work on the development of motivation extant in the literature. Wigfield suggested that the emergence of the different components of children’s STVs likely would be in the order of interest value first, followed by attainment value and utility value. As noted earlier, attainment value has ties to identity, which develops during early adolescence. Young children might have an understanding of some things being important to them, but the full meaning of attainment value as expressed in Eccles ( 2005 , 2009 ) will emerge later in development. Similarly, understanding what skills will be useful to one later also takes some time to develop; it is unlikely that a 7-year-old will have a clear sense of which of the things she is learning ultimately will be useful to her later. Wigfield also proposed that both the relations between children’s SCAs and STVs, and their relations to outcomes, will become stronger over time, something that Wigfield et al.’s study ( 1997 ) demonstrated. They found that the correlations of children’s SCAs and STVs increased in strength across the childhood years, as did the relations between children’s belief and values and their teachers’ ratings of their performance. One way to visualize this is to think of the arrows in Figure 1 as being faint early in the school years, and gradually filling in over time.
To conclude this section, we built on some unexpected early findings regarding change over time in children’s SCAs and STVs to examine their development across the school years. This led us to consider the very early emergence of these beliefs and values, which in some ways can be considered to be addressing the question of when does the model and its predictive links emerge? Such questions were not necessarily there at the beginning when Eccles-Parsons et al. ( 1983 ) first posed the model, but became interesting due to early findings, and lead investigators’ curiosity regarding the early development of motivational beliefs and values. A lesson for early career researchers is that even when one is working within a particular and (if we can presume to say this) well-specified framework findings can be a surprise, and the addition of new members to a research team will result in different issues being addressed than might have been anticipated at the beginning. Both of these things make the research enterprise exciting rather than too cut and dried.
What Is the Current Status of the Theory? How Is It Similar and Different Than the Initial Theory You Created?
We will consider “status” in two ways. First is status in the sense of the “relative standing” of the theory. In that sense, we (immodestly) say that the theory remains one of the most prominent theories in the motivation field; evidence for this is its inclusion in both special issues of Contemporary Educational Psychology (one published in 2000, one in 2020) devoted to major motivation theories in educational psychology, and its inclusion in McInerney and colleagues edited volumes entitled “Big Theories of Motivation” (McInerney & Van Etten, 2004 ; Liem & McInerney, 2018 ). Eccles and Wigfield’s ( 2020 ) article on the change from expectancy value theory to situated expectancy value has been cited nearly 1400 times; Wigfield and Eccles’ article in the 2000 Contemporary Educational Psychology special issue over 10,000. We take this as evidence that the theory is still vibrant and provides theoretical framing for the work of variety of researchers from around the world.
However, in some comments on another article focused on the theory, a reader wondered about the continued viability of a 40 + -year-old model. To address this, Wigfield asked a non-random sample of junior colleagues why the theory still guided much research in the field (including their own). They mentioned a number of things about SEVT: (1) it is a clearly defined theory from which to draw testable research questions, (2) there are good measures available to assess the relevant constructs, (3) the emphasis on both the context/situation and the individual rather than one or the other, (4) the comprehensive nature of the theory that captures more than one piece of the motivation puzzle and connects many dots, and (5) easy to use and explain to educators; the terminology is accessible to those outside academia.
Turning to status in the sense of “current state “of the theory, several things have happened since Eccles-Parsons et al. ( 1983 ) first proposed the model. One is that some of the constructs have been reconceptualized and elaborated for theoretical and empirical reasons. We mention two examples from the right side of the model here, attainment value and cost, and two from the left side. As discussed earlier, Eccles ( 2005 , 2009 ) increasingly has connected attainment value to individual’s developing identities. She has written in particular about the salience of tasks with respect to one’s gender identity, and also other central aspects of one’s identity. When activities mesh with one’s identity, then they are more likely to be pursued and incorporated into one’s broader sense of self. To date, this newer conceptualization of attainment value has not been addressed fully; we return to this point below.
Eccles-Parsons et al. ( 1983 ) proposed three dimensions of cost: effort cost, loss of valued alternatives, and psychological cost of failure. For many years, cost was neglected by researchers basing their work in EEVT; Flake et al. ( 2015 ) called it the “forgotten” component of expectancy-value theory. That began to change with Battle and Wigfield’s ( 2003 ) study of college students’ perceptions of the costs of graduate school, and then especially since 2014 or so. Researchers have developed new measures of cost for students in grades five through college (Flake et al., 2015 ; Gaspard et al., 2019 ; Perez et al., 2014 ), and proposed adding additional aspects of cost (see Wigfield et al., 2017 , for a discussion of these possibilities as well as our own suggestions regarding the possible dimensions of cost). For example, Flake et al. distinguished effort cost related to the task and effort cost unrelated to the task (outside effort cost). They called psychological cost emotional cost. Gaspard et al. initially distinguished emotional cost from effort cost but due to their high correlation ultimately recommended combining them. More recently, Beymer et al. ( 2023 ) developed measures of both anticipated cost (students’ sense of how costly their classes were going to be) and experienced costs measured at the end of the semester. They found that those with higher anticipated cost tended to have higher experience cost. Interestingly, those with higher anticipated cost had better achievement outcomes. Song et al. ( 2023 ) examined the factor structure of emotional cost, psychological cost, and anxiety. They found that emotional and psychological costs factored separately, whereas emotional cost and anxiety often overlapped empirically. This left the researchers wondering how distinct the constructs are. Finally, Matthews and Wigfield (in press), in an article discussing ways to racialize aspects of SEVT, proposed that racialized opportunity cost (see Tabron & Venzant Chambers, 2019 ; Venzant Chambers, 2022 ) be added as a component of cost. It refers to students of color’s sense of what they must give up about themselves when attending primarily white schools. This new work has not been fully incorporated into the figure representing SEVT, in part because there is not yet consensus on what cost comprises. That is a task for future research.
Based on results showing that aspects of cost correlated more strongly with SCAs than it did with aspects of STV and also that the cost dimensions factored separately from the other aspects of value, Barron and Hulleman ( 2015 ) proposed that expectancy value theory be re-named expectancy value cost theory. In our own writings, we have argued that the evidence is not strong enough yet to add the C to EEVT (Eccles & Wigfield, 2020 ; Wigfield & Eccles, 2020 ). Space precludes a long discussion here, but to us the factor analytic evidence is not compelling because all the aspects of task value form separate factors. Research by Part et al. ( 2020 ) supports our view; their factor analyses of students’ responses to a questionnaire measuring college life science students’ expectancies, values, and cost perceptions revealed an overall task value with different components including cost. Muenks et al. ( 2023 ) measured college biochemistry students’ expectancies, values, and costs in a “high stakes” biochemistry course and found that models that subsumed cost under values and those that had cost as distinct from value both fit the data well provide some support for both views. Muenks et al. suggested that the results of different studies supporting cost as separate from value versus cost as part of task value might reflect differences in educational contexts; that is, they might reflect situational differences. Future research can continue to address this issue.
We mention two broad changes to the socialization side of the model. First, as noted earlier, we (and others) have written extensively about the particular kinds of experiences students have in school that can impact, either positively or negatively, their SCAs and STVs. These include different aspects of the quality of teacher-student relationships, grading practices, and school structural issues, among other things We have not systematically included these in our depiction of the model, that is something we are working on currently. With respect to parent socialization, Simpkins et al. ( 2015 ) findings supported some of the predictive links in the parent socialization model shown in Fig. 2 but not all of them; we are in the process of revising that figure as well to reflect these results (Eccles, Wigfield, & Simpkins, in preparation).
From EEVT to SEVT: Why the Change?
Year 2020 brought a major change to the theory, perhaps the most significant change made in the past 20 years. Eccles and Wigfield ( 2020 ) proposed changing the name from Eccles expectancy-value theory to situated expectancy-value theory. Why this change? There were several reasons. First, situative views of motivation that emphasize the importance and (in some cases) the primacy of the situation’ role on individuals’ in-the-moment motivations are increasingly prominent in the motivation field (see Nolen, 2020 ). In the model, we have always considered the situation’s impact on children’s developing motivation to be an important aspect of the model; indeed, even the “precursor” to the model in Parsons et al. ( 1976 ) includes the term “situational” in one of the boxes. We believe all aspects of the model are situative, even if the model in Fig. 1 does not fully capture that aspect of our work. For example, the right side of the model shows students’ expectancies and values predicting performance and choice. We assume (and actually always have assumed) that these decisions and the subsequent enactments are very situated. Each person will arrive at each decision point with their own set of available options that operate either in the moment or over longer time frames. They will only be familiar with a very limited subset of all possible behaviors and options. They will only have a small subset of the skills and resources that could be drawn on in enacting whatever decision they make. Both their own view and the view of those around them of what is going on and the available options will be limited. As a result, both their own hierarchy of SCAs and STVs and the hierarchies of those around them will be limited and very much tied to their current “situation.” So rather than being global and generic (which the representation in Fig. 1 can seem to be) people’s motivation beliefs, STVs, and decisions are situated and specific.
Having said that, it is important to acknowledge that in her commentary for the special issue of Contemporary Educational Psychology on major motivation theories, Nolen ( 2020 ) stated that situative approaches are based in different epistemological traditions than more psychologically based theories of motivation such as expectancy-value theory. They are rooted in Vygotsky’s ( 1962 ) work and other work that emphasizes sociocultural aspects of learning, rather than learning as an individual phenomenon. The differences in traditions have implications for how to study motivation—that one should not just look at individuals, but individuals’ part of a complex system, such as a classroom. In the commentary, she did not directly express concern with our decision to change the name of EEVT to SEVT, instead making the broader comments about the nature of situative approaches and how they differ from socio-cognitive ones. The second author has had conversations with her about these issues, and he and Nolen agree that collaborations of researchers from both perspectives can bridge the gaps between them, as long as the collaborators are “are clear-eyed about the fundamental differences and can work out how to work with them for mutual benefit” (Nolen, personal communication, March 24, 2024).
Another reason for the change was to emphasize more clearly the role of culture in the model. As can be seen in Figure 1 , the model begins in the far left with a box devoted to the sociocultural and historical nature of life. The model was developed initially to capture the cultural phenomenon of girls being less likely to go into advanced math and science courses, majors, and careers. Thus, the constructs in the Cultural Milieu box focus on gender. As we stated in 2020, we are quite open for researchers to propose and assess other aspects of the cultural milieu and examine them in relation to children’s developing SCAs and STVs. The model should not be viewed as complete as is but fluid and situation based. Some of the constructs added to the cultural milieu box could be relatively broad ones that affect most people in a given culture; others could be much more specific.
Matthews and Wigfield (in press) provide one example of this elaboration process. To capture the cultural milieu in which many Black children and adolescents in the USA grow up, they proposed a variety of cultural circumstances, including systemic racism, power differentials, continued and even increasing school segregation, and others that many Black children face. They also described socialization features of the school and home environments, some problematic and some protective, that characterize Black children’s circumstances. They proposed that these circumstances or influences very likely affect Black children’s developing expectancies and values and urged researchers to look more closely at them.
It is important to make clear that we view cultural influences occurring throughout the model; that is, we do not view them as exogenous influences that only have impact at the outset. Rather, they are infused in each box. Again, the problem once again is due to the depiction of the model rather than how we conceptualize it and have conceptualized it. We are working to create a new, more multifaceted depiction of the model that will capture better how we see individuals’ expectancies and values being part of the particular situations they are in, and influenced by larger, systemic aspects of the cultures they are in. We anticipate it being more “Bronfenbrenner ( 1986 ) like,” in the sense of viewing the development of expectancies and values being enmeshed in and influenced by different levels of the environments and cultures in which they live. The new version of the model will be elaborated in our forthcoming book (Eccles, Wigfield, & Simpkins, in preparation).
We also stress the fact that proximal socializers (i.e., all of the proximally located individuals that make up each individual’s lifetime and space) are directly influenced both by their sociocultural context and the characteristics of the focal individual. Thus, for example, we hypothesized that parents would respond to their children differently as the children become older and that these changing responses will be influenced by socioculturally derived notions of the age appropriateness of the specific goals they might have at any given age as well as the age appropriateness of the characteristics and behaviors of the focal child (Eccles, 1993 ; Simpkins et al., 2015 ). The same would be true for the sex of the focal child, the race, ethnicity, nationality, and religion of the focal child, the sexual, racial, ethnic, religious identity of the child, etc. Furthermore, and tied to the point about the situated nature of the model, we assume that these processes accumulate over time to produce individuals who are uniquely positioned due to their own unique histories, memories, endowments, and their quite specific location in time and space to deal with their set of behavioral options at any one point in time. Like Nolen ( 2020 ), we agree that context is a changing system that includes all of the participants. Thus, individuals both learn from and co-create contexts as they participant in them.
The name change already is having an impact on researchers’ examination of students’ developing SCAs and STVs. Since the change there have been several symposia presented at conferences like AERA and APA that use SEVT rather than EVT. The researchers doing this work measure things more specifically, look at variation over the short term and longer term, and otherwise try to take seriously the idea that students’ SCAs and STVs are situationally bound, and fluid. To date, however, this work has not undertaken a careful analysis of the situations in which the studies have been done, other than to note the particular situation (e.g., introductory college physics classes, advanced college biology classes). To capture more fully a situative perspective, the linkages between characteristics of the situation and the psychological belief and values being measured will need to be studied more thoroughly. We urge researchers basing their work in SEVT also work with researchers taking a situative approach ala Nolen ( 2020 ).
To conclude this section with some broad recommendations regarding theory development, we suggest that theory developers be sensitive to research findings that either do support predictions or provide nuance to those predictions to be sure that the theory captures both the overall picture and subtleties in it. We also think that when there is good reason to do so changing or elaborating the nature of a given construct in the model, either based on research findings or due to theoretical considerations is a good way to keep even an “older” theory current. Although we have admiration for Bandura’s ( 1977 , 1997 ) consistent definition of self-efficacy, constructs like attainment value and cost are still “works in progress” and so changing definitions (and measurement) illustrate progress. Moving beyond individual constructs to the theory as a whole changing the name of a theory to reflect developments in the field is (potentially at least) also a way to keep it current. Adding the single word “situated” to expectancy value theory seems to be having that effect.
Finally, it perhaps is worth commenting on “what can be tested” in this theory? We have heard suggestions that because of its size and scope, the theory is untestable. We agree that trying to test all of the proposed links in the model in one study is not something that would be possible. However, by validating constructs and testing various sets of predictive links in different parts of the model gradually, we can determine which parts of the overall theory have the most support and which either have not been supported, or not yet assessed. This perhaps is analogous to Ryan and Deci’s ( 2017 ) comments about building support for self-determination theory “brick by brick.”
What Are the Contributions of This Theory? How Has It Benefitted Educational Psychology Research, Practice, and/or Policy?
We noted earlier that the theory has guided and continues to guide a great deal of research on the development of students’ expectancies and subjective task values and their impact on decision making of different kinds. This is one way the theory has benefitted educational psychology research. With respect to educational policy, the broadest impact of our research is on the decisions made by many school systems in the 1990s to create middle schools that (when done right) better meet early adolescents’ developmental characteristics compared to traditional junior high schools. Another impact on educational practice is the intervention work based in the theory; we discuss that next.
Researchers have conducted numerous interventions to enhance individuals’ STVs, beginning with Hulleman and Harackiewicz’s ( 2009 ) study showing that a utility value intervention enhanced high school students’ interest in science and also their performance. Harackiewicz and colleagues subsequently have shown that utility value interventions positively influence other motivational beliefs and values, choices to continue taking courses in the area of the intervention, and grades, both in the short-term and long-term STVs; they have done these interventions with the students themselves and also their parents (e.g., Harackiewicz et al., 2012 ; see Harackiewicz & Priniski, 2018 ; Rosenzweig & Wigfield, 2016 ; Rosenzweig et al., 2022 ). Gaspard et al. ( 2015 ) have done successful utility value interventions focused on increasing the utility value of math for German high school students; interestingly, however, results of a recent replication study were not quite as strong (Gaspard et al., 2019 ).
Harackiewicz et al. ( 2015 ) implemented a utility value intervention in different groups of college students, including both first-generation and underrepresented minority students. Intervention group students’ grades improved more than did the control group students. Most importantly, the intervention was particular successful in improving first-generation-underrepresented students’ performance, meaning that it reduced the achievement gap between these students and majority students. Thus, these interventions may be particularly effective for groups who need them the most. Second, Harackiewicz and her colleagues have shown that these brief utility value interventions have both short- (within a semester) and longer-term effects such subsequent course choice, academic major choice, and career aspirations in college, (e.g., Asher et al., 2023 ; Hecht et al., 2019b ). Asher et al. ( 2023 ) found (in a large scale RCT study) that their utility value intervention implemented in a gateway chemistry course improved college students’ persistence in STEM fields 2.5 years later, a rather remarkable finding. Importantly, and as was the case Harackiewicz et al. ( 2015 ), effects were stronger for the underrepresented minority students in the sample.
Hecht et al. ( 2019a ) proposed several processes or mechanisms by which these long-term effects occur. They stated that recursive processes, or feedback loops where (for example) increased valuing of a course leads students to work harder in it, leading to further increases in the course’s value, and so on, can lead to long-term effects. Others include (1) nonrecursive processes where increases in task value promote positive change in other motivational beliefs such as ASCs or ESs, making the individual more likely to succeed in the class and take additional courses; (2) “trigger and channel” processes by which increased value for a subject area increases the degree to which students take advantage of existing opportunities to continue in that area (e.g., available classes in the subject, available majors); and (3) learning “habits of mind” that are conducive to succeeding in different classes. They are just beginning to disentangle how these processes separately and jointly help us understand the long-term success of utility value interventions.
One interesting aspect of this work to us was the choice to focus on utility value as the aspect of task value on which to intervene. Harackiewicz et al. ( 2014 ) posited that utility value is the most malleable of the task value components, and so most likely to change during interventions. Although this is possible, we believe other aspects of individuals’ task values can be enhanced by interventions, so suggest researchers extend the utility value intervention work to other aspects of STVs. In an important step in this direction, Rosenzweig et al. ( 2020 ) showed that an intervention designed to reduce college students’ perceptions of the cost of physics was as effective as a utility value intervention in increasing the students’ performance in physics. We also believe attainment value–based interventions should be developed. These could be designed to provide students with information on the link between potential associated job characteristics with the students’ goals and identities. Another focus would be how STEM provide opportunities to help others and work/collaborate with people as ways of attracting women into STEM fields, as Harackiewicz et al. ( 2012 ) did in their intervention with parents.
Rosenzweig et al. ( 2022 ) provide detailed suggestions on how to develop interventions focused on the other aspects of task value, as well as interventions designed to enhance both expectancies and different aspects of subjective task value. They address questions such as which are the most feasible aspects of task value on which to intervene, and also how and when it is worthwhile to intervene on multiple constructs including expectancies. In line with the new “situated” aspect of the theory, Rosenzweig et al. noted that intervention researchers should attend carefully to the characteristics and academic needs of the groups of students on whom they are intervening. Students struggling in school likely need interventions to boost that they can do the tasks along with boosting their sense of the utility of the task for them. Rosenzweig and colleagues’ recent work looking at students’ reasons for either dropping out of STEM fields or switching from one STEM field to another provide fertile ground for researchers to understand the challenges students face in different subjects. This fuller understanding should clearly impact both intervention design and success.
What Are the Virtues of the Theory?
Greene ( 2022 ) presented a variety of possible internal and external virtues of various theories. Internal virtues are specific to the theory itself; external ones focus on the theory in relation to other theories. We are somewhat selective in the amount of detail we provide on each of the virtues, providing more detail on the ones we think are most relevant to SEVT.
Beginning with scope , current motivation theories in educational psychology vary in their scope in a number of ways, from number of constructs to number of areas covered to number of links between constructs and the processes by which the constructs are connected, among others. Currently, in the field, there are arguably five major theories: attribution theory, goal orientation theory, self-determination theory, situated expectancy value theory, and social cognitive theory (to focus on the major theories presented in the 2020 special issue of Contemporary Educational Psychology ) There are other theories or approaches that have emerged; Nolen ( 2020 ) calls her (and others’) situative views on motivation an approach rather than a theory. Complexity theory/complex dynamic system approaches (e.g., Hilpert & Marchand, 2018 ; Kaplan & Garner, 2020 ) are taking shape and challenging “traditional” linear models but so far have emphasized the complexity of motivation rather than focusing on particular well-researched constructs and links among them. The most fully developed model taking this perspective arguably is Kaplan and Garner’s (2017) dynamic system model of role identity. It is not a theory of motivation per se and space precludes full discussion of it; we thought it important to mention because of the criticisms researchers taking a complexity perspective aim at the five theories just mentioned. Moving forward it will be interesting to see whether there is integration of these perspectives. We believe that SEVT should be considered a complex and dynamic approach; Kaplan, Garner, and the second author currently are engaging in conversations about this to determine the extent of our agreement about this.
Returning to the five theories just mentioned, arguably self-determination theory and SEVT are the broadest in scope. Other theories, such as attribution theory and goal orientation theory, focus on fewer constructs and so potentially could be incorporated into theories broader in scope. Ryan and Deci ( 2020 ) posited that self-determination theory could be the all-encompassing theory, and they have incorporated goal theory into SDT. In SEVT, attributions are contained in the Interpretations of Experience box and goals in the self-schemata box, so it has the potential to be a more encompassing theory as well. Theories sometimes speak to different issues so we do not want to claim necessarily that SEVT could be the all-encompassing theory, but clearly its scope is broad with respect to constructs included, links among them, influences on them, and processes discussed.
Regarding unification , as we noted in the outset, Eccles-Parsons et al. ( 1983 ) worked to develop a unified approach by (1) taking constructs and postulates from different areas of psychology, particularly social, developmental, and educational psychology; (2) including a broad array of motivation constructs from different kinds of motivation theories, notably “historic” expectancy-value theory, attribution theory (the interpretations of experience box in Figure 1 ), social cognitive theory (the construct of expectancies), achievement emotions, goal theories, and identity theories. As can be seen in Figure 2 , there are a broad array of parent socialization variables included. We noted at various places in this article that we also have written extensivity about a variety of school socialization practices and their impact (while acknowledging that those have not all been included in the graphic depictions of the model yet; and (3) studying a variety of achievement domains or areas to determine how broadly the predictive links could be obtained.
Is the theory parsimonious? Perhaps we should ask if parsimony is really a virtue? There appears to be some debate about that in the field. We noted earlier relatively new work in the motivation field focused on the complexity of motivation and how it is influenced by many different aspects of day-to-day practices in classrooms. Proponents of these approaches (e.g., Hilpert & Marchand, 2018 ; Kaplan & Garner, 2020 ) are critical of more “traditional” social cognitively, post-positivistic based theoretical approaches including EEVT/SEVT as being too linear, deterministic, and simplistic (read parsimonious?) in their depiction of motivation. Perhaps in a post post-positive world, parsimony is no longer considered a desirable feature of a good theory.
Turning to plausibility and the related virtue of accuracy , we believe the evidence summarized in this article and presented in the literally hundreds of articles that have utilized EEVT/SEVT as their theoretical base provide strong evidence for the plausibility of the theory. We acknowledge that we have not reviewed that evidence here; reviews can be found in Eccles and Wigfield ( 2020 , 2023 ), Wigfield and Eccles ( 2020 ), and Wigfield et al. ( 2016 ), and elsewhere. The constructs and their links are prima facie plausible, and the evidence provided by the various studies provides a solid foundation of support for the constructs and premises in the theory. In other words, the evidence makes a good case for the theory’s accuracy.
We think the theory has been and will continue to be quite fruitful in all the ways listed in Greene’s ( 2022 ) Table 1. We and other researchers continuously develop new research questions and hypotheses based in the theory. As Eccles and Wigfield ( 2020 ) discussed, the current version of the theory as presented in Figure 1 is meant to be representative of constructs and linkages covered in the theory rather being an exhaustive set. So, the theory is expandable to investigate other research questions and hypotheses. Finally, in terms of practical applications, the extensive number of intervention studies guided by the theory arguably make it the current motivation theory with, if not the most, certainly many practical applications. The intervention research has influenced educational practices at different levels of education. We believe these points apply equally well to the practicality point in the list of external criteria.
With respect to internal consistency/coherence in our view, there is little that is contradictory about the phenomena in the theory. This is because of its careful development from the different fields noted above, as well as its basis in long-standing theories in the motivation field. Eccles-Parsons and colleagues purposely designed the theory to be internally consistent. Furthermore, the research done testing various key predictive links in the theory has provided good support for them; results have not been contradictory in any sense of that term.
The mechanisms behind the constructs and relations expressed in the theory are a work in progress to some degree. One way that mechanisms are depicted is the arrows linking various constructs. As Eccles and Wigfield ( 2020 ) stated, “The arrows represent hypothesized processes and links that play out over time as well as within smaller units of time when specific task choices are being made” (101859). Arrows of course do not themselves indicate what those mechanisms or processes are, but we have written about them in other places (e.g., Eccles, 2005 , 2009 ; Wigfield et al., 2016 , Wigfield et al., 2017 ). One set of mechanisms or processes are how individuals process information they receive about their performance and how that impacts their developing expectancies and values. Examples of such processes are temporal comparisons where people judge how they are doing now compared to how they were doing before, social comparisons or how we compare ourselves to others, and dimensional comparisons or comparisons of our performance in one domain vs another (see Wigfield et al., 2020 for discussion). Other processes are less well understood; Eccles and Wigfield ( 2020 and elsewhere) have written about how we do not have a full understanding of how individuals weigh different information they have to determine how much they value an activity, or how they form their hierarchies of both expectancies and values. In the socialization area, we have noted in different places in this article how teachers and parents (and other socializers) impact children’s developing expectancies and values through many different aspects of their relationships, along with structural issues in the home and school.
A particularly important issue to continue to address regarding these mechanisms is how their influence change over time, both with respect to how children of different ages understand them, as well as how mechanisms such as the quality of teacher-student relations themselves change over time. Then of course there is variation in things like teacher-student relations at a given age or grade level. Clearly these mechanisms are complex and multifaceted, and greatly influenced by the situations in which they occur.
We will group testability and specificity together. On the one hand, the model has been critiqued as being untestable due to its size and scope. As noted earlier, we agree that the full model never will be able to be assessed all at once as it is just too big and complex to do so. Having said that we think there are many ways to create meaningful sets of constructs in the model and examine connections across them to test various specific predictions in the model, from how expectancies and values predict performance and choice to how parents’ messages about children’s ability impact their developing expectancies and values. That is, although the model is large, the constructs in it are (for the most part) specific enough that measures can be and have been developed, allowing for testing of different predictions. A great deal of work has been done around the world to examine various predictions from, and we are pleased that the model continues to stimulate much research.
Turning to the other two external criteria ( analogy and practicality ), there are many points of agreement between SEVT and other theories. Social cognitive theory focuses on how self-efficacy predicts performance and choice, as does SEVT. Attribution theory has to do with the explanations individuals give for their outcomes, and such interpretations are a key part of the information students use to form their expectancies and values. Goals and goal orientations are also key constructs in the theory; one of the boxes is labeled “Goals and Self Schemata.” Eccles and Midgley ( 1989 ) and other have written about the importance of support for autonomy and structure in the development of motivation, key tenets of SDT. The list goes on.
Having said that it is interesting that there have not been direct tests of one theory vs. another, for the most part, to determine which might provide the best explanation of different outcomes. Why is that? Possibly because extant theories do not contain enough similar constructs to pit one against the other. Another is that measures developed within a given theory often are at different levels of specificity. For instance, many measures in self-determination theory are domain general; those based in other theories are more specific. Anderman ( 2020 ) has questioned whether we need all the current theories; moving forward this is an important issue to address and so we hope more direct tests can be made. An example we can mention is that our work has shown that both expectancies and subjective task values are important predictors of outcomes, rather than expectancies, as social cognitive theory would predict.
With respect to practicality , we think the intervention work based in the theory already is having an impact on how teaching is done at a variety of institutions across the county, both post-secondary and k-12. The intervention researchers are working with instructors to change their teaching practices in order to emphasize the utility value of what their students are learning, to give just one example. In that sense, the theory is useful to society.
What Do You See as Some Promising “Next Steps” or “Open Questions” for Future Development of the Theory?
There are a myriad of possible next steps for the theory and research based in it, and researchers are generating new topics frequently; an example is the 2023 SEVT-based symposium held at APA in which authors presented papers focused more on the situated nature of expectancies and subjective task values, their ties to self-regulated learning, and their function in different groups. Eccles and Wigfield ( 2020 ) provided a number of suggestions, such as investigating more fully the interplay of the different aspects of task in determining overall subjective task value and the factors influencing the formation of STV and ASC hierarchies. We think the analyses to address these issues will of necessity be quite complex and agree with Nagengast and Trautwein’s ( 2023 ) view that theory and method need to be closer in sync with each other for the field to advance in ways that help understand the complexity among these relations.
Speaking of methods, one issue that needs addressing moving forward is how best to measure expectancies, values, and many of the other constructs in the model. We point to Pajares’ ( 1996 ) seminal article on measuring self-efficacy and how the disparate ways of measuring it left it quite uncertain what was being measured in any given study purportedly studying self-efficacy. We hope this will not be such a big issue for SEVT; however, there are issues to address. We address three. Eccles and Wigfield developed measures of the major constructs many years ago; they had and have good psychometric properties but also have certain limits. One mentioned earlier is that measures of attainment value we developed focus on importance only and have not brought in the identity aspects of attainment Eccles has discussed in greater detail more recently. There are opportunities for researchers focused on identity and SEVT-based researchers to work together to develop such measures. We mentioned earlier the growing body of work on cost and noted some of the new measures of it. An important challenge for those interested in studying cost is that these measures look quite different even though they are measuring purportedly the same construct. We think it crucial for researchers studying cost to come together to try to agree on what its components are and how best to measure them. Last, one of the ways researchers taking the new “situated” approach is to give measures to study participants more frequently, even as much as once a week. Such measures by necessity must be brief in order to avoid “scale fatigue” on the part of the participants. Yet are such measures analogous to the longer measures used?
SEVT has been the theoretical basis for much work on gender differences in motivation and an increasing amount of work on ethnic group differences in motivation (e.g., Diemer et al., 2016 ; Peck et al., 2014 ), but more work needs to be done on how culture, ethnicity, gender, and (more importantly) their interactions impact the development of individuals’ expectancies and values (see Tonks et al., 2018 , and Wigfield & Gladstone, 2019 , for discussion of culture and ethnicity’s impact on the development of children’s expectancies and values). When studying ethnicity researchers basing their work in SEVT should address how experiences of racism, discrimination, and oppression influence children’s developing SCAs and STVs. Kumar et al. ( 2018 ) note that most achievement motivation theories (including EVT) have not attended nearly enough to the impact of these powerful forces on students’ motivation. As noted earlier, Matthews and Wigfield (in press) proposed ways to racialize certain aspects of the model, notably the cultural milieu box and socialization box to understand better the development of Black children’s motivational beliefs and values. They connect SEVT both to critical race theory (Ladson-Billings & Tate, 1995 and Neblett et al. ( 2021 ) racial socialization model that describes ways Black parents can help their children deal with experiences of racism and discrimination, and instill a strong sense of racial identity, something that has been shown to relate to Black students’ achievement in school. Their article is the first attempt to connect systematically these discrete bodies of work as a way to better understand the development of Black students’ motivational beliefs and values.
With respect to the socialization aspect of the model, researchers have focused primarily on the impact of different parenting practice and school environmental factors on children’s developing STVs and ASCs. Many other influences need attention. Building on this point, we think it is time to expand the influences contained in the different socialization focused boxes to develop these ideas more formally. As with the issues noted above, testing these influences in sophisticated ways will mean more situated and frequent measures of the constructs, and complex modeling analyses (among other things) to examine relations among them. In these and other ways, we think SEVT and dynamic complex system approaches have much in common and look forward to conversations about their synergy.
To conclude, SEVT remains vibrant, increasingly complex, and relevant to understanding choices individuals make and their performance on different activities. Yet there are many ways it can be expanded, and many questions yet to address. We looks forward to the next generation of research based in it.
When talking about work Eccles and Wigfield did together we use the pronoun “we,” or more generally, use first person. When talking about EVT work we did not do together we will use our names. Note that Jacque Eccles initially was J. E. Parsons, then J. Eccles-Parsons, and now J. S. Eccles.
Because this article is on theory development, we focus on our own longitudinal studies designed to test the model. Research from around the world has confirmed both the “structure” of the constructs and the predictive links of SCAs and STVs to performance and choice (see Eccles & Wigfield, 2020 , 2023 ; Wigfield & Eccles, 2020 ; Wigfield et al., 2016 for review).
Anderman, E. M. (2020). Motivation theory in the 21st century: Balancing precision and utility. Contemporary Educational Psychology, 61 , 101864
Article Google Scholar
Archambault, I., Eccles, J. S., & Vida, M. N. (2010). Ability self-concepts and subjective value in literacy: Joint trajectories from grades 1–12. Journal of Educational Psychology, 102 (4), 804–816. https://doi.org/10.1037/a0021075
Asher, M. W., Harackiewicz, J. M., Beymer, P. B., Hecht, C. A., Lamont, L. B., Else-Quest, N. M., Priniski, S. J., Thomas, J. B., Hyde, J. S., & Smith, J. L. (2023). Utility-value intervention promotes persistence and diversity in STEM. Psychological and Cognitive Sciences, 120 , 1–6.
Google Scholar
Atkinson, J. W. (1957). Motivational determinants of risk-taking behavior. Psychological Review, 64 , 359–372. https://doi.org/10.1037/h0043445
Bandura, A. (1997). Self-efficacy: The exercise of control . W. H. Freeman.
Bandura, S. (1977). Self-efficacy: Toward a unifying theory of behavioral change. Psychological Review, 84 (2), 191–215.
Barron, K. E., & Hulleman, C. S. (2015). Expectancy-value-cost model of motivation. In J. S. Eccles & K. Salmelo-Aro (Eds.), International encyclopedia of social and behavioral sciences: Motivational psychology (2nd ed.). Elsevier.
Battle, A., & Wigfield, A. (2003). College women’s value orientations toward family, career, and graduate school. Journal of Vocational Behavior, 62 , 56–75.
Battle, E. (1965). Motivational determinants of academic task persistence. Journal of Personality and Social Psychology, 2 , 209–218.
Battle, E. (1966). Motivational determinants of academic competence. Journal of Personality and Social Psychology, 4 , 534–642.
Beymer, P. N., Flake, J. K., & Schmidt, J. A. (2023). Disentangling students’ anticipated and experiences cost: The case for understanding both. Journal of Educational Psychology, 115 (4), 624–641.
Bronfenbrenner, U. (1986). Ecology of the family as a context for human development: Research perspectives. Developmental Psychology, 22 , 723–742.
Carnegie Council on Adolescent Development. (1989). Turning points: Preparing American youth for the 21 st century. Carnegie Foundation.
Crandall, V. C. (1969). Sex differences in expectancy of intellectual and academic reinforcement. In C. P. Smith (Ed.), Achievement -related motives in children (pp. 11–45). New York: Russell Sage Foundation.
Crandall, V. C., Katkovsky, W., & Crandall, V. J. (1965). Children’s beliefs in their own control of reinforcements in intellectual-academic achievement situations. Child Development, 36 , 91–109.
Diemer, M.A, Marchand, A. & McKellar, S. & Malanchuk, O. (2016). Promotive and corrosive factors in African American students’ math beliefs and achievement. Journal of Youth & Adolescence, 45 (6), 1208–1225.
Eccles, J. S. (1987). Gender roles and women’s achievement-related decisions. Psychology of Women Quarterly, 11 , 135–172.
Eccles, J. S. (1993). School and family effects on the ontogeny of children’s interests, self-perceptions, and activity choice. In J. Jacobs (Ed.), Nebraska symposium on motivation, 1992: Developmental perspectives on motivation (pp. 145–208). University of Nebraska Press.
Eccles, J. S. (2005). Subjective task values and the Eccles et al. model of achievement-related choices. In A. J. Elliot & C. S. Dweck (Eds.), Handbook of competence and motivation (pp. 105–121). Guilford.
Eccles, J. S. (2009). Who am I and what am I going to do with my life? Personal and collective identities as motivators of action. Educational Psychologist, 44 (2), 78–89.
Eccles, J. S., Adler, T. F., & Meece, J. L. (1984). Sex differences in achievement: A test of alternate theories. Journal of Personality and Social Psychology, 46 (1), 26–43.
Eccles, J. S., & Jacobs, J. E. (1986). Social forces shape math attitudes and performance. Signs: Journal of Women in Culture and Society, 11 (2), 367–380.
Eccles, J. S., Jacobs, J. E., & Harold, R. D. (1990). Gender-role stereotypes, expectancy effects, and parents’ socialization of gender differences. Journal of Social Issues, 46 , 183–201.
Eccles, J. S., & Midgley, C. (1989). Stage/environment fit: Developmentally appropriate classrooms for early adolescents. In R. Ames & C. Ames (Eds.), Research on motivation in education (Vol. 3, pp. 139–181). Academic Press.
Eccles, J., & Wigfield, A. (1985). Teacher expectations and student motivation. In J. B. Dusek (Ed.), T eacher expectancies (pp. 185–226). Erlbaum.
Eccles, J. S., & Wigfield, A. (2020). From expectancy-value theory to situated expectancy value theory: A developmental, social cognitive, and sociocultural perspective on motivation. Contemporary Educational Psychology, 61, 1018959
Eccles, J. S., Wigfield, A., & Schiefele, U. (1998). Motivation to succeed. In W. Damon (Series Ed.) & N. Eisenberg (Volume Ed.), Handbook of child psychology (5th ed., Vol. III, pp. 1017–1095). Wiley.
Eccles, J. S., & Wigfield, A. (1995). In the mind of the achiever: The structure of adolescents’ academic achievement related-beliefs and self-perceptions. Personality and Social Psychology Bulletin, 21 , 215–225.
Eccles, J. S., & Wigfield, A. (2023). Expectancy-value theory to situated expectancy-value theory: Reflections on 40+ years of working together. Motivation Science, 9 , 1–12.
Eccles, J. S., Wigfield, A., Harold, R., & Blumenfeld, P. B. (1993). Age and gender differences in children’s self- and task perceptions during elementary school. Child Development, 64 , 830–847. https://doi.org/10.1111/j.1467-8624.1993.tb02946.x
Eccles-Parsons, J., Adler, T. F., Futterman, R., Goff, S. B., Kaczala, C. M., Meece, J. L., & Midgley, C. (1983). Expectancies, values, and academic behaviors. In J. T. Spence (Ed.), Achievement and achievement motivation (pp. 75–146). W. H. Freeman.
Eccles-Parsons, J., Adler, T. F., & Kaczala, C. M. (1982a). Socialization of achievement attitudes and beliefs: Parental influences. Child Development, 53 , 310–321.
Eccles-Parsons, J., Kaczala, C. M., & Meece, J. L. (1982b). Socialization of achievement attitudes and beliefs: Classroom influences. Child Development, 53 , 322–339.
Eccles-Parsons, J., Meece, J. L., Adler, T. F., & Kaczala, C. M. (1982c). Sex differences in attributions and learned helplessness. Sex Roles, 8 (4), 421–432.
Flake, J. K., Barron, K. E., Hulleman, C., McCoach, D. B., & Welsh, M. E. (2015). Measuring cost: The forgotten component of expectancy-value theory. Contemporary Educational Psychology, 41 , 232–244.
Gaspard, H., Dicke, A., Flunger, B., Brisson, B., Hafner, I., Nagengast, B., & Trautwein, U. (2015). Fostering adolescents’ value beliefs for mathematics with a relevance intervention in the classroom. Developmental Psychology, 51 (9), 1226–1240.
Gaspard, H., Parrisius,C., Piesch, H., Wille, E., Nagengast, B., Trautwein,U., & Hulleman, C. S. (2019). The effectiveness of a utility-value intervention in math classrooms: A cluster-randomized trial. In M. Bong (Chair) Motivation interventions proven to work in K–12 classrooms: Improving students' value, confidence, self-regulation, and satisfaction (Symposium) . America Educatonal Research Association 103rd Annual Meeting, Toronto, CA.
Gaspard, H., Lauermann, F., Rose, N., Wigfield, A., & Eccles, J. S. (2020). Profiling the cross-domain development of students’ motivations: Joint trajectories of students’ ability self-concepts and values in math and language arts. Child Development, 91 , 1800–1818.
Greene, J. A. (2022). What can educational psychology learn from, and contribute to, theory development scholarship? Educational Psychology Review, 34 , 3011–3035.
Harackiewicz, J. M., Canning, E. A., Tibbetts, Y., Priniski, S. J., & Hyde, J. S. (2015). Closing achievement gaps with a utility-value intervention: Disentangling race and social class. Journal of Personality and Social Psychology, 111 (5), 745–765. https://doi.org/10.1037/pspp0000075
Harackiewicz, J. M., & Priniski, S. (2018). Improving student outcomes in higher education: The science of targeted interventions. Annual Review of Psychology, 69 , 409–435. https://doi.org/10.1146/annurev-psych-122216-011725
Harackiewicz, J. M., Rozek, C. S., Hulleman, C. S., & Hyde, J. S. (2012). Helping parents motivate adolescents in mathematics and science: An experimental test of a utility-value intervention. Psychological Science, 23 , 899–906.
Harackiewicz, J. M., Tibbits, Y., Canning, E., & Hyde, J. S. (2014). Harnessing values to promote motivation in education. In S. Karabenick & T. Urdan (Eds.), Advances in motivation and achievement: Motivational interventions (vol. 18, pp. 71–106). Emerald.
Hecht, C. A., Priniski, S. J., & Harackiewicz, J. M. (2019a). Understanding long-term effects of motivation interventions in a changing world. In E. N. Gonida & M. S. Lemos (Eds.), Advances in motivation and achievement (Vol. 20, pp. 81–98). Emerald.
Hecht, C. A., Harackiewicz, J. M., Priniski, S. J., Canning, E. A., Tibbetts, Y., & Hyde, J. S. (2019b). Promoting persistence in the biological and medical sciences: An expectancy-value approach to intervention. Journal of Educational Psychology, 111 , 1462–1477.
Heller, K. A., & Parsons, J. E. (1981). Sex differences in teachers’ evaluative feedback and students’ expectancies for success in mathematics. Child Development, 52 , 1015–1019.
Higgins, E., & Eccles-Parsons, J. (1983). Social cognition and the social life of the child: Stages as subcultures. In E. Higgins, D. Ruble, & W. Hartup (Eds.), Social cognition and social development (pp. 15–62). Cambridge University Press.
Hilpert, J. C., & Marchand, G. (2018). Complex systems research in educational psychology: Aligning theory and method. Educational Psychologist, 53.
Hulleman, C. S., & Harackiewicz, J. M. (2009). Promoting interest and performance in high school science classes. Science, 326 , 1410–1412.
Jacobs, J., & Eccles, J. (1986). Gender differences in math ability: The impact of media reports on parents. Educational Researcher, 14 , 20–25.
Jacobs, J., Lanza, S., Osgood, D. W., Eccles, J. S., & Wigfield, A. (2002). Ontogeny of children’s self-beliefs: Gender and domain differences across grades one through 12. Child Development, 73 , 509–527.
Kaplan, A., & Garner, J. K. (2017). A complex dynamic systems perspective on Iientity and its development: The dynamic systems model of role identity. Developmental Psychology, 53 (11), 2036–2051.
Kaplan, A., & Garner, J. K. (2020). Steps for applying the complex dynamical systems approach in educational research: A guide for the perplexed scholar. The Journal of Experimental Education, 88 (3), 486–502.
Kumar, R., Zusho, A., & Bondie, R. (2018). Weaving cultural relevance and achievement motivation into inclusive classrooms. Educational Psychologist, 53 , 73–96.
Ladson-Billings, G., & Tate, W. (1995). Toward a critical race theory of education. Teachers College Record, 97 (1), 47–68.
Liem, G. A. D., & McInerney, D. (Eds.). (2018). Recent advances in sociocultural influences on motivation and learning: Big theories revisited (2nd Ed., pp. 91–116). Information Age Press.
Matthews, & Wigfield. (in press). Past due! Racializing aspects of situated expectancy-value theory. Motivation Science.
McInerney, D., & Van Etten. S. (Eds.). (2004). Research on sociocultural influences on motivation and learning volume 4: Big theories revisited . Information Age Press.
Meece, J., Wigfield, A., & Eccles, J. (1990). Predictors of math anxiety and its influence on young adolescents’ course enrollment intentions and performances in mathematics. Journal of Educational Psychology, 82 , 60–70.
Midgley, C., Feldlaufer, H., & Eccles, J. S. (1988). The transition to junior high school: Beliefs of pre- and posttransition teachers. Journal of Youth and Adolescence, 17 (6), 543–562.
Midgley, C. M., Feldlaufer, H., & Eccles, J. S. (1989a). Changes in teacher efficacy and student self- and task-related beliefs during the transition to junior high school. Journal of Educational Psychology, 81 , 247–258.
Midgley, C., Feldlaufer, H., & Eccles, J. S. (1989b). Student/teacher relations and attitudes toward mathematics before and after the transition to junior high school. Child Development, 60 , 981–992.
Muenks, K., Miller, J. E., Scheutze, B. A., & Whitaker, T. A. (2023). Is cost separate from or part of subjective task value? An empirical examination of expectancy-value versus expectancy-value-cost perspectives. Contemporary Educational Psychology, 72 , 102149.
Musu-Gillette, L. E., Wigfield, A., Harring, J., & Eccles, J. S. (2014). Trajectories of change in student’s self-concepts of ability and values in math and college major choice. Educational Research and Evaluation, 21 (4), 343.
Nagengast, B., & Trautwein, U. (2023). Theoretical and methodological disintegration is the most fundamental limitation in contemporary motivation research. In M. Bong, JM Reeve, & S-I. Kim (Eds.), Motivation science: Controversies and insights (pp. 419–424). Oxford University Press.
Neblett, E. W., Rivas-Drake, D., & Umana-Taylor, A. J. (2021). The promise of racial and ethnic protective factors in promoting ethnic minority youth development. Child Development Perspectives, 6 (3), 295–303.
Nolen, S. B. (2020). A situative turn in the conversation on motivation theories. Contemporary Educational Psychology, 61 , 101866.
Pajares, F. (1996). Self-efficacy in academic settings. Review of Educational Research, 66 (4), 543–578.
Parsons, J. E., Frieze, I. H., & Ruble, D. N. (1976). Introduction. Special issue. Journal of Social Issues, 32 (3), 1–3.
Parsons, J. E., & Goff, S. B. (1980). Achievement motivation and values: An alternative perspective. In L. J. Fyans (Ed.), Achievement motivation. Springer.
Parsons, J. E., & Ruble, D. N. (1977). The development of achievement-related expectancies. Child Development, 48 , 1075–1079.
Part, R. M., Perea, H. N., Marchand, G. C., & Bernacki, M. L. (2020). Revisiting the dimensionality of subjective task value: Towards clarification of competing perspectives. Contemporary Educational Psychology, 62 , 101875.
Peck, S., Brodish, A., Malanchuk, O., Banerjee, M., & Eccles, J. S. (2014). Racial/ethnic socialization and identity development in Black families: The role of parent and youth reports. Developmental Psychology, 50 (7), 1897–1909.
Perez, T., Cromley, J. G., & Kaplan, A. (2014). The role of identity development, values, and costs in college STEM retention. Journal of Educational Psychology, 106 , 315–329.
Rosenzweig, E. Q., & Wigfield, A. (2016). STEM motivation interventions for adolescents: A promising start, but farther to go. Educational Psychologist, 51 , 146–163.
Rosenzweig, E. Q., Wigfield, A., & Eccles, J. S. (2022). Beyond utility value interventions: Recommendations for next steps in expectancy-value intervention research. Educational Psychologist, 57 (1), 11–30.
Rosenzweig, E. Q., Wigfield, A., & Hulleman, C. S. (2020). More useful, or not so bad? Examining the effects of utility value and cost reduction interventions in college physics. Journal of Educational Psychology, 112 , 166–182. https://doi.org/10.1037/edu0000370
Ruble, D. N., Frieze, I. H., & Parsons, J. E. (Eds.). (1976). Special Issue: Sex roles: Persistence and change. Journal of Social Issues, 32 (3).
Ryan, R. M., & Deci, E. L. (2017). Self-determination theory: Basic psychological needs in motivation, wellness, and development . Guilford.
Book Google Scholar
Ryan, R. M., & Deci, E. L. (2020). Intrinsic and extrinsic motivation for a self-determination theory perspective: Definitions, theory, practices, and future directions. Contemporary Educational Psychology, 61 , 101860.
Simmons, R. G., & Blyth, D. A. (1987). Moving into adolescence: The impact of pubertal change and school context . Aldine de Gruyter.
Simpkins, S. D., Fredricks, J., & Eccles, J. S. (2015). The role of parents in the ontogeny of achievement-related motivation and behavioral choices. Monographs of the Society for the Study of Child Development, 80 (2), 1–22. https://doi.org/10.1111/mono.12157
Song, U., Rosenzweig, E. Q., & Barger, M. M. (2023). Disentangling the relation among emotional cost, psychological cost, and anxiety with college students. Motivation and Emotion, 47 (6), 1046–1061.
Tabron, L. A., & Venzant Chambers, T. T. (2019). What is being Black and high achieving going to cost me in your school? Students speak out about their educational experiences through a racial opportunities lens. The High School Journal, 102 , 118–138.
Tonks, S. M., Wigfield, A., & Eccles, J. S. (2018). Expectancy value theory in cross-cultural perspective: What have we learned in the last 15 years? In G. A. D. Liem, & D. McInerney (Eds.), Recent advances in sociocultural influences on motivation and learning: Big theories revisited (2 nd Ed). Information Age Publishers.
Venzant Chambers, T. T. (2022). Racial opportunity cost: The toll of academic success on Black and Latinx students . Harvard Education Press.
Vygotsky, L. (1962). Thought and language . MIT Press.
Wigfield, A. (1994). Expectancy - value theory of achievement motivation: A developmental perspective. Educational Psychology Review, 6 , 49–78.
Wigfield, A., & Eccles, J. (1992). The development of achievement task values: A theoretical analysis. Developmental Review, 12 , 265–310.
Wigfield, A., & Eccles, J. S. (2000). Expectancy-value theory of motivation. Contemporary Educational Psychology, 25 , 68–81.
Wigfield, A., & Eccles, J. S. (2020). 35 years of research on students’ subjective task values and motivation: A look back and a look forward. In A. Elliot (Ed.), Advances in motivation science (Vol. 7, pp. 161–198). Elsevier.
Wigfield, A., Eccles, J., Mac Iver, D., Reuman, D., & Midgley, C. (1991). Transitions during early adolescence: Changes in children’s domain-specific self-perceptions and general self-esteem across the transition to junior high school. Developmental Psychology, 27 , 552–565.
Wigfield, A., Eccles, J. S., & Möller, J. (2020). How dimensional comparisons help to understand linkages between expectancies, values, performance, and choice. Educational Psychology Review, 32 , 657–680.
Wigfield, A., Eccles, J. S., Schiefele, U., Roeser, R., & Davis-Kean, P. (2006). Development of achievement motivation. In W. Damon & N. Eisenberg (Eds.), Handbook of child psychology (6th ed., Vol. 3, pp. 933–1002). Wiley.
Wigfield, A., Eccles, J. S., Yoon, K. S., Harold, R. D., Arbreton, A., Freedman-Doan, C., & Blumenfeld, P. C. (1997). Changes in children’s competence beliefs and subjective task values across the elementary school years: A three-year study. Journal of Educational Psychology, 89 , 451–469. https://doi.org/10.1037/0022-0663.89.3.451
Wigfield, A., & Gladstone, J. (2019). How students’ expectancies and values relate to their achievement in times of global change and uncertainty. In E. N. Gonida & M. Lemos (Eds.), Motivation in education at a time of global change: Theory, research, and implications for practice (Advances in motivation and achievement, Vol. 20, pp. 15-32). Emerald.
Wigfield, A., Rosenzweig, E. Q., & Eccles, J. S. (2017). Achievement values. In A. Elliot & C. S. Dweck (Eds.), Handbook of competence and motivation (2nd ed., pp. 116–134). Guilford.
Wigfield, A., Tonks, S., & Klauda, S. L. (2016). Expectancy-value theory. In K. R. Wentzel & D. Miele (Eds.), Handbook of motivation in school (2nd ed., pp. 55–74). Routledge.
Download references
Acknowledgements
We would like to thank the many wonderful colleagues who took part in these endeavors with us.
We received grant support for the research described in this article from NICHD, NIE, NIMH, NSF, and the Spencer Foundation.
Author information
Authors and affiliations.
School of Education, University of California, Irvine, Irvine, CA, USA
Jacquelynne S. Eccles
Department of Human Development and Quantitative Methodology, University of Maryland, College Park, MD, USA
Allan Wigfield
Department of Education and the Brain and Motivation Research Institute (bMRI), Korea University, Seoul, Korea
University of California Irvine, 3200 Education Bldg, Irvine, CA, 92697, USA
You can also search for this author in PubMed Google Scholar
Corresponding authors
Correspondence to Jacquelynne S. Eccles or Allan Wigfield .
Ethics declarations
Conflict of interest.
The authors declare no competing interests.
Additional information
Publisher's note.
Springer Nature remains neutral with regard to jurisdictional claims in published maps and institutional affiliations.
This article is part of the Topical Collection on Theory Development in Educational Psychology
Rights and permissions
Open Access This article is licensed under a Creative Commons Attribution 4.0 International License, which permits use, sharing, adaptation, distribution and reproduction in any medium or format, as long as you give appropriate credit to the original author(s) and the source, provide a link to the Creative Commons licence, and indicate if changes were made. The images or other third party material in this article are included in the article's Creative Commons licence, unless indicated otherwise in a credit line to the material. If material is not included in the article's Creative Commons licence and your intended use is not permitted by statutory regulation or exceeds the permitted use, you will need to obtain permission directly from the copyright holder. To view a copy of this licence, visit http://creativecommons.org/licenses/by/4.0/ .
Reprints and permissions
About this article
Eccles, J.S., Wigfield, A. The Development, Testing, and Refinement of Eccles, Wigfield, and Colleagues’ Situated Expectancy-Value Model of Achievement Performance and Choice. Educ Psychol Rev 36 , 51 (2024). https://doi.org/10.1007/s10648-024-09888-9
Download citation
Accepted : 23 April 2024
Published : 17 May 2024
DOI : https://doi.org/10.1007/s10648-024-09888-9
Share this article
Anyone you share the following link with will be able to read this content:
Sorry, a shareable link is not currently available for this article.
Provided by the Springer Nature SharedIt content-sharing initiative
- Situated expectancy-value theory
- Decision-making
- Gender differences
Advertisement
- Find a journal
- Publish with us
- Track your research
Academia.edu no longer supports Internet Explorer.
To browse Academia.edu and the wider internet faster and more securely, please take a few seconds to upgrade your browser .
Enter the email address you signed up with and we'll email you a reset link.
- We're Hiring!
- Help Center
Moderating Effect of Homeschooling on Retention and Academic Achievement of STEM Majors at a Private, Faith-Based, Liberal Arts College

Related Papers
Journal of College Student Development
Thalia Mulvihill
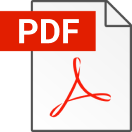
Jeanine SanClemente
College Transition Experiences of Women Who Were Homeschooled: A Phenomenological Study by Jeanine SanClemente MA, Seattle University, 1991 BA, University of Washington, 1990 Dissertation Submitted in Partial Fulfillment of the Requirements for the Degree of Doctor of Philosophy Social and Behavioral Sciences
Journal of College Orientation, Transition, and Retention
Jeannine Kranzow
Jeremey Wolfe
Kayla Gilmore
With the rise of families choosing to homeschool their children, myths abound on the effectiveness of homeschooling in regard to socialization and academic achievement. For this reason, this study identifies how homeschool students stack up against public school students academically. To determine this, multiple factors have been explored: the curriculum that is being used in both educational environments, self-motivation of students, standardized test scores, which are a set of questions and scores given to all the students in a standard way in a state to compare the relative performance of students, and college success from both educational environments. In this study, primary students, students between the ages of 5 and 11, have been the main focus in identifying academic achievement.
Journal of School Choice
Philip Sadler
Kyle Gracey
This mixed-method study identifies cyber high school graduates' perceptions of the effect of a cyber high school education on successful transition to traditional university. The study examined students’ perceptions of the advantages and disadvantages their cyber education experience contributed to their academic and social transition to college. In addition, the level of involvement of cyber high school graduates in university-based social activities was compared to the similar involvement of their university peers. The study was conducted at four universities in the Pennsylvania State System of Higher Education. The analyses of quantitative data from a survey of 32 cyber high school graduates’ academic self-efficacy showed that cyber high school graduates believed they had the academic abilities to succeed in college. Responses from participants for select questions from the 2008 National Survey of Student Engagement were compared to responses by a representative sample of the general student population at study sites to the same questions. Results indicated that involvement of cyber high school graduates in university-based social activities was not significantly different than the involvement of their university peers. Qualitative analysis was applied to data from focus group interviews involving 14 cyber high school graduates. Findings revealed that unique features of cyber education, particularly related to pace and learner independence, had an effect on cyber high school graduates’ early college experiences. Negative academic transition experiences resulted from adjustments to a loss of control over the flexibility and pace of their learning. Negative social transition experiences resulted from the increased daily interactions with peers that cyber graduates did not have during their cyber high school experiences. However, students reported more positive than negative effects. The majority of students believed their cyber education adequately prepared them for transition to college. Participants perceived they gained academic skills in cyber high school which enabled successful transition. They also believed themselves to be socially well-adjusted.
Rebecca Lambert
Acta Educationis Generalis
Kinga M A G D O L N A Mandel
Introduction: In the presented article, we are looking for the solutions and challenges of homeschooling in terms of further education and labour market inclusion. Purpose: The purpose of the paper is to discuss the conceptual framework of a research-initiative on homeschooling. What are the consequences? Because of a lack of adequate state language usage, is there a forced migration in the pupils’ career paths? To what extent are homeschoolers included into traditional compulsory education, lifelong learning, the labor market, and the society? Methods: This is a paper with the conceptual framework of a research, where snowball sampling based qualitative interviews are planned. Results: From the research to be conducted, the authors expect a reliable picture of the causes, challenges and consequences of homeschooling on lifelong learning, the labour market and social inclusion. Discussion: It seems that homeschooling in the Seclerland is a de-schooling solution, because it is usuall...
Canadian Journal of Behavioural Science/Revue canadienne des sciences du comportement
Sandra Martin-chang
RELATED PAPERS
Terry Harding
Joseph (Joey) M . Sabol
Opus et Educatio
Denise Carr
billy achia
The Journal of Higher Education
Donald L Rubin
Journal of Pedagogy
Marta McCabe
Timothy Hagen
james walke
Journal of advances in education and philosophy
lydia wamocha
Problems of Education in the 21st Century
Pamela Buhere
Literacy in Composition Studies
Alicia McCartney
Boulder and Tempe: Education and the Public …
Brandi Roberts
PhD, Indiana University of Pennsylvania
Elaine Huber
Journal of Adolescence
Sharon Greenhennessy
Leo Pedraza
Amy Rathbun
Billy Gage Raley
American Speech
Lauren Hall-Lew
Dawn Rockholt
Abby Ampuja
MICHAEL ARYEE
Online Submission
Katherine Green , Dr. Carolyn McKeon
Ashley Sweat
Roydon Pellew
Andrew Mandley
Research in Higher Education
Community College Journal of Research and Practice
Daniel Solorzano
Debbie Barry
University of St. Thomas Journal of Law and Public Policy
Mary Hasson
Jillian Kinzie
Andrea Clements
Dr. Consuela Cooper
Kesha James
Cynthia Avery
Steven Wilkerson
RELATED TOPICS
- We're Hiring!
- Help Center
- Find new research papers in:
- Health Sciences
- Earth Sciences
- Cognitive Science
- Mathematics
- Computer Science
- Academia ©2024

IMAGES
VIDEO
COMMENTS
Success Starts With Critical Thinking, Problem-Solving Skills. The robot lab at the University of Illinois at Urbana-Champaign's Department of Computer Science. Image: joefutrelle/Flickr. Our ...
Employment in STEM occupations has grown 79 percent in the past three decades, according to U.S. Bureau of Labor Statistics. ... teaches critical-thinking skills, and instills a mindset that will help students find success across numerous areas and disciplines. However, Long said, "too often the opportunity to learn and to be inspired by STEM ...
In the diverse landscape of today's industries, professionals in STEM careers are often the catalysts for innovation and progress. As you navigate complex challenges, critical thinking becomes ...
The design of a STEM lesson also leads to foster student's critical thinking skills. "Experiences that are interdisciplinary and supported by collaborative problem-based, design-based, and/or inquiry learning strategies can have a significant impact on students' critical thinking skills" (Rehman, 2015, p. 63).
This article emphasizes the pivotal role of professionals in STEM careers as drivers of innovation and highlights critical thinking as an indispensable skill in navigating complex challenges. It offers ten actionable tips for refining critical thinking capabilities in STEM.
thinking skills and approach to learning, both critical roots of STEM success. After all, the STEM disciplines require not only content knowledge but also robust thinking dispositions—such as curiosity and inquiry, questioning and skepticism, assessment and analysis—as well as a strong learning mindset and confidence when
KEY ISSUES AND PROMISING PROGRAMS. This brief, the ninth in a series, explores issues and promising practices in paving CTE pathways to high-STEM occupations—a key piece of the STEM college and career readiness puzzle. Other briefs focus on related parts of the puzzle, such as the role that math and science standards play in students ...
skills—generally thought to be core skills for STEM occupations—were extremely important in their job. Notably, the importance of noncognitive or implicit skills on the job increases as workers age, while the importance of technical skills remains relatively constant. Findings in the survey will provide workers,
Critical thinking in science and many other disciplines should encompass creative, analytical, practical, and wise thinking. Underlying it are both cognitive processes and dispositions-that is, what a person can do and what a person chooses to do. Critical thinking is both domain-general and domain-specific. The domain-specific elements of it ...
The framework comprises critical thinking (including critical mathematical modelling and philosophical inquiry), systems thinking, and design-based thinking. Collectively, these thinking skills contribute to adaptive and innovative thinking (McKenna, 2014) and ultimately lead to the development of learning innovation (Sect. 3.4; English, 2018).
These thinking skills have been chosen because of their potential to enhance STEM-based problem solving and interdisciplinary concept development (English et al., 2020; Park et al., 2018; Slavit et al., 2021).Highlighting these ways of thinking, however, is not denying the importance of other thinking skills such as creativity, which is incorporated within the adaptive and innovative thinking ...
The Effects of STEM Education on the Students' Critical Thinking Skills and STEM Perceptions. Yasemin Hacioglu, Filiz Gulhan. Article Info Abstract Article History Received: 18 July 2020 Critical thinking and STEM career perception are important in 21th century and STEM education is necessary to promote middle school students' critical
The top five skills were active learning (on the job), critical thinking, complex problem-solving, creative problem-solving, and interpersonal skills. Occupation-specific STEM skills came down the list at number 8. All but the last of these five was held to be more characteristic of STEM, compared to non-STEM employees.
Nearly half of STEM workers say good written and communication skills are extremely important in their job, and nearly 70 percent say the same of critical thinking skills. By comparison, only 36 percent of STEM workers said high-level math, analytical, or computer skills—generally thought to be core skills for STEM occupations—were ...
STEM career aspirations. STEM career aspirations can be considered as a subset of career aspirations, defined as 'an individual's expressed career-related goals or choices' (Rojewski, 2005, p. 132), which contribute to future STEM-related career paths (Mau & Li, 2018).Hou and Leung explained that STEM career aspirations refer to the evolving goals, ideals, and intentions of individuals ...
One of the main benefits of STEM education is helping students develop critical thinking and problem-solving skills, which are essential for success in any career field. It encourages students to think creatively and independently and helps them understand and apply complex concepts and ideas. A former National Math and Science Initiative ...
Successful job performance in STEM extends beyond having technical knowledge and skills, requiring additional competencies and the ability to perform unfamiliar tasks. For example, employees are expected to contribute to the development of less experienced employees, provide extra effort when needed, and be a supporter of organizational ...
Increase teamwork and collaboration. Develop critical thinking skills. Explore careers and occupations. Boost students social-emotional learning. Boost curiosity. Outcomes of STEM Education: Learning and Achievement. STEM education helps students engage in the creation, planning, improving, and testing of inventions across various disciplines.
Soft Skills for STEM Success. Author: Katie McPhee. While there are currently thousands of STEM positions open, there is a lack of work-ready STEM professionals graduating from higher education institutions. They may have the required technical skills, but many candidates lack the soft skills necessary for workplace success.
seen as a set of skills that are needed to contribute to student success during the study and in the workplace (Partnership for Twenty-first Century Skills: 2003). at is why Universities strive to develop students' critical thinking skills. In modern discussions about the nature of higher education, the concept that emerges most widely is
STEM Education is important for kids because it teaches thinking skills that will help them to be successful in any career that they choose. Kids who are studying STEM often have the opportunity to work on projects and figure out how to solve real-life problems. Kids learn to work in groups with other students.
A STEM major is any student who pursues the studies of science, technology, engineering or mathematics or the subfields of these majors. STEM jobs are expected to grow faster than all other occupations in the next five years. STEM programs open a window of opportunity, as there are many different potential career paths to choose from, including ...
Check out the top ways STEM education gives students the core skills they need to be successful in the modern workplace. 1. STEM education develops problem-solving skills. Problem-solving is a must for a variety of different career paths but is even more important for STEM careers where solving problems is the entire purpose of the work.
Embrace the Benefits of STEM and Design Thinking. Here are 5 ways to set yourself up for success when teaching t STEM education: Provide a dedicated space for STEM learning - At WhyMaker, we have lots of resources and we are here to support you in creating an exciting Makerspace for teaching STEM. Here's a guide for creating your own ...
The critical thinking and problem-solving skills developed in STEM subjects apply to virtually all other fields and pursuits. And you can never really know when or how a student might find their ...
4 Engage Actively. To truly thrive in your teaching career, engage with your students actively and encourage them to think critically. Pose open-ended questions that stimulate discussion and ...
To address the seven guiding questions posed for authors of articles in this special issue, we begin by discussing the development (in the late 1970s-early 1980s) of Eccles' expectancy-value theory of achievement choice (EEVT), a theory developed to explain the cultural phenomenon of why girls were less likely to participate in STEM courses and careers. We then discuss how we tested key ...
This mixed-method study identifies cyber high school graduates' perceptions of the effect of a cyber high school education on successful transition to traditional university. The study examined students' perceptions of the advantages and disadvantages their cyber education experience contributed to their academic and social transition to college.
Science is a rigorous, systematic endeavor that builds and organizes knowledge in the form of testable explanations and predictions about the world. Modern science is typically divided into three major branches: the natural sciences (e.g., physics, chemistry, and biology), which study the physical world; the social sciences (e.g., economics, psychology, and sociology), which study individuals ...
Committee: House Education and the Workforce: Related Items: Data will display when it becomes available. Date: