- Search Menu
- Sign in through your institution
- Author Guidelines
- Submission Site
- Open Access Options
- Self-Archiving Policy
- Reasons to Submit
- About Journal of Surgical Protocols and Research Methodologies
- Editorial Board
- Advertising & Corporate Services
- Journals on Oxford Academic
- Books on Oxford Academic


Article Contents
Cardiothoracic surgery, general surgery, head and neck surgery, orthopaedic surgery, urology and gynaecology, conflict of interest statement.
- < Previous
Robotic surgery: an evolution in practice

- Article contents
- Figures & tables
- Supplementary Data
Elizabeth Z Goh, Tariq Ali, Robotic surgery: an evolution in practice, Journal of Surgical Protocols and Research Methodologies , Volume 2022, Issue 1, January 2022, snac003, https://doi.org/10.1093/jsprm/snac003
- Permissions Icon Permissions
Robotic surgery is a progression on the minimally invasive spectrum and represents an evolution in practice across numerous disciplines.
From its origins in the late 1980s, pioneering technologies like the ROBODOC for hip replacements and the PROBOT for urological procedures were early iterations of the idea that mechanical augmentations could at the very least be useful adjuncts in the complex task that is surgery [ 1 ]. In the 1990s, researchers from the United States (US) National Aeronautics and Space Administration and Stanford Research Institute investigated the potential of robotics for telepresence surgery [ 1 ]. Subsequent US Army funding attempted to devise a system to remotely operate on wounded soldiers via robotic equipment, in hopes of decreasing battlefield mortality [ 1 ]. Commercial development introduced Automated Endoscopic System for Optimal Positioning (AESOP) (Computer Motion, CA), a voice-controlled robotic arm with an endoscopic camera, to the civilian surgical community [ 1 ]. This was superseded in the 2000s by two comprehensive master–slave platforms: the da Vinci system (Intuitive Surgical, CA), an eponymous nod to Leonardo da Vinci’s fifteenth-century ‘mechanical knight’ automaton [ 2 ], and the Zeus system (Computer Motion, CA), which was designed for cardiac surgery [ 1 ]. A company merger established the former as today’s main platform [ 1 ].
The da Vinci system consists of a console from which the surgeon remotely controls arms connected to a robotic cart beside the patient [ 3 ]. A dual-camera endoscope mounted on one arm transmits images of the surgical field to the console, providing the surgeon with a magnified three-dimensional (3D) view [ 3 ]. In response, the surgeon manipulates instruments attached to the other arms via the console [ 3 ]. The assistant is positioned beside the patient to suction and retract at the surgical field [ 3 ].
Robotic surgery offers advantages over conventional endoscopic surgery in visualization, dexterity and ergonomics, while maintaining the peri-operative benefits of minimally invasive surgery [ 1 ]. The dual-camera system offers 3D views with depth perception, unlike conventional endoscopic views [ 1 ]. Precision features include articulated ‘EndoWrist’ instruments with increased degrees of freedom, removal of the fulcrum effect and motion scaling with tremor filtration [ 1 , 3 ]. Accordingly, objective advantages over laparoscopic techniques in terms of dexterity and muscle fatigue have been demonstrated [ 4 ]. The remote console also allows an ergonomic operating position while optimizing visualization and manoeuvrability [ 1 ]. Recent da Vinci iterations have included a reconfigured robotic arm design to improve access; faster docking to reduce operative time; fluorescence-detection to identify structures and lesions of interest; robotic staplers to overcome difficulties in endoscopic stapler positioning by the assistant and a dual console for training [ 5 , 6 ].
Feasibility, efficacy and cost considerations exist. Access concerns may be ameliorated with a pre-operative screening endoscopy, whereas operative time reduces with experience [ 3 ]. Ongoing technological advances and global uptake of robotic surgery are expected to improve efficacy through optimization of case selection and equipment guided by growing longitudinal data [ 3 ]. Purchase and maintenance costs are significant, but will be offset by high volume use as well as savings from reduced length of stay and improved clinical outcomes [ 3 ].
The benefits of 3D vision and enhanced manoeuvrability provided by robotic surgery are crucial in the mediastinum, which contains many vital structures. Myriad applications exist for cardiac surgery, including cardiac revascularization and mitral valve repair, which were some of the earliest robotic surgeries performed [ 7 ]. Robotic thymectomy for thymomas is aided by fluorescence-guided detection of the tumour and adjacent structures [ 5 ]. Robotic lobectomy for lung cancer is also gaining traction, with Yang et al. ’s 10-year cohort study reporting comparable oncologic and peri-operative outcomes to video-assisted and open approaches [ 8 ].
Robotic surgery is feasible for numerous general surgical procedures, pending cost and operative time considerations, which will improve with technological advances. It has been used for rectal cancer resection, with the 2017 ROLARR trial finding comparable open conversion rates with laparoscopic techniques [ 9 ], and Lee et al. ’s large cohort study finding comparable resection quality with transanal techniques [ 10 ]. Robotic surgery is also a safe and effective clinical alternative for common operations such as gastrectomy [ 11 ], Roux-en-Y gastric bypass [ 12 ] and thyroidectomy [ 13 ]; as well as rare procedures such as median arcuate ligament (MAL) release in MAL syndrome [ 14 ]. Recent da Vinci iterations incorporate a more flexible robotic arm configuration to simplify set-up and facilitate four-quadrant access for complex procedures, and specific single-site surgery instruments with similar peri-operative benefits to single-port laparoscopic surgery [ 6 ].
The head and neck area is difficult to access due to its complex anatomy and confined space. Transoral robotic surgery (TORS) is an emerging option for oropharyngeal carcinoma, as it enables minimally invasive access to the oropharynx without large and mutilating open procedures such as a mandibulotomy and/or pharyngotomy, which cause significant functional and aesthetic deficits [ 15 ]. It also offers similar oncologic and functional outcomes to radiotherapy, pending further comparisons [ 16 , 17 ]. In addition, TORS is being increasingly used for cancers of unknown origin. Systematic reviews by Farooq et al. [ 18 ] and Fu et al. [ 19 ] found that tongue base mucosectomies and lingual tonsillectomies performed with TORS and transoral laser microsurgery (TLM) identified the primary tumour in over 70% of cases with negative conventional diagnostic findings. Other indications for TORS include laryngeal tumours [ 20 ] and parapharyngeal space tumours [ 21 ]; salvage surgery [ 22 ]; free flap reconstruction [ 23 ] and sleep apnoea surgery [ 24 ].
Various robotic systems for orthopaedic procedures exist. Haptic systems, which provide intra-operative feedback based on pre-operative data for accurate resection and reconstruction, are commonly used [ 25 ]. A common application is robotic-arm-assisted total knee arthroplasty, which has been found to result in decreased iatrogenic trauma to periarticular soft tissue and bone, increased accuracy of component positioning and improved peri-operative outcomes compared to conventional jig-based techniques [ 26 , 27 ]. Cost-effectiveness analysis of robotic arthroplasty is also in progress via the Robotic Arthroplasty: a Clinical and cost Effectiveness Randomised controlled (RACER) trial [ 28 ]. Still under investigation for clinical use are passive systems, such as the da Vinci platform for hip and shoulder arthroscopy, and active systems, which can independently perform procedures without surgeon input [ 29 ].
Robotic surgery is particularly suited for surgical access within the anatomically restrained pelvic space. Robotic-assisted radical prostatectomy is one of the most common robotic procedures. It is a widely-accepted management option for prostate cancer, with Tewari et al. ’s landmark meta-analysis reporting comparable oncologic and peri-operative outcomes to laparoscopic and open techniques [ 30 ]. Robotic partial nephrectomy is an emerging indication, with Bravi et al. ’s prospective multicentre cohort study reporting better peri-operative outcomes than laparoscopic and open approaches for anatomically low-risk renal tumours [ 31 ]. Robotic surgery provides improved outcomes for complex benign hysterectomy, where superior post-operative quality-of-life may offset the increased operating time, and endometrial cancer staging, where obesity and other comorbidities are common in the population [ 32 ]. There is emerging evidence for its use in cervical and ovarian cancer [ 33 ], myomectomy and sacrocolpopexy [ 32 ].
Robotic surgery is an emerging modality across numerous surgical specialties. It offers advantages over conventional endoscopic surgery in visualization, dexterity and ergonomics, while maintaining the benefits of minimally invasive surgery. Feasibility, efficacy and cost concerns may be ameliorated with technological advances and increased uptake. Robust longitudinal comparisons with established treatment modalities are imperative to support this evolution in practice.
None declared.
This research received no specific grant from any funding agency in the public, commercial or not-for-profit sectors.
Lanfranco AR , Castellanos AE , Desai JP , Meyers WC . Robotic surgery: a current perspective . Ann Surg 2004 ; 239 : 14 .
Google Scholar
Moran ME . The da Vinci robot . J Endourol 2006 ; 20 : 986 – 90 .
Weinstein GS , O'Malley BW Jr , Desai SC , Quon H . Transoral robotic surgery: does the ends justify the means? Curr Opin Otolaryngol Head Neck Surg 2009 ; 17 : 126 – 31 .
Kuo L-J , Ngu JC-Y , Lin Y-K , Chen C-C , Tang Y-H . A pilot study comparing ergonomics in laparoscopy and robotics: beyond anecdotes, and subjective claims . J Surg Case Rep 2020 ; 2020 :rjaa005.
Zirafa CC , Romano G , Key TH , Davini F , Melfi F . The evolution of robotic thoracic surgery . Ann Cardiothorac Surg 2019 ; 8 : 210 .
Hagen ME , Tauxe WM , Morel P . Robotic applications in advancing general surgery. In: Technological Advances in Surgery, Trauma and Critical Care . New York: Springer , 2015 , 377 – 90
Google Preview
Doulamis IP , Spartalis E , Machairas N , Schizas D , Patsouras D , Spartalis M , et al. The role of robotics in cardiac surgery: a systematic review . J Robot Surg 2019 ; 13 : 41 – 52 .
Yang H-X , Woo KM , Sima CS , Bains MS , Adusumilli PS , Huang J , et al. Long-term survival based on the surgical approach to lobectomy for clinical stage I non-small cell lung cancer: comparison of robotic, video assisted thoracic surgery, and thoracotomy lobectomy . Ann Surg 2017 ; 265 : 431 .
Jayne D , Pigazzi A , Marshall H , Croft J , Corrigan N , Copeland J , et al. Effect of robotic-assisted vs conventional laparoscopic surgery on risk of conversion to open laparotomy among patients undergoing resection for rectal cancer: The ROLARR randomized clinical trial . JAMA 2017 ; 318 : 1569 – 80 .
Lee L , de Lacy B , Ruiz MG , Liberman AS , Albert MR , Monson JR , et al. A multicenter matched comparison of transanal and robotic total mesorectal excision for mid and low-rectal adenocarcinoma . Ann Surg 2019 ; 270 : 1110 – 6 .
Ojima T , Nakamura M , Hayata K , Kitadani J , Katsuda M , Takeuchi A , et al. Short-term outcomes of robotic gastrectomy vs laparoscopic gastrectomy for patients with gastric cancer: a randomized clinical trial . JAMA Surg 2021 ; 156 : 954 – 63 .
El Chaar M , King K , Salem JF , Arishi A , Galvez A , Stoltzfus J . Robotic surgery results in better outcomes following Roux-en-Y gastric bypass: Metabolic and Bariatric Surgery Accreditation and Quality Improvement Program analysis for the years 2015–2018 . Surg Obes Relat Dis 2021 ; 17 : 694 – 700 .
Chen Y-H , Kim H-Y , Anuwong A , Huang T-S , Duh Q-Y . Transoral robotic thyroidectomy versus transoral endoscopic thyroidectomy: a propensity-score-matched analysis of surgical outcomes . Surg Endosc 2021 ; 35 : 6179 – 89 .
Bustos R , Papamichail M , Mangano A , Valle V , Giulianotti PC . Robotic approach to treat median arcuate ligament syndrome: a case report . J Surg Case Rep 2020 ; 2020 :rjaa088.
Golusiński W , Golusińska-Kardach E . Current role of surgery in the management of oropharyngeal cancer . Front Oncol 2019 ; 9 : 388 .
De Virgilio A , Costantino A , Mercante G , Pellini R , Ferreli F , Malvezzi L , et al. Transoral robotic surgery and intensity-modulated radiotherapy in the treatment of the oropharyngeal carcinoma: a systematic review and meta-analysis . Eur Arch Otorhinolaryngol 2021 ; 278 : 1321 – 35 .
Nichols AC , Theurer J , Prisman E , Read N , Berthelet E , Tran E , et al. Radiotherapy versus transoral robotic surgery and neck dissection for oropharyngeal squamous cell carcinoma (ORATOR): an open-label, phase 2, randomised trial . Lancet Oncol 2019 ; 20 : 1349 – 59 .
Farooq S , Khandavilli S , Dretzke J , Moore D , Nankivell PC , Sharma N , et al. Transoral tongue base mucosectomy for the identification of the primary site in the work-up of cancers of unknown origin: systematic review and meta-analysis . Oral Oncol 2019 ; 91 : 97 – 106 .
Fu TS , Foreman A , Goldstein DP , de Almeida JR . The role of transoral robotic surgery, transoral laser microsurgery, and lingual tonsillectomy in the identification of head and neck squamous cell carcinoma of unknown primary origin: a systematic review . J Otolaryngol Head Neck Surg 2016 ; 45 : 1 – 10 .
Gorphe P . A contemporary review of evidence for transoral robotic surgery in laryngeal cancer . Front Oncol 2018 ; 8 : 121 .
De Virgilio A , Costantino A , Mercante G , Di Maio P , Iocca O , Spriano G . Trans-oral robotic surgery in the management of parapharyngeal space tumors: a systematic review . Oral Oncol 2020 ; 103 :104581.
Gazda P , Gauche C , Chaltiel L , Chabrillac E , Vairel B , De Bonnecaze G , et al. Functional and oncological outcomes of salvage transoral robotic surgery: a comparative study . Eur Arch Otorhinolaryngol 2021 ; 1 – 10 .
Chalmers R , Schlabe J , Yeung E , Kerawala C , Cascarini L , Paleri V . Robot-assisted reconstruction in head and neck surgical oncology: the evolving role of the reconstructive microsurgeon . ORL J Otorhinolaryngol Relat Spec 2018 ; 80 : 178 – 85 .
Meccariello G , Cammaroto G , Montevecchi F , Hoff PT , Spector ME , Negm H , et al. Transoral robotic surgery for the management of obstructive sleep apnea: a systematic review and meta-analysis . Eur Arch Otorhinolaryngol 2017 ; 274 : 647 – 53 .
Chen AF , Kazarian GS , Jessop GW , Makhdom A . Robotic technology in orthopaedic surgery . J Bone Joint Surg 2018 ; 100 : 1984 – 92 .
Kayani B , Konan S , Tahmassebi J , Pietrzak J , Haddad F . Robotic-arm assisted total knee arthroplasty is associated with improved early functional recovery and reduced time to hospital discharge compared with conventional jig-based total knee arthroplasty: a prospective cohort study . Bone Joint J 2018 ; 100 : 930 – 7 .
Kayani B , Tahmassebi J , Ayuob A , Konan S , Oussedik S , Haddad FS . A prospective randomized controlled trial comparing the systemic inflammatory response in conventional jig-based total knee arthroplasty versus robotic-arm assisted total knee arthroplasty . Bone Joint J 2021 ; 103 : 113 – 22 .
Parsons H , Smith T , Rees S , Fox J , Grant N , Hutchinson C , et al. Robotic Arthroplasty: a Clinical and cost Effectiveness Randomised controlled trial. (RACER) . Southampton: National Institute for Health Research Evaluation, Trials and Studies Coordinating Centre (NETSCC) 2020 . URL: https://www.journalslibrary.nihr.ac.uk/programmes/hta/NIHR128768/ .
Karthik K , Colegate-Stone T , Dasgupta P , Tavakkolizadeh A , Sinha J . Robotic surgery in trauma and orthopaedics: a systematic review . Bone Joint J 2015 ; 97 : 292 – 9 .
Tewari A , Sooriakumaran P , Bloch DA , Seshadri-Kreaden U , Hebert AE , Wiklund P . Positive surgical margin and perioperative complication rates of primary surgical treatments for prostate cancer: a systematic review and meta-analysis comparing retropubic, laparoscopic, and robotic prostatectomy . Eur Urol 2012 ; 62 : 1 – 15 .
Bravi CA , Larcher A , Capitanio U , Mari A , Antonelli A , Artibani W , et al. Perioperative outcomes of open, laparoscopic, and robotic partial nephrectomy: a prospective multicenter observational study (The RECORd 2 Project) . Eur Urol Focus 2021 ; 7 : 390 – 6 .
Varghese A , Doglioli M , Fader AN . Updates and controversies of robotic-assisted surgery in gynecologic surgery . Clin Obstet Gynecol 2019 ; 62 : 733 .
Zanagnolo V , Garbi A , Achilarre MT , Minig L . Robot-assisted surgery in gynecologic cancers . J Minim Invasive Gynecol 2017 ; 24 : 379 – 96 .
Month: | Total Views: |
---|---|
February 2022 | 28 |
March 2022 | 100 |
April 2022 | 122 |
May 2022 | 145 |
June 2022 | 124 |
July 2022 | 124 |
August 2022 | 182 |
September 2022 | 289 |
October 2022 | 485 |
November 2022 | 428 |
December 2022 | 320 |
January 2023 | 330 |
February 2023 | 357 |
March 2023 | 509 |
April 2023 | 588 |
May 2023 | 533 |
June 2023 | 420 |
July 2023 | 427 |
August 2023 | 383 |
September 2023 | 554 |
October 2023 | 688 |
November 2023 | 1,027 |
December 2023 | 648 |
January 2024 | 645 |
February 2024 | 639 |
March 2024 | 561 |
April 2024 | 486 |
May 2024 | 601 |
June 2024 | 276 |
Email alerts
Citing articles via.
- Advertising and Corporate Services
- Journals Career Network
- JSPRM Twitter
Affiliations
- Online ISSN 2752-616X
- Copyright © 2024 Oxford University Press and JSCR Publishing Ltd
- About Oxford Academic
- Publish journals with us
- University press partners
- What we publish
- New features
- Open access
- Institutional account management
- Rights and permissions
- Get help with access
- Accessibility
- Advertising
- Media enquiries
- Oxford University Press
- Oxford Languages
- University of Oxford
Oxford University Press is a department of the University of Oxford. It furthers the University's objective of excellence in research, scholarship, and education by publishing worldwide
- Copyright © 2024 Oxford University Press
- Cookie settings
- Cookie policy
- Privacy policy
- Legal notice
This Feature Is Available To Subscribers Only
Sign In or Create an Account
This PDF is available to Subscribers Only
For full access to this pdf, sign in to an existing account, or purchase an annual subscription.

An official website of the United States government
The .gov means it’s official. Federal government websites often end in .gov or .mil. Before sharing sensitive information, make sure you’re on a federal government site.
The site is secure. The https:// ensures that you are connecting to the official website and that any information you provide is encrypted and transmitted securely.
- Publications
- Account settings
- My Bibliography
- Collections
- Citation manager
Save citation to file
Email citation, add to collections.
- Create a new collection
- Add to an existing collection
Add to My Bibliography
Your saved search, create a file for external citation management software, your rss feed.
- Search in PubMed
- Search in NLM Catalog
- Add to Search
A systematic review of robotic surgery: From supervised paradigms to fully autonomous robotic approaches
Affiliations.
- 1 Hamlyn Centre for Robotic Surgery and Artificial Intelligence, Imperial College London, London, UK.
- 2 National Hospital for Neurology and Neurosurgery, London, UK.
- PMID: 34953033
- DOI: 10.1002/rcs.2358
Background: From traditional open surgery to laparoscopic surgery and robot-assisted surgery, advances in robotics, machine learning, and imaging are pushing the surgical approach to-wards better clinical outcomes. Pre-clinical and clinical evidence suggests that automation may standardise techniques, increase efficiency, and reduce clinical complications.
Methods: A PRISMA-guided search was conducted across PubMed and OVID.
Results: Of the 89 screened articles, 51 met the inclusion criteria, with 10 included in the final review. Automatic data segmentation, trajectory planning, intra-operative registration, trajectory drilling, and soft tissue robotic surgery were discussed.
Conclusion: Although automated surgical systems remain conceptual, several research groups have developed supervised autonomous robotic surgical systems with increasing consideration for ethico-legal issues for automation. Automation paves the way for precision surgery and improved safety and opens new possibilities for deploying more robust artificial intelligence models, better imaging modalities and robotics to improve clinical outcomes.
Keywords: robotic assisted surgery; robotic autonomy; supervised autonomous robotic surgery.
© 2021 John Wiley & Sons Ltd.
PubMed Disclaimer
Similar articles
- Evaluation of objective tools and artificial intelligence in robotic surgery technical skills assessment: a systematic review. Boal MWE, Anastasiou D, Tesfai F, Ghamrawi W, Mazomenos E, Curtis N, Collins JW, Sridhar A, Kelly J, Stoyanov D, Francis NK. Boal MWE, et al. Br J Surg. 2024 Jan 3;111(1):znad331. doi: 10.1093/bjs/znad331. Br J Surg. 2024. PMID: 37951600 Free PMC article.
- The evolution of image guidance in robotic-assisted laparoscopic prostatectomy (RALP): a glimpse into the future. Makary J, van Diepen DC, Arianayagam R, McClintock G, Fallot J, Leslie S, Thanigasalam R. Makary J, et al. J Robot Surg. 2022 Aug;16(4):765-774. doi: 10.1007/s11701-021-01305-5. Epub 2021 Sep 4. J Robot Surg. 2022. PMID: 34480674 Review.
- Rethinking Autonomous Surgery: Focusing on Enhancement over Autonomy. Battaglia E, Boehm J, Zheng Y, Jamieson AR, Gahan J, Majewicz Fey A. Battaglia E, et al. Eur Urol Focus. 2021 Jul;7(4):696-705. doi: 10.1016/j.euf.2021.06.009. Epub 2021 Jul 8. Eur Urol Focus. 2021. PMID: 34246619 Free PMC article. Review.
- Artificial intelligence and robotics: a combination that is changing the operating room. Andras I, Mazzone E, van Leeuwen FWB, De Naeyer G, van Oosterom MN, Beato S, Buckle T, O'Sullivan S, van Leeuwen PJ, Beulens A, Crisan N, D'Hondt F, Schatteman P, van Der Poel H, Dell'Oglio P, Mottrie A. Andras I, et al. World J Urol. 2020 Oct;38(10):2359-2366. doi: 10.1007/s00345-019-03037-6. Epub 2019 Nov 27. World J Urol. 2020. PMID: 31776737 Review.
- Operational framework and training standard requirements for AI-empowered robotic surgery. O'Sullivan S, Leonard S, Holzinger A, Allen C, Battaglia F, Nevejans N, van Leeuwen FWB, Sajid MI, Friebe M, Ashrafian H, Heinsen H, Wichmann D, Hartnett M, Gallagher AG. O'Sullivan S, et al. Int J Med Robot. 2020 Oct;16(5):1-13. doi: 10.1002/rcs.2020. Epub 2020 Jun 8. Int J Med Robot. 2020. PMID: 31144777 Review.
- Artificial Intelligence (AI)-Robotics Started When Human Capability Reached Limit, Human Creativity Begin Again When the Capability of AI-Robotics Reaches a Plateau. Yi S. Yi S. Neurospine. 2024 Mar;21(1):3-5. doi: 10.14245/ns.2448234.117. Epub 2024 Mar 31. Neurospine. 2024. PMID: 38569625 Free PMC article. No abstract available.
- What are the key stability challenges in high-bandwidth, non-minimum phase systems with time-varying, and non-smooth delays? Weiwei T, Shaohui W, Sabzevari K. Weiwei T, et al. Heliyon. 2024 Mar 7;10(6):e26949. doi: 10.1016/j.heliyon.2024.e26949. eCollection 2024 Mar 30. Heliyon. 2024. PMID: 38509937 Free PMC article.
- Autonomous Magnetic Navigation in Endoscopic Image Mosaics. Mattille M, Boehler Q, Lussi J, Ochsenbein N, Moehrlen U, Nelson BJ. Mattille M, et al. Adv Sci (Weinh). 2024 May;11(19):e2400980. doi: 10.1002/advs.202400980. Epub 2024 Mar 14. Adv Sci (Weinh). 2024. PMID: 38482737 Free PMC article.
- Robotic assisted minimally invasive esophagectomy versus minimally invasive esophagectomy. Xue M, Liu J, Lu M, Zhang H, Liu W, Tian H. Xue M, et al. Front Oncol. 2024 Jan 15;13:1293645. doi: 10.3389/fonc.2023.1293645. eCollection 2023. Front Oncol. 2024. PMID: 38288099 Free PMC article.
- Review of Enhanced Handheld Surgical Drills. Usevitch DE, Bronheim RS, Cartagena-Reyes MA, Ortiz-Babilonia C, Margalit A, Jain A, Armand M. Usevitch DE, et al. Crit Rev Biomed Eng. 2023;51(6):29-50. doi: 10.1615/CritRevBiomedEng.2023049106. Crit Rev Biomed Eng. 2023. PMID: 37824333 Free PMC article. Review.
- Tonutti M, Elson DS, Yang GZ, Darzi AW, Sodergren MH. The role of technology in minimally invasive surgery: state of the art, recent developments and future directions. Postgrad Med J. 2017;93(1097):159-167.
- Smith ZA, Fessler RG. Paradigm changes in spine surgery-evolution of minimally invasive techniques. Nat Rev Neurol. 2012;8(8):443-450.
- Ashrafian H, Clancy O, Grover V, Darzi A. The evolution of robotic surgery: surgical and anaesthetic aspects. Br J Anaesth. 2017;119(suppl_1):i72-i84.
- Leonard S, Wu KL, Kim Y, Krieger A, Kim PC. Smart tissue anastomosis robot (STAR): a vision-guided robotics system for laparoscopic suturing. IEEE Trans Biomed Eng. 2014;61(4):1305-1317.
- Tsui C, Klein R, Garabrant M. Minimally invasive surgery: national trends in adoption and future directions for hospital strategy. Surg Endosc. 2013;27(7):2253-2257.
Publication types
- Search in MeSH
Grants and funding
- 208857/Z/17/Z/WT_/Wellcome Trust/United Kingdom
LinkOut - more resources
Full text sources.
- Ovid Technologies, Inc.
Miscellaneous
- NCI CPTAC Assay Portal
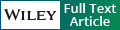
- Citation Manager
NCBI Literature Resources
MeSH PMC Bookshelf Disclaimer
The PubMed wordmark and PubMed logo are registered trademarks of the U.S. Department of Health and Human Services (HHS). Unauthorized use of these marks is strictly prohibited.
Thank you for visiting nature.com. You are using a browser version with limited support for CSS. To obtain the best experience, we recommend you use a more up to date browser (or turn off compatibility mode in Internet Explorer). In the meantime, to ensure continued support, we are displaying the site without styles and JavaScript.
- View all journals
- Explore content
- About the journal
- Publish with us
- Sign up for alerts
- Review Article
- Open access
- Published: 08 April 2024
The evolution of robotics: research and application progress of dental implant robotic systems
- Chen Liu ORCID: orcid.org/0009-0000-1771-5430 1 , 2 , 3 , 4 na1 ,
- Yuchen Liu 1 , 2 , 3 , 4 na1 ,
- Rui Xie 1 , 2 , 3 , 4 ,
- Zhiwen Li 1 , 2 , 3 , 4 ,
- Shizhu Bai ORCID: orcid.org/0000-0002-2439-3211 1 , 2 , 3 , 4 &
- Yimin Zhao 1 , 2 , 3 , 4
International Journal of Oral Science volume 16 , Article number: 28 ( 2024 ) Cite this article
1979 Accesses
1 Citations
17 Altmetric
Metrics details
- Medical research
- Oral diseases
- Preclinical research
The use of robots to augment human capabilities and assist in work has long been an aspiration. Robotics has been developing since the 1960s when the first industrial robot was introduced. As technology has advanced, robotic-assisted surgery has shown numerous advantages, including more precision, efficiency, minimal invasiveness, and safety than is possible with conventional techniques, which are research hotspots and cutting-edge trends. This article reviewed the history of medical robot development and seminal research papers about current research progress. Taking the autonomous dental implant robotic system as an example, the advantages and prospects of medical robotic systems would be discussed which would provide a reference for future research.
Similar content being viewed by others
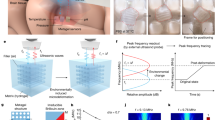
Injectable ultrasonic sensor for wireless monitoring of intracranial signals
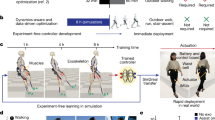
Experiment-free exoskeleton assistance via learning in simulation
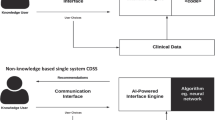
An overview of clinical decision support systems: benefits, risks, and strategies for success
The development of medical robots has been a long journey of exploration. After being practically validated in industrial robots, this technology has become widespread globally and is now an essential part of modern production and lifestyles. Medical robots are increasingly in the vanguard of the field in diagnosis, treatment, visualization, and other areas of clinical practice. We are currently witnessing a transformative shift from cutting-edge research to the widespread application of medical robots. This review focused on the historical trajectory of medical robots, with a particular emphasis on the development history, current research status, and prospects of dental implant robotic systems.
Definition and history of robots
Definition and architectures of robots.
According to the International Organization for Standardization (ISO), a robot is an automatic, position-controlled, programmable multi-functional manipulator with several axes. It can process various materials, parts, tools, and special devices through programmable automation to perform intended tasks. 1 A robot’s structure typically consists of four parts: the actuation system, the drive-transmission system, the control system, and the intelligent system. The actuation system is the part of the robot that directly performs work, similar to a human hand. The drive-transmission system transmits force and motion to the actuator through a power source. The control system comprises a control computer, control software, and servo controllers, similar to a human brain. The intelligent system typically includes a perception system and an analytical decision-making intelligent system.
Evolution of robots
The history of robots can be traced back over 3 000 years. 2 Throughout history, scientists and craftsmen have designed and manufactured robot prototypes that simulate animal or human characteristics. 1 However, these inventions can only be classified as mechanical devices that primarily achieved automated functions through mechanical and physical principles with the lack of intelligence and autonomy of modern robots. These inventions demonstrate the level of engineering technology and mechanical manufacturing in ancient times, laying the foundation for later research on robots. Joseph Engelberger, recognized as the Father of Robotics, founded Unimation Corporation in 1958, the world’s first robot-manufacturing factory, which marked the official start of the industrialization of robots. In 1978, Unimation developed a Programmable Universal Machine for Assembly (PUMA) which represents a significant milestone in the development of international industrial robotics. In recent years, robotics has expanded significantly due to the continued development of sensor types, intelligent algorithms, and multidisciplinary integration. The technology has advanced from the initial industrial robotic arms to bionic robots, soft robots, nanorobots, and other forms.
Classification of robotics
The International Federation of Robotics (IFR) classifies robotics into two distinct categories: industrial robotics and service robotics, in accordance with the international standard ISO 8373:2012. 3 Industrial robotics are multipurpose manipulators with automatic control and programmability, which can operate with fixed or autonomous mobility and are primarily used in industrial production. 3 Service robotics are driving mechanisms that can perform useful tasks but do not include industrial automation applications. The IFR has classified service robotics into different segments to meet the diverse requirements of various industries (Fig. 1 ).
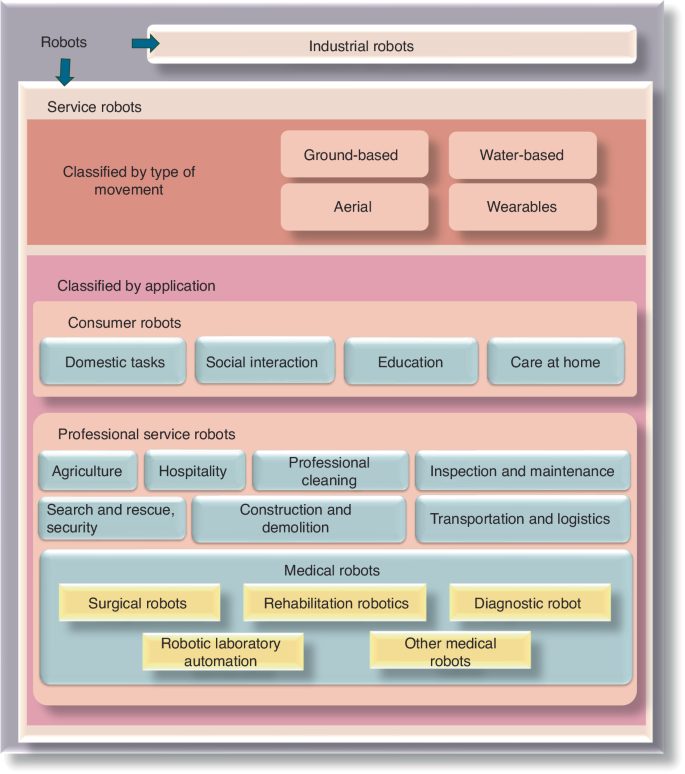
Categories of robots according to the International Federation of Robotics
Medical robotics
In 1985, the Puma 200 robot (Westinghouse Electric, Pittsburgh, PA) was used for needle placement in computed tomography (CT)-guided brain biopsy at the Los Angeles Hospital in the United States, marking the beginning of the era of medical robot applications. 4 , 5 After nearly 40 years of continuous development and progress, medical robotics have been widely used in multiple fields, including surgery, nursing, and rehabilitation, demonstrating numerous remarkable advantages and potential.
Yang 6 , 7 , 8 has divided the level of autonomy of medical robotics into six levels, as follows: (0) no autonomy, (1) robot assistance, (2) task autonomy, (3) conditional autonomy, (4) high autonomy, and (5) full autonomy. At level 0, the robot requires operators to perform all tasks, including monitoring, generating performance options, selecting the option to perform (decision making), and executing the decision made, such as the da Vinci robotic system (Intuitive Inc., California, USA). At level 1, operators are required to continuously control the robot while the robot provides guidance with positional constraints. The Mako Smart Robotics used in orthopedic surgery is an example. At level 2, operators are required to discretely rather than continuously control the robot, and the robot can independently complete specific tasks based on operator instructions and pre-programmed procedures. An example of this level is the ROBODOC, which performs total hip and total knee replacement surgeries. At level 3, robots have the ability to perform surgeries based on pre-programmed procedures and can also modify the pre-planned schedule in real time to accommodate changes in the intraoperative position of the target object. An example of such robotics is the CyberKnife radiation therapy robotics, which has respiratory tracking functionality. At the higher levels of autonomy (specifically level 5 and possibly level 4), the robot is not only a medical device but also capable of practicing medicine, which currently does not exist due to some regulatory, ethical, and legal considerations. 6 , 7 , 8
Medical robotics are classified by IFR as special robotics with a combination of medical diagnosis methods with new technologies, such as artificial intelligence (AI) and big data, to provide services such as surgery, rehabilitation, nursing, medical transportation, and consultation for patients. 9 Medical robotics are categorized into the following five types based on their functions: surgical robotics, rehabilitation robotics, diagnostic robotics, laboratory analysis automation, and other robotics (robotics used for medical transportation are not included in this category).
Surgical robotics
Minimally invasive surgery and accurate intervention require surgeons to exercise more discernment, expand their range of vision, and increase their flexibility which brings the surgical robotics development (the surgical robot architecture 10 was shown in Fig. 2 ). Not only can it be equipped with an advanced three-dimensional (3D) imaging system and augmented reality technology to provide high-definition images of the surgical scene, but it is also capable of displaying important anatomical structures such as blood vessel and nerve locations in real-time. This allows surgeons to perform precise operations with the assistance of robots. For higher-level automatic medical robots, precise surgical operations are performed through image guidance and navigation systems based on preoperative planning. Moreover, the robotic arm has a high level of precision and stability that surpasses the capabilities of a free hand. This allows it to perform small and delicate operations with reduced errors caused by physician experience, fatigue, and hand tremors. In addition, the surgical robot also integrates artificial intelligence technology, which can perform automatic diagnostic analysis, adjust surgical strategies, and provide personalized surgical plans through deep learning. 11 Therefore, surgical robots could utilize vision, speech recognition, telecommunication, 3D imaging, and artificial intelligence technologies to enhance surgical skills through sensing and image guidance systems. This overcomes the limitations of manual operations and improves surgical accuracy and reliability. In comparison to conventional surgery, robotic-assisted surgery could reduce trauma, shorten recovery periods, and relieve pain. 12 , 13 Additionally, it can be used for remote surgery, operates continuously without fatigue, reduces the workload of medical staff, and minimizes occupational exposure for surgeons. Medical robotics have gradually entered the commercialization stage and have been utilized in clinical settings (Table 1 ). Currently, the most well-known surgical robot is the da Vinci system, which enables surgeons to accurately and minimally perform invasive surgery for multiple complicated diseases with good hand-eye coordination and magnification.
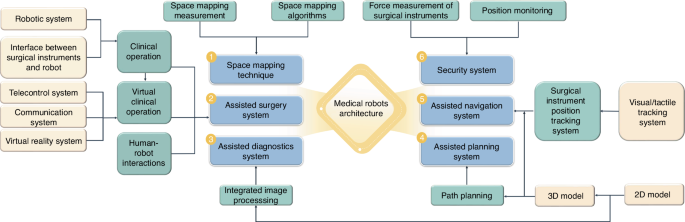
The surgical robotic architecture
Dental treatment involves the special anatomical structure of the mouth and is characterized by limited visibility, narrow operation space, and the disturbance of saliva and tongue. As a result, the dental operation is intricate and mainly reliant on the surgeon’s experience and expertize, which takes inexperienced surgeons a long time to acquire. With the successful use of the da Vinci robotic system in laparoscopic surgery, surgeons are beginning to consider its potential application in maxillofacial surgery. Da Vinci robot has been used for cleft palate repair, 14 , 15 treating patients with obstructive sleep apnea-hypopnea syndrome (OSAHS), 16 as well as oral and oropharyngeal tumor resection. 17 , 18 However, due to the complexity of the oropharyngeal anatomy, the multiple robotic arms of the da Vinci system limit the surgeon’s vision, which is not conducive to surgical performance. In order to overcome these shortcomings, flexible robots (such as The Flex) approved by the Food and Drug Administration have made it possible to be used for oropharyngeal surgery. Additionally, oral and cranio-maxillofacial bone surgery, such as orthognathic surgery and dental implant surgery, requires accurate ostomies, which cannot be achieved by the da Vinci system. Robotic-assisted dental implant surgery research originated in 2001, and related studies have shown a gradual increase in recent years. In addition to conventional implant surgery, dental implant robotics can also perform zygomatic implant placement. 19 , 20 Among these studies, the largest number of articles were published in China, followed by the United States (Fig. 3 ). In Part 3 of this article, the relevant studies on dental implant robotics will be elaborated in detail.
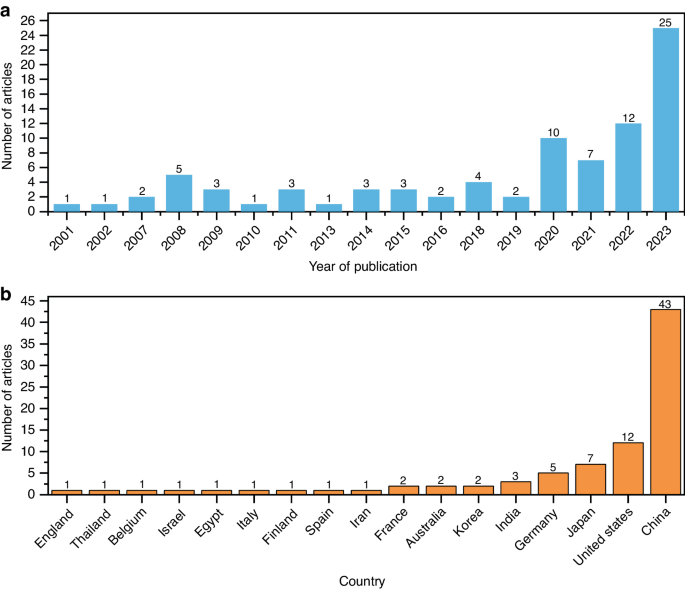
Related research on robotic-assisted dental implant placement. a The number of published papers on dental implant robotics in different years and b in different countries (as of December 2023)
Rehabilitation robotics
Rehabilitation robotics are significant area and research hotspot in medical robotics, second only to surgical robotics. Rehabilitation robotics are classified into two categories, as follows: therapeutic and assistive robotics. Therapeutic robotics provide psychological or physical treatment to improve specific functions of patients and are widely used in physical training and functional recovery of patients with paralysis and in improving the interactive ability of children with autism through behavioral induction. 21 Assistive robotics aim to improve the quality of life for individuals with musculoskeletal or neuromuscular impairments by compensating for or replacing their mobility or functionality. 22 , 23 , 24 For instance, Mike Topping’s Handy1 assists the most severely disabled with several everyday functions. 25 Similarly, Israel’s ReWalk provides powered hip and knee motion to enable individuals with spinal cord injury to stand upright, walk, turn, climb, and descend stairs. 26 Moreover, Japan’s wearable powered prosthesis, HAL, can enable patients to control joint movements independently by detecting bioelectrical signals on the skin surface during movement, in combination with foot pressure sensors. 27
Diagnostic robotics
Diagnostic robotics aid doctors in conducting examinations and making diagnoses, with the aim of improving accuracy, convenience, non-invasiveness, and safety of diagnosis. For instance, wireless capsule endoscopy introduced by Given Imaging (now Medtronic) allows minimally invasive inspection of the gastrointestinal tract. Patients can swallow a pillcam that captures images deep within the intestines, which has revolutionized gastrointestinal endoscopy and is now a clinically viable alternative to standard interventional endoscopy. Furthermore, wearable robotics are increasingly being utilized to non-invasively detect various health indicators and assist in disease diagnosis.
Laboratory robotics
Laboratory robotics handle and analyze samples in medical laboratories. Innovations in robotics and information technologies have created new opportunities for laboratory automation. These robots tirelessly and accurately perform tasks, improving the precision and reliability of experiments while reducing costs. At the University of Virginia Medical Center, robots operate instruments and analyze blood gases and electrolytes in the hospital laboratory. In addition, the robotic system works continuously, not only improving laboratory efficiency but also reducing the burden on laboratory techniques. 28 Nicole Rupp, based in Germany, has utilized the Dobot Magician robot to develop an economical automated laboratory system that coordinates various instruments for experiments. The results obtained from this system were not statistically different from those obtained from manual experiments. 29
Other medical robotics
The medical field has witnessed a significant increase in the use of robotics, leading to the development of new types of robots and functions to cater to the requirements of doctors and patients. Other medical robotics include providing non-medical operational services, such as assisting nurses with guidance, transportation, cleaning, inspection, monitoring, and disinfection. Moreover, robotics could be available for daily home care, providing assistance, monitoring behavior and health, as well as offering companionship for older individuals. 30 Furthermore, there are robots specifically designed to train emergency personnel. These robots can simulate complex trauma scenarios with multiple injuries in a highly accurate manner. 31 Robotic surgery simulation practice can be combined with virtual reality (VR), 3D-printed organ tissue models, or anesthetized live animals to rapidly improve the robotic surgical skills required by novice surgeons. In addition, to pandemics such as Ebola and COVID-19, the use of sampling robotics can effectively reduce the risk of infection. There are robots also designed for emergency rescue, medical education, and training. 32 , 33 Soft robotics, bionic robotics, nanorobots, and other robotics suitable for various functional needs are also hot topics in current medical robotic research, and they exhibit the typical characteristics of specialization, personalization, remoteness, intelligence, and immersion.
Dental implant robotic system
Implantology is widely considered the preferred treatment for patients with partial or complete edentulous arches. 34 , 35 The success of the surgery in achieving good esthetic and functional outcomes is directly related to correct and prosthetically-driven implant placement. 36 Accurate implant placement is crucial to avoid potential complications such as excessive lateral forces, prosthetic misalignment, food impaction, secondary bone resorption, and peri-implantitis. 37 Any deviation during the implant placement can result in damage to the surrounding blood vessels, nerves, and adjacent tooth roots and even cause sinus perforation. 38 Therefore, preoperative planning must be implemented intraoperatively with utmost precision to ensure quality and minimize intraoperative and postoperative side effects. 39
Currently, implant treatment approaches are as follows: Free-handed implant placement, Static computer-aided implant placement, and dynamic computer-aided implant placement. The widely used free-handed implant placement provides less predictable accuracy and depends on the surgeon’s experience and expertise. 40 Deviation in implant placement is relatively large among surgeons with different levels of experience. When novice surgeons face complex cases, achieving satisfactory results can be challenging. A systematic review 41 based on six clinical studies indicated that the ranges of deviation of the platform, apex, and angle from the planned position with free-handed implant placement were (1.25 ± 0.62) mm–(2.77 ± 1.54) mm, (2.10 ± 1.00) mm–(2.91 ± 1.52) mm, and 6.90°± 4.40°–9.92°± 6.01°, respectively. Static guides could only provide accurate guidance for the initial implantation position. However, it is difficult to precisely control the depth and angle of osteotomies. 42 The lack of real-time feedback on drill positioning during surgery can limit the clinician’s ability to obtain necessary information. 42 , 43 , 44 Besides, surgical guides may also inhibit the cooling of the drills used for implant bed preparation, which may result in necrosis of the overheated bone. Moreover, the use of static guides is limited in patients with limited accessibility, especially for those with implants placed in the posterior area. Additionally, the use of guides cannot flexibly adjust the implant plan intraoperatively. With dynamic computer-aided implant placement, the positions of the patient and drills could be tracked in real-time and displayed on a computer screen along with the surgical plan, thus allowing the surgeon to adjust the drilling path if necessary. However, the surgeons may deviate from the plan or prepare beyond it without physical constraints. During surgery, the surgeon may focus more on the screen for visual information rather than the surgical site, which can lead to reduced tactile feedback. 45 The results of a meta-analysis showed that the platform deviation, apex deviation, and angular deviation were 0.91 mm (95% CI 0.79–1.03 mm), 1.26 mm (95% CI 1.14–1.38 mm), and 3.25° (95% CI 2.84°–3.66°) respectively with the static computer-aided implant placement, and 1.28 mm (95% CI 0.87–1.69 mm), 1.68 mm (95% CI 1.45–1.90 mm), and 3.79° (95% CI 1.87–5.70°), respectively, with dynamic computer-aided implant placement. The analysis results showed that both methods improved the accuracy compared to free-handed implant placement, but they still did not achieve ideal accuracy. 46 Gwangho et al. 47 believe that the key point of a surgical operation is still manually completed by surgeons, regardless of static guide or dynamic navigation, and the human factors (such as hand tremble, fatigue, and unskilled operation techniques) also affect the accuracy of implant placement.
Robotic-assisted implant surgery could provide accurate implant placement and help the surgeon control handpieces to avoid dangerous tool excursions during surgery. 48 Furthermore, compared to manual calibration, registration, and surgery execution, automatic calibration, registration, and drilling using the dental implant robotic system reduces human error factors. This, in turn, helps avoid deviations caused by surgeons’ factors, thereby enhancing surgical accuracy, safety, success rates, and efficiency while also reducing patient trauma. 7 With the continuous improvement of technology and reduction of costs, implant robotics are gradually becoming available for commercial use. Yomi (Neocis Inc., USA) has been approved by the Food and Drug Administration, while Yakebot (Yakebot Technology Co., Ltd., Beijing, China), Remebot (Baihui Weikang Technology Co., Ltd, Beijing, China), Cobot (Langyue dental surgery robot, Shecheng Co. Ltd., Shanghai, China), Theta (Hangzhou Jianjia robot Co., Ltd., Hangzhou, China), and Dcarer (Dcarer Medical Technology Co., Ltd, Suzhou, China) have been approved by the NMPA. Dencore (Lancet Robotics Co., Ltd., Hangzhou, China) is in the clinical trial stage in China.
Basic research on dental implant robotic system
Compared to other surgeries performed with general anesthesia, dental implant surgery can be completed under local anesthesia, with patients awake but unable to remain completely still throughout the entire procedure. Therefore, research related to dental implant robotic system, as one of the cutting-edge technologies, mainly focuses on acquiring intraoperative feedback information (including tactile and visual information), different surgical methods (automatic drilling and manual drilling), patient position following, and the simulation of surgeons’ tactile sensation.
Architecture of dental implant robotic system
The architecture of dental implant robotics primarily comprises the hardware utilized for surgical data acquisition and surgical execution (Fig. 4 ). Data acquisition involves perceiving, identifying, and understanding the surroundings and the information required for task execution through the encoders, tactile sensors, force sensors, and vision systems. Real-time information obtained also includes the robot’s surrounding environment, object positions, shapes, sizes, surface features, and other relevant information. The perception system assists the robot in comprehending its working environment and facilitates corresponding decision-making as well as actions.
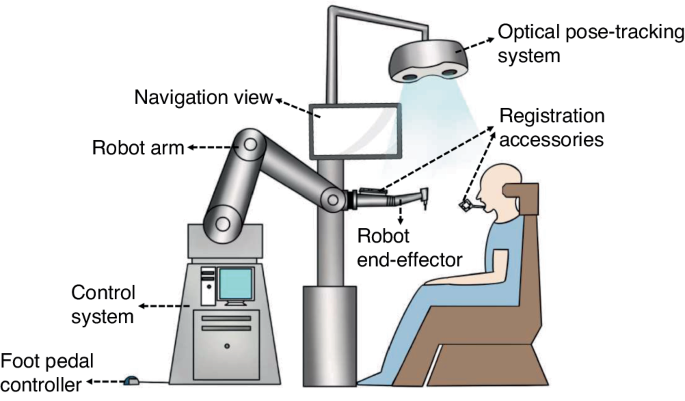
The architecture of dental implant robotics
During the initial stage of research on implant robotics, owing to the lack of sensory systems, fiducial markers and corresponding algorithms were used to calculate the transformation relationship between the robot’s and the model’s coordinate system. The robot was able to determine the actual position through coordinate conversions. Dutreuil et al. 49 proposed a new method for creating static guides on casts using robots based on the determined implant position. Subsequently, Boesecke et al. 50 developed a surgical planning method using linear interpolation between start and end points, as well as intermediate points. The surgeon performed the osteotomies by holding the handpieces, with the robot guidance based on preoperatively determined implant position. Sun et al. 51 and McKenzie et al. 52 registered cone-beam computed tomography (CBCT) images, the robot’s coordinate system, and the patient’s position using a coordinate measuring machine, which facilitated the transformation of preoperative implant planning into intraoperative actions.
Neocis has developed a dental implant robot system called Yomi (Neocis Inc.) 53 based on haptic perception and connects a mechanical joint measurement arm to the patient’s teeth to track their position. The joint encoder provides information on the drill position, while the haptic feedback of handpieces maneuvered by the surgeon constrains the direction and depth of implant placement.
Optical positioning is a commonly used localization method that offers high precision, a wide -field -of -view, and resistance to interference. 54 This makes it capable of providing accurate surgical guidance for robotics. Yu et al. 55 combined image-guided technology with robotic systems. They used a binocular camera to capture two images of the same target, extract pixel positions, and employ triangulation to obtain three-dimensional coordinates. This enabled perception of the relative positional relationship between the end-effector and the surrounding environment. Yeotikar et al. 56 suggested mounting a camera on the end-effector of the robotic arm, positioned as close to the drill as possible. By aligning the camera’s center with the drill’s line of sight at a specific height on the lower jaw surface, the camera’s center accurately aligns with the drill’s position in a two-dimensional space at a fixed height from the lower jaw. This alignment guides the robotic arm in drilling through specific anatomical landmarks in the oral cavity. Yan et al. 57 proposed that the use of “eye-in-hand” optical navigation systems during surgery may introduce errors when changing the handpiece at the end of the robotic arm. Additionally, owing to the narrow oral environment, customized markers may fall outside the camera’s field of view when the robotic arm moves to certain positions. 42 To tackle this problem, a dental implant robot system based on optical marker spatial registration and probe positioning strategies is designed. Zhao et al constructed a modular implant robotic system based on binocular visual navigation devices operating on the principles of visible light with “eye-to-hand” mode, allowing complete observation of markers and handpieces within the camera’s field of view, thereby ensuring greater flexibility and stability. 38 , 58
The dental implant robotics execution system comprises hardware such as motors, force sensors, actuators, controllers, and software components to perform tasks and actions during implant surgery. The system receives commands, controls the robot’s movements and behaviors, and executes the necessary tasks and actions. Presently, research on dental implant robotic systems primarily focuses on the mechanical arm structure and drilling methods.
The majority of dental implant robotic systems directly adopt serial-linked industrial robotic arms based on the successful application of industrial robots with the same robotic arm connection. 59 , 60 , 61 , 62 These studies not only establish implant robot platforms to validate implant accuracy and assess the influence of implant angles, depths, and diameters on initial stability but also simulate chewing processes and prepare natural root-shaped osteotomies based on volume decomposition. Presently, most dental implant robots in research employ a single robotic arm for surgery. Lai et al. 62 indicated that the stability of the handpieces during surgery and real-time feedback of patient movement are crucial factors affecting the accuracy of robot-assisted implant surgery. The former requires physical feedback, while the latter necessitates visual feedback. Hence, they employed a dual-arm robotic system where the main robotic arm was equipped with multi-axis force and torque sensors for performing osteotomies and implant placement. The auxiliary arm consisted of an infrared monocular probe used for visual system positioning to address visual occlusion issues arising from changes in arm angles during surgery.
The robots mentioned above use handpieces to execute osteotomies and implant placement. However, owing to limitations in patient mouth opening, performing osteotomies and placing implants in the posterior region can be challenging. To overcome the spatial constraints during osteotomies in implant surgery, Yuan et al. 63 proposed a robot system based on earlier research which is laser-assisted tooth preparation. This system involves a non-contact ultra-short pulse laser for preparing osteotomies. The preliminary findings confirmed the feasibility of robotically controlling ultra-short pulse lasers for osteotomies, introducing a novel method for a non-contact dental implant robotic system.
Position following of dental implant robotic system
It can be challenging for patients under local anesthesia to remain completely still during robot-assisted dental implant surgery. 52 , 64 , 65 , 66 , 67 Any significant micromovement in the patient’s position can severely affect clinical surgical outcomes, such as surgical efficiency, implant placement accuracy compared to the planned position, and patient safety. Intraoperative movement may necessitate re-registration for certain dental implant robotic systems. In order to guarantee safety and accuracy during surgery, the robot must detect any movement in the patient’s position and promptly adjust the position of the robotic arm in real time. Yakebot uses binocular vision to monitor visual markers placed outside the patient’s mouth and at the end of the robotic arm. This captures motion information and calculates relative position errors. The robot control system utilizes preoperatively planned positions, visual and force feedback, and robot kinematic models to calculate optimal control commands for guiding the robotic arm’s micromovements and tracking the patient’s micromovements during drilling. As the osteotomies are performed to the planned depth, the robotic arm compensates for the patient’s displacement through the position following the function. The Yakebot’s visual system continuously monitors the patient’s head movement in real time and issues control commands every 0.008 s. The robotic arm is capable of following the patient’s movements with a motion servo in just 0.2 s, ensuring precise and timely positioning.
The simulation of surgeons’ tactile sensation in dental implant robotic systems
Robot-assisted dental implant surgery requires the expertise and tactile sense of a surgeon to ensure accurate implantation. Experienced surgeons can perceive bone density through the resistance they feel in their hands and adjust the force magnitude or direction accordingly. This ensures proper drilling along the planned path. However, robotic systems lack perception and control, which may result in a preference for the bone side with lower density. This can lead to inaccurate positioning compared to the planned implant position. 61 , 62 Addressing this challenge, Li et al. 68 established force-deformation compensation curves in the X, Y, and Z directions for the robot’s end-effector based on the visual and force servo systems of the autonomous dental robotic system, Yakebot. Subsequently, a corresponding force-deformation compensation strategy was formulated for this robot, thus proving the effectiveness and accuracy of force and visual servo control through in vitro experiments. The implementation of this mixed control mode, which integrates visual and force servo systems, has improved the robot’s accuracy in implantation and ability to handle complex bone structures. Based on force and visual servo control systems, Chen et al. 69 have also explored the relationship between force sensing and the primary stability of implants placed using the Yakebot autonomous dental robotic system through an in vitro study. A significant correlation was found between Yakebot’s force sensing and the insertion torque of the implants. This correlation conforms to an interpretable mathematical model, which facilitates the predictable initial stability of the implants after placement.
During osteotomies with heat production (which is considered one of the leading causes of bone tissue injury), experienced surgeons could sense possible thermal exposure via their hand feeling. However, with free-handed implant placement surgery, it is challenging to perceive temperature changes during the surgical process and establish an effective temperature prediction model that relies solely on a surgeon’s tactile sense. Zhao et al. 70 , using the Yakebot robotic system, investigated the correlation between drilling-related mechanical data and heat production and established a clinically relevant surrogate for intraosseous temperature measurement using force/torque sensor-captured signals. They also established a real-time temperature prediction model based on real-time force sensor monitoring values. This model aims to effectively prevent the adverse effects of high temperatures on osseointegration, laying the foundation for the dental implant robotic system to autonomously control heat production and prevent bone damage during autonomous robotic implant surgery.
The innovative technologies mentioned above allow dental implant robotic systems to simulate the tactile sensation of a surgeon and even surpass the limitations of human experience. This advancement promises to address issues that free-handed implant placement techniques struggle to resolve. Moreover, this development indicates substantial progress and great potential for implantation.
Clinical research on dental implant robotic systems
Clinical workflow of dental implant robotic systems.
The robotic assistant dental implant surgery consists of three steps: preoperative planning, intraoperative phase, and postoperative phase (Fig. 5 ). For preoperative planning, it is necessary to obtain digital intraoral casts and CBCT data from the patient, which are then imported into preoperative planning software for 3D reconstruction and planning implant placement. For single or multiple tooth gaps using implant robotic systems (except Yakebot), 61 , 62 , 71 , 72 a universal registration device (such as the U-shaped tube) must be worn on the patients’ missing tooth site using a silicone impression material preoperatively to acquire CBCT data for registration. The software performs virtual placement of implant positions based on prosthetic and biological principles of implant surgery, taking into account the bone quality of the edentulous implant site to determine the drilling sequence, insertion depth of each drill, speed, and feed rate. For single or multiple tooth implants performed using Yakebot, there is no need for preoperative CBCT imaging with markers. However, it is necessary to design surgical accessories with registration holes, brackets for attaching visual markers, and devices for assisting mouth opening and suction within the software (Yakebot Technology Co., Ltd., Beijing, China). These accessories are manufactured using 3D printing technology.
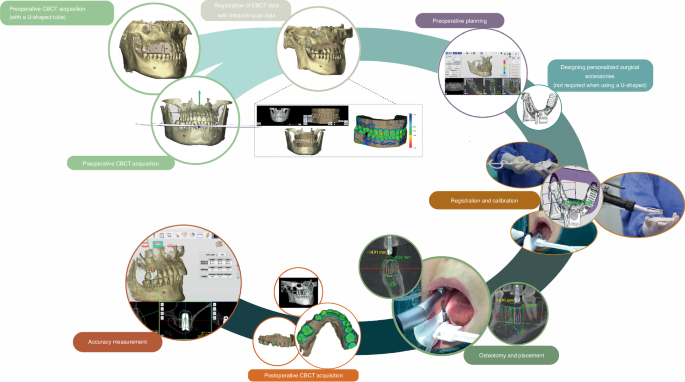
Clinical workflow of robotic-assisted dental implant placement
For the intraoperative phase, the first step is preoperative registration and calibration. For Yakebot, the end-effector marker is mounted to the robotic arm, and the spatial positions are recorded under the optical tracker. The calibration plate with the positioning points is then assembled into the implant handpiece for drill tip calibration. Then, the registration probe is inserted in the registration holes of the jaw positioning plate in turn for spatial registration of the jaw marker and the jaw. Robot-assisted dental implant surgery usually does not require flapped surgery, 73 , 74 , yet bone grafting due to insufficient bone volume in a single edentulous space or cases of complete edentulism requiring alveolar ridge preparation may require elevation of flaps. For full-arch robot-assisted implant surgery, a personalized template with a positioning marker is required and should be fixed with metallic pins for undergoing an intraoperative CBCT examination, thus facilitating the robot and the jaws registration in the visual space and allowing the surgical robot to track the patient’s motion. The safe deployment of a robot from the surgical site is an essential principle for robot-assisted implant surgery. In the case of most robots, such as Yomi, the surgeon needs to hold the handpieces to control and supervise the robot’s movement in real time and stop the robotic arm’s movement in case of any accidents. With Yakebot, the entire surgery is performed under the surgeon’s supervision, and immediate instructions are sent in response to possible emergencies via a foot pedal. Additionally, the recording of the entrance and exit of the patient’s mouth ensures that the instruments would not damage the patient’s surrounding tissues. The postoperative phase aims at postoperative CBCT acquisition and accuracy measurement.
In clinical surgical practice, robots with varying levels of autonomy perform implant surgeries differently. According to the autonomy levels classified by Yang et al. 6 , 8 , 33 for medical robots, commercial dental implant robotic systems (Table 2 ) currently operate at the level of robot assistance or task autonomy.
The robot-assistance dental implant robotic systems provide haptic, 75 visual or combined visual and tactile guidance during dental implant surgery. 46 , 76 , 77 Throughout the procedure, surgeons must maneuver handpieces attached to the robotic guidance arm and apply light force to prepare osteotomies. 62 The robotic arm constrains the 3D space of the drill as defined by the virtual plan, enabling surgeons to move the end of the mechanical arm horizontally or adjust its movement speed. However, during immediate implant placement or full-arch implant surgery, both surgeons and robots may struggle to accurately perceive poor bone quality, which should prompt adjustments at the time of implant placement. This can lead to incorrect final implant positions compared to the planned locations.
The task-autonomous dental implant robotic systems can autonomously perform partial surgical procedures, such as adjusting the position of the handpiece to the planned position and preparing the implant bed at a predetermined speed according to the pre-operative implant plan, and surgeons should send instructions, monitor the robot’s operation, and perform partial interventions as needed. For example, the Remebot 77 , 78 requires surgeons to drag the robotic arm into and out of the mouth during surgery, and the robot automatically performs osteotomies or places implants according to planned positions under the surgeon’s surveillance. The autonomous dental implant robot system, Yakebot, 73 , 79 , 80 can accurately reach the implant site and complete operations such as implant bed preparation and placement during surgery. It can be controlled by the surgeon using foot pedals and automatically stops drilling after reaching the termination position before returning to the initial position. Throughout the entire process, surgeons only need to send commands to the robot using foot pedals.
Clinical performance of robot-assisted implant surgery
Figure 6 shows the results of accuracy in vitro, in vivo, and clinical studies on robot-assisted implant surgery. 20 , 46 , 48 , 55 , 62 , 64 , 67 , 68 , 69 , 70 , 71 , 72 , 75 , 76 , 77 , 78 , 79 , 80 , 81 , 82 , 83 , 84 , 85 , 86 , 87 , 88 , 89 The results suggest that platform and apex deviation values are consistent across different studies. However, there are significant variations in angular deviations among different studies, which may be attributed to differences in the perception and responsiveness to bone quality variances among different robotic systems. Therefore, future development should focus on enhancing the autonomy of implant robots and improving their ability to recognize and respond to complex bone structures.
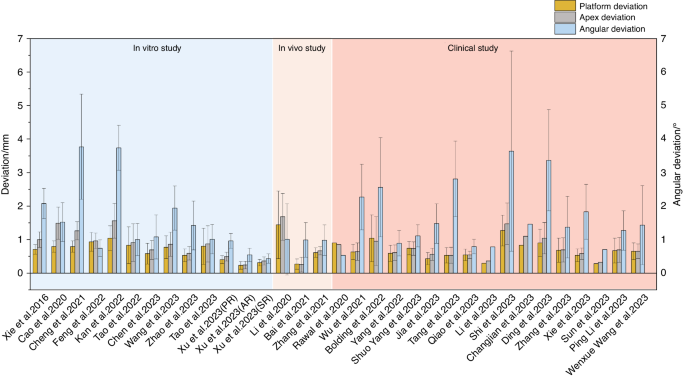
Accuracy reported in studies on robotic-assisted implant placement
Xu et al. 77 conducted a phantom experimental study comparing the implant placement accuracy in three levels of dental implant robotics, namely passive robot (Dcarer, level 1), semi-active robot (Remebot, level 2), and active robot (Yakebot, level 2) (Fig. 7 ). The study found that active robot had the lowest deviations at the platform and apex of the planned and actual implant positions, While the semi-active robot also had the lowest angular deviations. Chen et al. 46 and Jia et al. 79 conducted clinical trials of robotic implant surgery in partially edentulous patients using a semi-active dental implant robotic system (level 1) and an autonomous dental implant robot (level 2). The deviations of the implant platform, apex, and angle were (0.53 ± 0.23) mm/(0.43 ± 0.18) mm, (0.53 ± 0.24) mm/(0.56 ± 0.18) mm and 2.81° ± 1.13°/1.48° ± 0.59°, respectively. These results consistently confirmed that robotic systems can achieve higher implant accuracy than static guidance and that there is no significant correlation between accuracy and implant site (such as anterior or posterior site). The platform and angle deviation of autonomous dental implant robots were smaller than those of semi-active dental implant robotic systems. Li et al. 73 reported the use of the autonomous dental implant robot (level 2) to complete the placement of two adjacent implants with immediate postoperative restoration. The interim prosthesis fabricated prior to implant placement was seated without any adjustment, and no adverse reactions occurred during the operation.
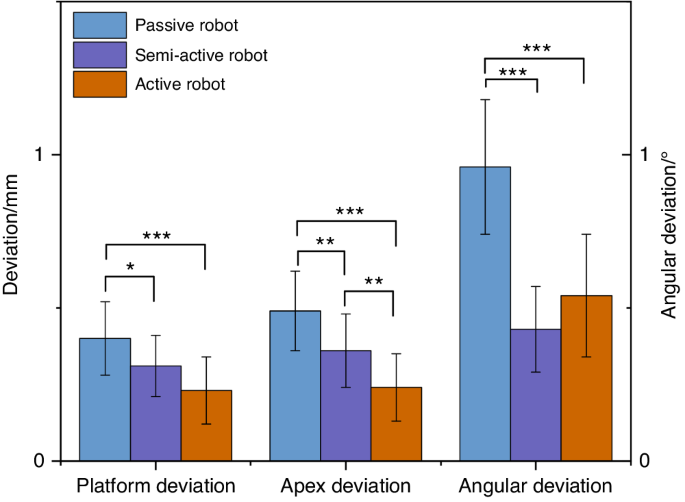
Comparison of accuracy of dental implant robotics with different levels of autonomy (phantom experiments) (* P < 0.05, ** P < 0.01, *** P < 0.001)
Bolding et al., 53 Li et al., 20 Jia et al., 79 and Xie et al. 90 used dental implant robots to conduct clinical trials in full-arch implant surgery with five or six implants placed in each jaw. The deviations of implant platform, apex, and angle are shown in Fig. 8 . The haptic dental implant robot (level 1) used by Bolding et al., 53 achieved more deviations compared to other studies that used semi-active (level 1) or active robots (level 2). As its handpiece must be maneuvered by the surgeon, human errors such as surgeon fatigue may not be avoided. Owing to the parallel common implant placement paths between various implant abutments, prefabricated temporary dentures could be seated smoothly, and some patients wore temporary complete dentures immediately after surgery. These results indicate that robotic systems can accurately locate and perform implant placement during surgery.

Comparison of accuracy in robotic-assisted full-arch implant placement
As there are relatively few studies of implant robots in clinical applications, Tak ́acs et al. 91 conducted a meta-analysis under in vitro conditions with free-handed, static-guided, dynamic navigated, and robotic-assisted implant placements, as shown in Fig. 9 . It was found that, compared to free-handed, static guided and dynamic navigated implant placements, robotic-assisted implant placements have more advantages in terms of accuracy. However, in vitro studies cannot fully simulate the patients’ oral condition and bone quality. Recent clinical studies 89 , 92 , 93 have shown a lower deviation in robotic-assisted implant placements compared to static-guided and dynamic-navigated implant placements. Common reasons for deviations in static-guided and dynamic-navigated implant placements include the following: deflection caused by hand tremors due to dense bone during surgery, surgeons’ experience, and other human factors. Larger clinical studies will be needed in the future to evaluate the differences between robotic and conventional surgical approaches and to provide guidance for the further development and refinement of robotic techniques.
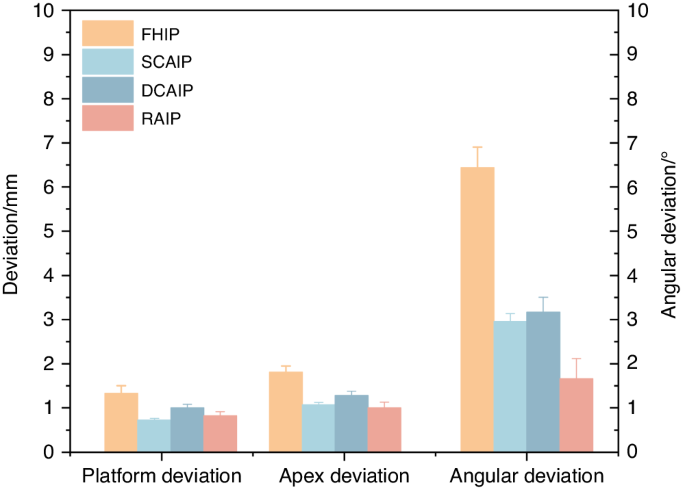
Comparison of accuracy of free-handed, static, dynamic, and robotic-assisted implant placement. (FHIP free-hand implant placement, SCAIP static computer-aided implant placement, DCAIP dynamic computer-aided implant placement, RAIP robot-assisted implant placement)
For the long-term follow-up performance of robotic systems used in dental implant procedures, none of the comparative studies was longer than a year. One 1-year prospective clinical study by Xie et al. 90 showed that the peri-implant tissues after robot-assisted full arch surgery at 1-year visit remained stable. There is little evidence indicating clinical outcomes especially for patient-reported outcomes. A more detailed clinical assessment should be included for further research.
Current issues with dental implant robotic systems
Need for further simplification of robotic surgical procedures.
Although robotic-assisted dental implant surgery can improve accuracy and treatment quality, 94 it involves complex registration, calibration, and verification procedures that prolong the duration of surgery. These tedious processes may introduce new errors, 61 and lower work efficiency, especially in single tooth implant placement 62 that could extend visit times and affect patient satisfaction. 62 Besides, surgeons are required to undergo additional training to familiarize themselves with the robotic system. 87
Need for improved flexibility of dental implant robotic system
During implantation, the drill tips at the end of the robotic arms cannot be tilted, and this can increase the difficulty of using robots in posterior sections with limited occlusal space. 61 , 62 In addition, currently available marker systems require patients to wear additional devices to hold the marker in place. If these markers are contaminated or obstructed by blood, the visual system may not be able to detect them, limiting surgical maneuverability to some extent. During immediate implant placement or in cases of poor bone quality in the implant site, the drill tips may deviate towards the tooth sockets or areas of lower bone density, seriously affecting surgical precision.
Currently, only one study has developed a corresponding force-deformation compensation strategy for robots, 68 but clinical validation is still lacking. Additionally, the dental implant robotic system, along with other dental implant robots developed for prosthetics, endodontics, and orthodontics, is currently single-functional. Multi-functional robots are required for performing various dental treatments.
Difficulties in promoting the use of dental implant robotic system
Despite the enormous potential of robotic systems in the medical field, similar to the development of computer-aided design/computer-aided manufacturing technology, introducing and applying this technology faces multiple challenges in the initial stages. The high cost of robotic equipment may limit its promotion and application in certain regions or medical institutions. Surgeons require specialized technical training before operating robotic systems, which translates to additional training costs and time investment. 95
Prospects in the use of dental implant robotic system
Medical robots possess high-precision sensing and positioning capabilities, which enable precise operations at small scales. They are also equipped with safety mechanisms and stability controls to ensure the safety of medical procedures and reduce risks to patients. As technology evolves, hardware and algorithms are continuously updated, resulting in constant performance improvements. Today, medical robots are widely used in surgery, diagnosis, and rehabilitation. 7 They enable precise and minimally invasive operation, thus reducing patient trauma and pain, shortening hospitalization, and speeding recovery, as well as reducing the need for re-operations and blood transfusions. 96 In addition, medical robots can reduce radiation exposure for both surgeons and patients. By leveraging machine learning and artificial intelligence technologies, robots can provide personalized and intelligent treatment plans and recommendations based on large amounts of data, improving diagnostic efficiency. Robots with remote operation capabilities can enable remote surgeries or consultations across regions, facilitating access to medical services. Moreover, robots can work continuously, ensuring medical quality and consistency while reducing surgeons ’neck and back pain, 97 as well as numbness in the hands and wrists experienced by surgeons. 98 Besides, they also reduce mental and physical stress, improving surgeons’ quality of life and extending their career longevity.
From da Vinci surgical robotic system to dental implant robotic system, these innovative technologies are leading unprecedented changes in the medical field. Dental implant robotic system continuously improves software modules and optimizes operating procedures to become more intelligent, more flexible and easier to learn and use. In the future, more extensive clinical trials will be needed to continuously observe and evaluate the long-term outcomes of robot-assisted implant surgery, especially in multi-center clinical trials. Moreover, measured outcomes must include well-defined clinical outcomes (such as pathophysiology 99 ), technical outcomes (including those derived from robotic kinematic and haptic sensors 100 ), patient-reported outcomes (such as quality-of-life indicators and overall satisfaction with treatment 99 ), and wider outcomes that reflect potential robotic disruption (ergonomic benefits, impacts on accessibility to surgery 100 ) where relevant. In addition, the evaluation of dental implant robots requires the analysis of learning curves. Large prospective cohorts provide the first opportunity to capture real-world learning curves, which can be used to develop training mechanisms that shorten learning curves and minimize any negative impact on patients. 99 , 100
As a pioneering attempt, the dental implant robotic system provides an important exploration and paradigm for the application of another dental robotic system. As technology continues to advance, robotics and artificial intelligence will provide more precise diagnostic and treatment options, more intelligent medical decision support systems, as well as more flexible and precise surgical procedures. These revolutionary technologies will continue to drive advances in medicine and healthcare, opening up new possibilities for future clinical practice.
With novel technology advancements, medical robotics are bringing a new era to medicine. Innovative medical robotics can perform surgical procedures, aid rehabilitation, make diagnoses, achieve robotic laboratory automation and other robots suitable for various functional needs. In the field of dentistry, the most widely utilized robotic system presently is the dental implant robotic system. Implant robotic systems could offer a more flexible approach for the precise planning, and visual and haptic guidance of surgical procedures. Various clinical trials have confirmed the high accuracy of implant robotic-assisted surgery achieved and toward long-term implant success. However, there is still much room for improvement in terms of further simplification, the flexibility of robotic surgical procedures, and systematic education. By leveraging machine learning and artificial intelligence technologies, more precise diagnostic and treatment options, intelligent medical decision support systems, and flexible and precise surgical procedures will be provided for future clinical practice.
Fukuda, T., Dario, P. & Yang, G. Z. Humanoid robotics—history, current state of the art, and challenges. Sci. Robot. 2 , eaar4043 (2017).
Article PubMed Google Scholar
Dong, J. What you should know about the history of robotics. Robot Ind. 1 , 108–114 (2015).
Google Scholar
Standardization, I.O.F. Robots and robotic devices—vocabulary. ISO 8373:2021.
Liu, H. H., Li, L. J., Shi, B., Xu, C. W. & Luo, E. Robotic surgical systems in maxillofacial surgery: a review. Int. J. Oral. Sci. 9 , 63–73 (2017).
Article PubMed PubMed Central Google Scholar
Kwoh, Y. S., Hou, J., Jonckheere, E. A. & Hayati, S. A robot with improved absolute positioning accuracy for CT guided stereotactic brain surgery. IEEE Trans. Biomed. Eng. 35 , 153–160 (1988).
Article CAS PubMed Google Scholar
Troccaz, J., Dagnino, G. & Yang, G. Z. Frontiers of medical robotics: from concept to systems to clinical translation. Annu. Rev. Biomed. Eng. 21 , 193–218 (2019).
Dupont, P. E. et al. A decade retrospective of medical robotics research from 2010 to 2020. Sci. Robot. 6 , eabi8017 (2021).
Yang, G. Z. et al. Medical robotics—regulatory, ethical, and legal considerations for increasing levels of autonomy. Sci. Robot. 2 , eaam8638 (2017).
Yip, M. et al. Artificial intelligence meets medical robotics. Science 381 , 141–146 (2023).
Wang, T. M. et al. Medical Surgical Robotics. China Science Publishing & Media Ltd. http://find.nlc.cn/search/showDocDetails?docId=-9060319075756851951&dataSource=ucs01 (2013).
Liu, Y. et al. Fully automatic AI segmentation of dental implant surgery related tissues based on cone beam computed tomography images. Int. J. Oral Sci. (2024) (Accept for publication).
Alemzadeh, K. & Raabe, D. Prototyping artificial jaws for the robotic dental testing simulator. Proc. Inst. Mech. Eng. Part H 222 , 1209–1220 (2008).
Article CAS Google Scholar
Kazanzides, P. et al. Surgical and interventional robotics: core concepts, technology, and design. IEEE Robot. Autom. Mag. 15 , 122–130 (2008).
Khan, K., Dobbs, T., Swan, M. C., Weinstein, G. S. & Goodacre, T. E. Trans-oral robotic cleft surgery (TORCS) for palate and posterior pharyngeal wall reconstruction: a feasibility study. J. Plast. Reconstr. Aesthet. Surg. 69 , 97–100 (2016).
Nadjmi, N. Transoral robotic cleft palate surgery. Cleft Palate Craniofac. J. 53 , 326–331 (2016).
Vicini, C. et al. Transoral robotic tongue base resection in obstructive sleep apnoea-hypopnoea syndrome: a preliminary report. ORL J. Otorhinolaryngol. Relat. Spec. 72 , 22–27 (2010).
Weinstein, G. S. et al. Transoral robotic surgery alone for oropharyngeal cancer: an analysis of local control. Arch. Otolaryngol. Head. Neck Surg. 138 , 628–634 (2012).
Kayhan, F. T., Kaya, H. & Yazici, Z. M. Transoral robotic surgery for tongue-base adenoid cystic carcinoma. J. Oral. Maxillofac. Surg. 69 , 2904–2908 (2011).
Olivetto, M., Bettoni, J., Testelin, S. & Lefranc, M. Zygomatic implant placement using a robot-assisted flapless protocol: proof of concept. Int. J. Oral. Maxillofac. Surg. 52 , 710–715 (2023).
Li, C. et al. Autonomous robotic surgery for zygomatic implant placement and immediately loaded implant-supported full-arch prosthesis: a preliminary research. Int. J. Implant. Dent. 9 , 12 (2023).
Article CAS PubMed PubMed Central Google Scholar
Saleh, M. A., Hanapiah, F. A. & Hashim, H. Robot applications for autism: a comprehensive review. Disabil. Rehabil. Assist. Technol. 16 , 580–602 (2021).
Chen, X. P. Advancement and challenges of medical robots from an interdisciplinary viewpoint. Chin. Bull. Life Sci. 34 , 965–973 (2022).
Winchester, P. et al. Changes in supraspinal activation patterns following robotic locomotor therapy in motor-incomplete spinal cord injury. Neurorehabil. Neural Repair 19 , 313–324 (2005).
Alashram, A. R., Annino, G. & Padua, E. Robot-assisted gait training in individuals with spinal cord injury: a systematic review for the clinical effectiveness of Lokomat. J. Clin. Neurosci. 91 , 260–269 (2021).
Topping, M. An overview of the development of Handy 1, a rehabilitation robot to assist the severely disabled. Artif. Life Robot. 4 , 188–192 (2000).
Article Google Scholar
Meng, F., Peng, X. Y. & Xu, Y. N. Analysis of and research on the development of lower limb wearable exoskeleton. J. Mech. Transm. 46 , 163–169 (2022).
Ezaki, S. et al. Analysis of gait motion changes by intervention using robot suit hybrid assistive limb (HAL) in myelopathy patients after decompression surgery for ossification of posterior longitudinal ligament. Front. Neurorobot. 15 , 650118 (2021).
Tegally, H., San, J. E., Giandhari, J. & de Oliveira, T. Unlocking the efficiency of genomics laboratories with robotic liquid-handling. BMC Genomics 21 , 729 (2020).
Rupp, N., Peschke, K., Koppl, M., Drissner, D. & Zuchner, T. Establishment of low-cost laboratory automation processes using AutoIt and 4-axis robots. SLAS Technol. 27 , 312–318 (2022).
Wu, Y. H., Fassert, C. & Rigaud, A. S. Designing robots for the elderly: appearance issue and beyond. Arch. Gerontol. Geriatr. 54 , 121–126 (2012).
Liu, Y. et al. Boosting framework via clinical monitoring data to predict the depth of anesthesia. Technol. Health Care 30 , 493–500 (2022).
Yang, G. Z. et al. Combating COVID-19-The role of robotics in managing public health and infectious diseases. Sci. Robot. 5 , eabb5589 (2020).
Gao, A. et al. Progress in robotics for combating infectious diseases. Sci. Robot. 6 , eabf1462 (2021).
Yu, H. et al. Management of systemic risk factors ahead of dental implant therapy: a beard well lathered is half shaved. J. Leukoc. Biol. 110 , 591–604 (2021).
Cheng, L. et al. [A review of peri-implant microbiology]. Hua XI Kou Qiang Yi Xue Za Zhi 37 , 7–12 (2019).
PubMed Google Scholar
Patel, R. & Clarkson, E. Implant surgery update for the general practitioner: dealing with common postimplant surgery complications. Dent. Clin. North Am. 65 , 125–134 (2021).
Herrera, D. et al. Prevention and treatment of peri-implant diseases — the EFP S3 level clinical practice guideline. J. Clin. Periodontol. 50 , 4–76 (2023).
Wu Q., Research on the creation and application of the spatial mapping devices of the dental implant robot system. Fourth Military Medical University. Vol. graduate. (2016).
Ruff, C., Richards, R., Ponniah, A., Witherow, H., Evans, R. & Dunaway, D. Computed maxillofacial image in surgical navigation. Int. J. Comput. Assist. Radiol. Surg. 2 , 412–418 (2007).
Tal, H. & Moses, O. A comparison of panoramic radiography with computed tomography in the planning of implant surgery. Dentomaxillofac. Radiol. 20 , 40–42 (1991).
Tattan, M., Chambrone, L., Gonzalez-Martin, O. & Avila-Ortiz, G. Static computer-aided, partially guided, and free-handed implant placement: a systematic review and meta-analysis of randomized controlled trials. Clin. Oral. Implant. Res. 31 , 889–916 (2020).
Shen, P. et al. Accuracy evaluation of computer-designed surgical guide template in oral implantology. J. Cranio Maxillofac. Surg. 43 , 2189–2194 (2015).
Vercruyssen, M., Fortin, T., Widmann, G., Jacobs, R. & Quirynen, M. Different techniques of static/dynamic guided implant surgery: modalities and indications. Periodontology 2000 66 , 214–227 (2014).
Zhao Y. Clinical study of an autonomous dental implant robot. In: 2021 Compendium of Papers from the Sixth National Oral and Maxillofacial Prosthodontics Annual Meeting of the Oral and Maxillofacial Prosthodontics Committee of the Chinese Dental Association . 7-8 https://doi.org/10.26914/c.cnkihy.2021.063176 (2021).
Kivovics, M., Takacs, A., Penzes, D., Nemeth, O. & Mijiritsky, E. Accuracy of dental implant placement using augmented reality-based navigation, static computer assisted implant surgery, and the free-hand method: an in vitro study. J. Dent. 119 , 104070 (2022).
Chen, W. et al. Accuracy of dental implant placement with a robotic system in partially edentulous patients: a prospective, single-arm clinical trial. Clin. Oral. Implant. Res. 34 , 707–718 (2023).
Gwangho, K., Hojin, S., Sungbeen, I., Dongwan, K. & Sanghwa, J. A study on simulator of human-robot cooperative manipulator for dental implant surgery. 2009 IEEE International Symposium on Industrial Electronics, Seoul, Korea (South) . 2159-2164 https://doi.org/10.1109/ISIE.2009.5222561 (2009).
Alqutaibi, A. Y., Hamadallah, H. H., Abu, Z. B., Aloufi, A. M. & Tarawah, R. A. Applications of robots in implant dentistry: a scoping review. J. Prosthet. Dent . 11:S0022-3913(23)00770-9. Epub ahead of print. https://doi.org/10.1016/j.prosdent.2023.11.019 (2023).
Dutreuil, J. G. F. L. Computer Assisted Dental Implantology: A New Method and a Clinical Validation. In: Niessen, W.J., Viergever, M.A. (eds) Medical Image Computing and Computer-Assisted Intervention – MICCAI 2001. MICCAI 2001. 2208 https://doi.org/10.1007/3-540-45468-3_46 (2001).
R. Boesecke, J. B. J. R. Robot Assistant for Dental Implantology . (Springer: Berlin, Heidelberg, 2001). .
Sun, X. et al. Automated dental implantation using image-guided robotics: registration results. Int. J. Comput. Assist. Radiol. Surg. 6 , 627–634 (2011).
Sun, X., Yoon, Y., Li, J. & Mckenzie, F. D. Automated image-guided surgery for common and complex dental implants. J. Med. Eng. Technol. 38 , 251–259 (2014).
Bolding, S. L. & Reebye, U. N. Accuracy of haptic robotic guidance of dental implant surgery for completely edentulous arches. J. Prosthet. Dent. 128 , 639–647 (2022).
Zhou, G. et al. Intraoperative localization of small pulmonary nodules to assist surgical resection: a novel approach using a surgical navigation puncture robot system. Thorac. Cancer 11 , 72–81 (2020).
K, Y. et al. Stereo vision based robot navigation system using modulated potential field for implant surgery. IEEE International Conference on Industrial Technology 493–498 https://doi.org/10.1109/ICIT.2015.7125147 (2015).
S, Y., A, M. P. & Y, V. D. R., Automation of end effector guidance of robotic arm for dental implantation using computer vision. IEEE Distributed Computing, VLSI, Electrical Circuits and Robotics 84–89. https://doi.org/10.1109/DISCOVER.2016.7806263 (2016).
Yan, B. et al. Optics-guided Robotic System for Dental Implant Surgery. Chin. J. Mech. Eng. 35 , 55 (2022).
Xie R. The study on accurary of the Dental Implantology Robotic System. Fourth Military Medical University . Vol. graduate. (2016).
Wilmes, B. & Drescher, D. Impact of insertion depth and predrilling diameter on primary stability of orthodontic mini-implants. Angle Orthod. 79 , 609–614 (2009).
Wilmes, B., Su, Y. Y. & Drescher, D. Insertion angle impact on primary stability of orthodontic mini-implants. Angle Orthod. 78 , 1065–1070 (2008).
Shi, J. Y. et al. Improved positional accuracy of dental implant placement using a haptic and machine-vision-controlled collaborative surgery robot: a pilot randomized controlled trial. J. Clin. Periodontol. 51 , 24–32 (2024).
Qiao, S. C., Wu, X. Y., Shi, J. Y., Tonetti, M. S. & Lai, H. C. Accuracy and safety of a haptic operated and machine vision controlled collaborative robot for dental implant placement: a translational study. Clin. Oral. Implant. Res. 34 , 839–849 (2023).
Yuan, F. S. et al. Preliminary study on the automatic preparation of dental implant socket controlled by micro-robot. Zhonghua Kou Qiang Yi Xue Za Zhi 53 , 524–528 (2018).
CAS PubMed Google Scholar
Kan, T. S. et al. Evaluation of a custom-designed human-robot collaboration control system for dental implant robot. Int. J. Med. Robot. Comput. Assist. Surg. 18 , e2346 (2022).
Cheng, K. J. et al. Accuracy of dental implant surgery with robotic position feedback and registration algorithm: an in-vitro study. Comput. Biol. Med. 129 , 104153 (2021).
Feng, Y. et al. An image-guided hybrid robot system for dental implant surgery. Int. J. Comput. Assist. Radiol. Surg. 17 , 15–26 (2022).
Tao, B. et al. The accuracy of a novel image-guided hybrid robotic system for dental implant placement: an in vitro study. Int. J. Med. Robot. Comput. Assist. Surg. 19 , e2452 (2023).
Li Z. W. The study on accuracy of the Dental Implantology Robotic System. Air Force Medical University Vol. Graduate. (2021).
Chen, D., Chen, J., Wu, X., Chen, Z. & Liu, Q. Prediction of primary stability via the force feedback of an autonomous dental implant robot. J. Prosthet. Dent . S0022-3913(23)00755-2. Epub ahead of print. https://doi.org/10.1016/j.prosdent.2023.11.008 (2023).
Zhao, R. et al. Correlation between intraosseous thermal change and drilling impulse data during osteotomy within autonomous dental implant robotic system: an in vitro study. Clin. Oral Implant. Res. 35 , 258–267 (2023).
Yang, S. et al. Accuracy of autonomous robotic surgery for single-tooth implant placement: a case series. J. Dent. 132 , 104451 (2023).
Rawal, S., Tillery, D. J. & Brewer, P. Robotic-assisted prosthetically driven planning and immediate placement of a dental implant. Compend. Contin. Educ. Dent. 41 , 26–30 (2020).
Li, Z., Xie, R., Bai, S. & Zhao, Y. Implant placement with an autonomous dental implant robot: a clinical report. J. Prosthet. Dent . S0022-3913(23)00124-5. Epub ahead of print. https://doi.org/10.1016/j.prosdent.2023.02.014 (2023).
Talib, H. S., Wilkins, G. N. & Turkyilmaz, I. Flapless dental implant placement using a recently developed haptic robotic system. Br. J. Oral. Maxillofac. Surg. 60 , 1273–1275 (2022).
Ali, M. Flapless dental implant surgery enabled by haptic robotic guidance: a case report. Clin. Implant Dent. Relat. Res . Epub ahead of print. https://doi.org/10.1111/cid.13279 (2023).
Chen, J. et al. Comparison the accuracy of a novel implant robot surgery and dynamic navigation system in dental implant surgery: an in vitro pilot study. BMC Oral. Health 23 , 179 (2023).
Xu, Z. et al. Accuracy and efficiency of robotic dental implant surgery with different human-robot interactions: an in vitro study. J. Dent. 137 , 104642 (2023).
Yang, S., Chen, J., Li, A., Li, P. & Xu, S. Autonomous robotic surgery for immediately loaded implant-supported maxillary full-arch prosthesis: a case report. J. Clin. Med. 11 , 6594 (2022).
Jia, S., Wang, G., Zhao, Y. & Wang, X. Accuracy of an autonomous dental implant robotic system versus static guide-assisted implant surgery: a retrospective clinical study. J. Prosthet. Dent . S0022-3913(23)00284-6. Epub ahead of print. https://doi.org/10.1016/j.prosdent.2023.04.027 (2023).
Bai, S. Z. et al. Animal experiment on the accuracy of the Autonomous Dental Implant Robotic System. Zhonghua Kou Qiang Yi Xue Za Zhi 56 , 170–174 (2021).
Zhao, Y. et al. Effect of the number and distribution of fiducial markers on the accuracy of robot-guided implant surgery in edentulous mandibular arches: an in vitro study. J. Dent. 134 , 104529 (2023).
Mozer, P. S. Accuracy and deviation analysis of static and robotic guided implant surgery: a case study. Int. J. Oral. Maxillofac. Implants 35 , e86–e90 (2020).
Chen, J. et al. Accuracy of immediate dental implant placement with task-autonomous robotic system and navigation system: an in vitro study. Clin. Oral Implant. Res . Epub ahead of print. https://doi.org/10.1111/clr.14104 (2023).
Cao, Z. et al. Pilot study of a surgical robot system for zygomatic implant placement. Med. Eng. Phys. 75 , 72–78 (2020).
Zhang, K., Yu, M. L., C, C. & Xu, B. H. Preliminary research on the accuracy of implant surgery assisted by implant surgery robot. China Med. Device Inf. 27 , 25–28 (2021).
CAS Google Scholar
Tao, B. et al. Accuracy of dental implant surgery using dynamic navigation and robotic systems: an in vitro study. J. Dent. 123 , 104170 (2022).
Ding, Y. et al. Accuracy of a novel semi-autonomous robotic-assisted surgery system for single implant placement: a case series. J. Dent. 139 , 104766 (2023).
Li, P. et al. Accuracy of autonomous robotic surgery for dental implant placement in fully edentulous patients: a retrospective case series study. Clin. Oral. Implant. Res. 34 , 1428–1437 (2023).
Wang, W. et al. Accuracy of the Yakebot dental implant robotic system versus fully guided static computer-assisted implant surgery template in edentulous jaw implantation: a preliminary clinical study. Clin. Implant Dent. Relat. Res . https://doi.org/10.1111/cid.13278 . Epub ahead of print (2023).
Xie, R. et al. Clinical evaluation of autonomous robotic-assisted full-arch implant surgery: a 1-year prospective clinical study. Clin. Oral Implant. Res . Epub ahead of print. https://doi.org/10.1111/clr.14243 (2024).
Takacs, A. et al. Advancing accuracy in guided implant placement: a comprehensive meta-analysis: meta-analysis evaluation of the accuracy of available implant placement methods. J. Dent. 139 , 104748 (2023).
He, J. et al. In vitro and in vivo accuracy of autonomous robotic vs. fully guided static computer-assisted implant surgery. Clin. Implant Dent. Relat. Res. https://doi.org/10.1111/cid.13302 (2024). Epub ahead of print.
Zhang, S. et al. Accuracy of implant placement via dynamic navigation and autonomous robotic computer-assisted implant surgery methods: a retrospective study. Clin. Oral. Implant. Res. 35 , 220–229 (2024).
Shi, B. & Huang, H. Computational technology for nasal cartilage-related clinical research and application. Int. J. Oral. Sci. 12 , 21 (2020).
Zhou, L., Teng, W., Li, X. & Su, Y. Accuracy of an optical robotic computer-aided implant system and the trueness of virtual techniques for measuring robot accuracy evaluated with a coordinate measuring machine in vitro. J. Prosthet. Dent . 11:S0022-3913(23)00751-5. Epub ahead of print. https://doi.org/10.1016/j.prosdent.2023.11.004 (2023).
Forsmark, A. et al. Health economic analysis of open and robot-assisted laparoscopic surgery for prostate cancer within the prospective multicentre LAPPRO trial. Eur. Urol. 74 , 816–824 (2018).
Rokhshad, R., Keyhan, S. O. & Yousefi, P. Artificial intelligence applications and ethical challenges in oral and maxillo-facial cosmetic surgery: a narrative review. Maxillofac. Plast. Reconstr. Surg. 45 , 14 (2023).
Gofrit, O. N. et al. Surgeons’ perceptions and injuries during and after urologic laparoscopic surgery. Urology 71 , 404–407 (2008).
Tonetti, M. S. et al. Relevant domains, core outcome sets and measurements for implant dentistry clinical trials: the Implant Dentistry Core Outcome Set and Measurement (ID-COSM) international consensus report. J. Clin. Periodontol. 50 , 5–21 (2023).
Marcus, H. J. et al. The IDEAL framework for surgical robotics: development, comparative evaluation and long-term monitoring. Nat. Med. 30 , 61–75 (2024).
Sun, Z. J. & Tian, Z. M. Advances in neurosurgical surgical robotics. Chin. J. Minimally Invasive Neurosurg 5 , 238–240 (2008).
Abdul-Muhsin, H. P. V. History of Robotic Surgery. In: (eds Kim, K.) Robotics in General Surgery . (Springer, New York, NY, 2014).
Ewing, D. R., Pigazzi, A., Wang, Y. & Ballantyne, G. H. Robots in the operating room–the history. Semin. Laparosc. Surg. 11 , 63–71 (2004).
Leal, G. T. & Campos, C. O. 30 Years of robotic surgery. World J. Surg. 40 , 2550–2557 (2016).
Falcone, T., Goldberg, J., Garcia-Ruiz, A., Margossian, H. & Stevens, L. Full robotic assistance for laparoscopic tubal anastomosis: a case report. J. Laparoendosc. Adv. Surg. Tech. 9 , 107–113 (1999).
Maeso, S. et al. Efficacy of the Da Vinci surgical system in abdominal surgery compared with that of laparoscopy: a systematic review and meta-analysis. Ann. Surg. 252 , 254–262 (2010).
M, J. et al. The hands-on orthopaedic robot “acrobot”: Early clinical trials of total knee replacement surgery. IEEE Trans. Robot. Autom. 19 , 902–911 (2003).
Schweikard, A., Shiomi, H. & Adler, J. Respiration tracking in radiosurgery. Med. Phys. 31 , 2738–2741 (2004).
Lieberman, I. H. et al. Bone-mounted miniature robotic guidance for pedicle screw and translaminar facet screw placement: Part I—technical development and a test case result. Neurosurgery 59 , 641–650 (2006).
Reddy, V. Y. et al. View-synchronized robotic image-guided therapy for atrial fibrillation ablation: experimental validation and clinical feasibility. Circulation 115 , 2705–2714 (2007).
Subramanian, P., Wainwright, T. W., Bahadori, S. & Middleton, R. G. A review of the evolution of robotic-assisted total hip arthroplasty. Hip Int. 29 , 232–238 (2019).
S, V., G, P. H., J, F. M., J, A. L. & P, C. ViKY robotic scope holder: initial clinical experience and preliminary results using instrument tracking. IEEE/ASME Trans. Mechatron. 15 , 879–886 (2010).
Zhao, R. F., Li, Z. W. & Bai, S. Z. Application of surgical robots in stomatology. Chin. J. Robot. Surg. 3 , 351–366 (2022).
Riga, C. V., Bicknell, C. D., Rolls, A., Cheshire, N. J. & Hamady, M. S. Robot-assisted fenestrated endovascular aneurysm repair (FEVAR) using the Magellan system. J. Vasc. Interv. Radiol. 24 , 191–196 (2013).
Herry, Y. et al. Improved joint-line restitution in unicompartmental knee arthroplasty using a robotic-assisted surgical technique. Int. Orthop. 41 , 2265–2271 (2017).
Wu, Q. & Zhao, Y. M. Application of robotics in stomatology. Int. J. Comput. Dent. 45 , 615–620 (2018).
Lang, S. et al. A european multicenter study evaluating the flex robotic system in transoral robotic surgery. Laryngoscope 127 , 391–395 (2017).
Download references
This work was supported by the National Natural Science Foundation of China [grant number 81970987].
Author information
These authors contributed equally: Chen Liu, Yuchen Liu
Authors and Affiliations
State Key Laboratory of Oral & Maxillofacial Reconstruction and Regeneration, Xi’an, China
Chen Liu, Yuchen Liu, Rui Xie, Zhiwen Li, Shizhu Bai & Yimin Zhao
National Clinical Research Center for Oral Diseases, Xi’an, China
Shaanxi Key Laboratory of Stomatology, Xi’an, China
Digital Center, School of Stomatology, The Fourth Military Medical University, Xi’an, China
You can also search for this author in PubMed Google Scholar
Contributions
Conceptualization, Bai S.Z., and Zhao Y.M.; writing—original draft preparation, Liu C and Liu Y.C.; writing—review and editing, Xie R and Li Z.W., Bai S.Z., and Zhao Y.M. All authors have read and agreed to the published version of the paper.
Corresponding authors
Correspondence to Shizhu Bai or Yimin Zhao .
Ethics declarations
Competing interests.
The authors declare no competing interests.
Rights and permissions
Open Access This article is licensed under a Creative Commons Attribution 4.0 International License, which permits use, sharing, adaptation, distribution and reproduction in any medium or format, as long as you give appropriate credit to the original author(s) and the source, provide a link to the Creative Commons licence, and indicate if changes were made. The images or other third party material in this article are included in the article’s Creative Commons licence, unless indicated otherwise in a credit line to the material. If material is not included in the article’s Creative Commons licence and your intended use is not permitted by statutory regulation or exceeds the permitted use, you will need to obtain permission directly from the copyright holder. To view a copy of this licence, visit http://creativecommons.org/licenses/by/4.0/ .
Reprints and permissions
About this article
Cite this article.
Liu, C., Liu, Y., Xie, R. et al. The evolution of robotics: research and application progress of dental implant robotic systems. Int J Oral Sci 16 , 28 (2024). https://doi.org/10.1038/s41368-024-00296-x
Download citation
Received : 15 January 2024
Revised : 11 March 2024
Accepted : 13 March 2024
Published : 08 April 2024
DOI : https://doi.org/10.1038/s41368-024-00296-x
Share this article
Anyone you share the following link with will be able to read this content:
Sorry, a shareable link is not currently available for this article.
Provided by the Springer Nature SharedIt content-sharing initiative
This article is cited by
High-precision all-in-one dual robotic arm strategy in oral implant surgery.
- Yuehua Ding
BDJ Open (2024)
Quick links
- Explore articles by subject
- Guide to authors
- Editorial policies

Help | Advanced Search
Computer Science > Robotics
Title: accelerating surgical robotics research: a review of 10 years with the da vinci research kit.
Abstract: Robotic-assisted surgery is now well-established in clinical practice and has become the gold standard clinical treatment option for several clinical indications. The field of robotic-assisted surgery is expected to grow substantially in the next decade with a range of new robotic devices emerging to address unmet clinical needs across different specialities. A vibrant surgical robotics research community is pivotal for conceptualizing such new systems as well as for developing and training the engineers and scientists to translate them into practice. The da Vinci Research Kit (dVRK), an academic and industry collaborative effort to re-purpose decommissioned da Vinci surgical systems (Intuitive Surgical Inc, CA, USA) as a research platform for surgical robotics research, has been a key initiative for addressing a barrier to entry for new research groups in surgical robotics. In this paper, we present an extensive review of the publications that have been facilitated by the dVRK over the past decade. We classify research efforts into different categories and outline some of the major challenges and needs for the robotics community to maintain this initiative and build upon it.
Subjects: | Robotics (cs.RO) |
Cite as: | [cs.RO] |
(or [cs.RO] for this version) | |
Focus to learn more arXiv-issued DOI via DataCite | |
: | Focus to learn more DOI(s) linking to related resources |
Submission history
Access paper:.
- Other Formats

References & Citations
- Google Scholar
- Semantic Scholar
DBLP - CS Bibliography
Bibtex formatted citation.

Bibliographic and Citation Tools
Code, data and media associated with this article, recommenders and search tools.
- Institution
arXivLabs: experimental projects with community collaborators
arXivLabs is a framework that allows collaborators to develop and share new arXiv features directly on our website.
Both individuals and organizations that work with arXivLabs have embraced and accepted our values of openness, community, excellence, and user data privacy. arXiv is committed to these values and only works with partners that adhere to them.
Have an idea for a project that will add value for arXiv's community? Learn more about arXivLabs .
- Introduction
- Conclusions
- Article Information
Data are from the Michigan Surgical Quality Collaborative from January 1, 2012, through June 30, 2018. These data reflect practices at all hospitals included in the study.
Proportional use of robotic, laparoscopic, and open approaches for general surgical procedures are shown in the 4 years before and after hospitals began performing robotic general surgery. From 2012 to 2018, 23 of 73 hospitals (31.5%) in the Michigan Surgical Quality Collaborative started performing robotic general surgery. These data are restricted to those hospitals.
eTable 1. Trends in the Use of Open Surgery for Specific Procedures, 2012-2018
eTable 2. Trends in the Use of Laparoscopic Surgery for Specific Procedures, 2012-2018
eFigure. Proportion of Hospitals and Surgeons Performing Any Robotic General Surgery in Michigan, 2012-2018
See More About
Sign up for emails based on your interests, select your interests.
Customize your JAMA Network experience by selecting one or more topics from the list below.
- Academic Medicine
- Acid Base, Electrolytes, Fluids
- Allergy and Clinical Immunology
- American Indian or Alaska Natives
- Anesthesiology
- Anticoagulation
- Art and Images in Psychiatry
- Artificial Intelligence
- Assisted Reproduction
- Bleeding and Transfusion
- Caring for the Critically Ill Patient
- Challenges in Clinical Electrocardiography
- Climate and Health
- Climate Change
- Clinical Challenge
- Clinical Decision Support
- Clinical Implications of Basic Neuroscience
- Clinical Pharmacy and Pharmacology
- Complementary and Alternative Medicine
- Consensus Statements
- Coronavirus (COVID-19)
- Critical Care Medicine
- Cultural Competency
- Dental Medicine
- Dermatology
- Diabetes and Endocrinology
- Diagnostic Test Interpretation
- Drug Development
- Electronic Health Records
- Emergency Medicine
- End of Life, Hospice, Palliative Care
- Environmental Health
- Equity, Diversity, and Inclusion
- Facial Plastic Surgery
- Gastroenterology and Hepatology
- Genetics and Genomics
- Genomics and Precision Health
- Global Health
- Guide to Statistics and Methods
- Hair Disorders
- Health Care Delivery Models
- Health Care Economics, Insurance, Payment
- Health Care Quality
- Health Care Reform
- Health Care Safety
- Health Care Workforce
- Health Disparities
- Health Inequities
- Health Policy
- Health Systems Science
- History of Medicine
- Hypertension
- Images in Neurology
- Implementation Science
- Infectious Diseases
- Innovations in Health Care Delivery
- JAMA Infographic
- Law and Medicine
- Leading Change
- Less is More
- LGBTQIA Medicine
- Lifestyle Behaviors
- Medical Coding
- Medical Devices and Equipment
- Medical Education
- Medical Education and Training
- Medical Journals and Publishing
- Mobile Health and Telemedicine
- Narrative Medicine
- Neuroscience and Psychiatry
- Notable Notes
- Nutrition, Obesity, Exercise
- Obstetrics and Gynecology
- Occupational Health
- Ophthalmology
- Orthopedics
- Otolaryngology
- Pain Medicine
- Palliative Care
- Pathology and Laboratory Medicine
- Patient Care
- Patient Information
- Performance Improvement
- Performance Measures
- Perioperative Care and Consultation
- Pharmacoeconomics
- Pharmacoepidemiology
- Pharmacogenetics
- Pharmacy and Clinical Pharmacology
- Physical Medicine and Rehabilitation
- Physical Therapy
- Physician Leadership
- Population Health
- Primary Care
- Professional Well-being
- Professionalism
- Psychiatry and Behavioral Health
- Public Health
- Pulmonary Medicine
- Regulatory Agencies
- Reproductive Health
- Research, Methods, Statistics
- Resuscitation
- Rheumatology
- Risk Management
- Scientific Discovery and the Future of Medicine
- Shared Decision Making and Communication
- Sleep Medicine
- Sports Medicine
- Stem Cell Transplantation
- Substance Use and Addiction Medicine
- Surgical Innovation
- Surgical Pearls
- Teachable Moment
- Technology and Finance
- The Art of JAMA
- The Arts and Medicine
- The Rational Clinical Examination
- Tobacco and e-Cigarettes
- Translational Medicine
- Trauma and Injury
- Treatment Adherence
- Ultrasonography
- Users' Guide to the Medical Literature
- Vaccination
- Venous Thromboembolism
- Veterans Health
- Women's Health
- Workflow and Process
- Wound Care, Infection, Healing
Get the latest research based on your areas of interest.
Others also liked.
- Download PDF
- X Facebook More LinkedIn
Sheetz KH , Claflin J , Dimick JB. Trends in the Adoption of Robotic Surgery for Common Surgical Procedures. JAMA Netw Open. 2020;3(1):e1918911. doi:10.1001/jamanetworkopen.2019.18911
Manage citations:
© 2024
- Permissions
Trends in the Adoption of Robotic Surgery for Common Surgical Procedures
- 1 Department of Surgery, University of Michigan, Ann Arbor
- 2 Center for Healthcare Outcomes and Policy, University of Michigan School of Medicine, Ann Arbor
- 3 currently a medical student at University of Michigan School of Medicine, Ann Arbor
Question Given concerns that robotic surgery is increasing for common surgical procedures with limited evidence and unclear clinical benefit, how is the use of robotic surgery changing over time?
Findings In this cohort study of 169 404 patients in 73 hospitals, the use of robotic surgery for all general surgery procedures increased from 1.8% to 15.1% from 2012 to 2018. Hospitals that launched robotic surgery programs had a broad and immediate increase in the use of robotic surgery, which was associated with a decrease in traditional laparoscopic minimally invasive surgery.
Meaning These findings highlight a need to continually monitor the adoption of robotic surgery to ensure that enthusiasm for new technology does not outpace the evidence needed to use it in the most effective clinical contexts.
Importance Increasing use of robotic surgery for common surgical procedures with limited evidence and unclear clinical benefit is raising concern. Analyses of population-based trends in practice and how hospitals’ acquisition of robotic surgical technologies is associated with their use are limited.
Objective To characterize trends in the use of robotic surgery for common surgical procedures.
Design, Setting, and Participants This cohort study used clinical registry data from Michigan from January 1, 2012, through June 30, 2018. Trends were characterized in the use of robotic surgery for common procedures for which traditional laparoscopic minimally invasive surgery was already considered a safe and effective approach for most surgeons when clinically feasible. A multigroup interrupted time series analysis was performed to determine how procedural approaches (open, laparoscopic, and robotic) change after hospitals launch a robotic surgery program. Data were analyzed from March 1 through April 19, 2019.
Exposures Initiation of robotic surgery.
Main Outcomes and Measures Procedure approach (ie, robotic, open, or laparoscopic).
Results The study cohort included 169 404 patients (mean [SD] age, 55.4 [16.9] years; 90 595 women [53.5%]) at 73 hospitals. The use of robotic surgery increased from 1.8% in 2012 to 15.1% in 2018 (8.4-fold increase; slope, 2.1% per year; 95% CI, 1.9%-2.3%). For certain procedures, the magnitude of the increase was greater; for example, for inguinal hernia repair, the use of robotic surgery increased from 0.7% to 28.8% (41.1-fold change; slope, 5.4% per year; 95% CI, 5.1%-5.7%). The use of robotic surgery increased 8.8% in the first 4 years after hospitals began performing robotic surgery (2.8% per year; 95% CI, 2.7%-2.9%). This trend was associated with a decrease in laparoscopic surgery from 53.2% to 51.3% (difference, −1.9%; 95% CI, −2.2% to −1.6%). Before adopting robotic surgery, hospitals’ use of laparoscopic surgery increased 1.3% per year. After adopting robotic surgery, the use of laparoscopic surgery declined 0.3% (difference in trends, −1.6%; 95% CI, −1.7% to −1.5%).
Conclusions and Relevance These results suggest that robotic surgery has continued to diffuse across a broad range of common surgical procedures. Hospitals that launched robotic surgery programs had a broad and immediate increase in the use of robotic surgery, which was associated with a decrease in traditional laparoscopic minimally invasive surgery.
Robotic surgery continues to diffuse across an increasingly broad range of surgical procedures. However, concerns have been raised that robotic surgery is more costly 1 , 2 and may be no more effective 3 , 4 than other established operative approaches, such as traditional laparoscopic minimally invasive and open surgery. With respect to costs, for example, robotic surgery has been associated with episode costs as much as 25% higher compared with laparoscopic surgery. There are also concerns about the rapid growth of robotic surgery in areas with limited evidence to support its use and little theoretical benefit or clinical rationale (eg, inguinal hernia repair). The US Food and Drug Administration (FDA) recently issued a warning against the use of robotic surgery for the treatment of breast and cervical cancers. 5 In their communication, they expressed concerns about the lack of epidemiologic data characterizing the use of robotic surgery in real-world practice settings. Current estimates are limited to single-center studies, 6 - 8 device manufacturers’ financial statements, 9 and claims data, which may be inaccurate owing to unreliable coding. 10 , 11 We used population-based data from a manually abstracted statewide clinical registry to characterize contemporary trends in the adoption of robotic surgery across a range of general surgical procedures, which now represent the largest market for the technology in the United States.
This cohort study used data from the Michigan Surgical Quality Collaborative (MSQC), an Agency for Healthcare Research and Quality–recognized patient safety organization. The MSQC represents a voluntary partnership between 73 Michigan hospitals and Blue Cross/Blue Shield of Michigan that focuses on clinical quality improvement for surgical care. Hospitals participating in the MSQC perform more than 90% of all surgical procedures in Michigan. The MSQC maintains a clinical registry using a standardized data collection platform, validated case-sampling methods, and trained nurse data abstractors at each participating site. Data accuracy is maintained through rigorous training, internal data audits, and annual site visits by MSQC program staff. This data source allowed us to identify robotic procedures with greater precision and accuracy than is possible using claims data. This study was approved by the University of Michigan institutional review board, which deemed the study exempt from informed consent owing to use of secondary data. This study was designed and reported in adherence to the Strengthening the Reporting of Observational Studies in Epidemiology ( STROBE ) reporting guideline.
We used data from the complete MSQC clinical registry file to identify all inpatient and outpatient general surgical episodes from January 1, 2012, through June 30, 2018. Procedures were identified and categorized by Current Procedural Terminology codes. We focused on general surgical procedures, which represent the clinical domain with the largest growth in robotic surgery. These files include additional information on patient age, demographic characteristics, and comorbid conditions in addition to detailed procedural information (eg, operative approach and anesthesia type), postoperative complications, death, and resource use (readmissions and emergency department visits).
Our primary outcome of interest was the surgical approach—robotic, laparoscopic, or open. The MSQC data were manually abstracted, and data on surgical approach were derived directly from the operative reports rather than procedural codes. Procedures were considered robotic if surgeons reported using the surgical robot in their operative report. Cases in which a robotic procedure was unexpectedly converted to another approach (eg, conversion to open procedure for bleeding) were characterized as robotic because this was the original approach chosen by the surgeon.
Data were analyzed from March 1 through April 19, 2019. The purpose of this analysis was to characterize trends in the use of surgical approaches over time for common general surgical procedures. We first reported raw proportions that were not adjusted for patient or hospital characteristics. We evaluated trends by calculating the fold change in each approach over time by dividing the proportional use of robotic surgery in 2018 by the proportional use in 2012. We also calculated the annual increase or decrease in the proportional use of each approach using linear regression. The coefficient for study years, modeled as a continuous variable, is reported as the annual trend. We then replicated the overall analysis stratified by specific procedures to determine whether overall trends were influenced by changes in practice for certain procedures.
To determine how hospitals change their practices after they begin performing robotic surgery, we performed a multigroup interrupted time series analysis. During the study period, 23 of the 73 MSQC participating hospitals (31.5%) began performing robotic surgery (32 hospitals were already performing robotic surgery at the time that MSQC began collecting data on this approach in 2012). We determined the date of the first robotic general surgery procedure within each of the hospitals that adopted robotic surgery during the study period. We then centered all hospitals on this date and evaluated the trends in the proportional use of each approach in the years before and after the hospital performed its first robotic operation. We used linear splines to model absolute levels and trends in the periods before and after introduction of robotic surgery. This analysis was designed to test the incremental association of adopting robotic surgery with trends in surgical practice but not to make assumptions about what would have happened had the hospital not begun performing robotic surgery. Our primary analysis was not adjusted for specific procedures, but we generated estimates for each procedure group in a sensitivity analysis. We estimated cluster-robust standard errors to account for repeated observations within hospitals. We performed all statistical analyses using Stata, version 14.2 statistical software (StataCorp LLC).
Characteristics for the 169 404 patients and 73 hospitals are included in Table 1 . The mean (SD) age for all patients was 55.4 (16.9) years; 90 595 (53.5%) were women and 78 809 (46.5%) were men. Cholecystectomy was the most common operation (62 854 [37.1%]). Of the 73 hospitals included in the study, 31 (42.5%) had fewer than 200 beds and 11 (15.1%) had at least 500 beds. Sixty-two hospitals (84.9%) were teaching hospitals, and the mean (SD) total surgical volume was 12 068 (10 933) cases.
From January 2012 through June 2018, the use of robotic surgery for all general surgery procedures increased from 1.8% to 15.1% (8.4-fold change; slope, 2.1% per year; 95% CI, 1.9%-2.3%) ( Figure 1 and Table 2 ). During the same period, the use of both laparoscopic and open surgery declined. For example, the proportional use of open surgery was 42.4% in 2012 compared with 32.4% in 2018 (0.8-fold change; slope, −1.5% per year; 95% CI, −1.8% to −1.2%) (eTable 1 and eTable 2 in the Supplement ). Trends in robotic surgery use were similar for specific procedures, although for some, the magnitude of the increase was greater. For example, the use of robotic surgery for inguinal hernia repair increased from 0.7% to 28.8% from January 2012 through June 2018 (41.1-fold change; slope, 5.4% per year; 95% CI, 5.1%-5.7%).
The proportion of hospitals and surgeons performing robotic surgery increased from January 2012 through June 2018. For example, 8.7% of surgeons performed robotic general surgery in 2012 compared with 35.1% in 2018 (eFigure in the Supplement ). During the study period, 23 hospitals (31.5%) began performing robotic surgery. In those hospitals, the use of robotic surgery increased from 3.1% in the first year to 13.1% in the fourth year after hospitals began performing robotic general surgery operations (overall mean in first 4 years, 8.8%; slope, 2.8% per year; difference, 2.8% [95% CI, 2.7%-2.9%]) ( Figure 2 and Table 3 ). The use of laparoscopic surgery decreased from 53.2% to 51.3% after hospitals began performing robotic surgery (difference, −1.9%; 95% CI, −2.2% to −1.6%) ( Table 3 ). Before hospitals performed robotic surgery, a trend toward greater use of laparoscopic surgery occurred (slope, 1.3% per year). A trend toward less laparoscopic surgery after hospitals began performing robotic surgery occurred (slope, −0.3% per year; difference, −1.6%; 95% CI, −1.7% to −1.5%). Results remained the same when stratified across specific procedures.
This study used a unique, clinically oriented, and manually abstracted data source to characterize the use of robotic surgery across a wide range of common general surgical procedures. These data identify the correct procedure approach with greater precision and accuracy than claims. We found that the use of robotic surgery increased dramatically from 2012 to 2018. Although the use of robotic surgery increased across all procedures, for certain operations, such as inguinal hernia repair, practice patterns shifted by an order of magnitude toward greater use of robotics. We also found that the use of robotic surgery increased rapidly and diffused widely across numerous different procedures in the years after hospitals begin performing robotic surgery. This trend was associated with a decrease in the use of open and laparoscopic minimally invasive procedures, which for most surgeons was already considered a safe and effective approach when clinically feasible.
Recent work suggests that the United States now performs more robotic surgery than any other country in the world, although overall trends in other specialties (eg, urology) toward greater use of robotic surgery have been present for many years. 9 Based on robotic device manufacturers’ financial statements, procedure volumes exceeded 600 000 in 2017, with the largest and fastest growing contributor being the field of general surgery. 9 This finding suggests that the clinical footprint for robotic surgery will continue to increase as it has for more than a decade already. However, accurate data on how robotic surgery is being applied in contemporary practice is lacking. Prior studies are limited to single-center reports and claims-based analyses that may be inaccurate owing to unreliable coding. 6 - 8 , 10 , 11 This inaccuracy is problematic because it may limit our ability to understand the clinical implications of this rapid change in practice. It also limits the ability of policy makers and regulators to scope oversight or, more broadly, the public health implications of rapid changes in surgical practice.
Within this context, regulators from the FDA recently expressed safety concerns about the rapidly growing use of robotic approaches for certain cancer operations. 5 These concerns stem from the limited evidence of benefit (eg, fewer complications or better oncologic resection quality) for robotic surgery. For example, randomized clinical trials have failed to demonstrate the benefits of robotic surgery over other approaches in the treatment of rectal cancer 12 and have shown even potentially worse outcomes in procedures for cervical cancer. 4 Observational studies that compared robotic surgery with more established laparoscopic or open approaches have also failed to demonstrate superior outcomes after inguinal hernia repair, 8 kidney resections, 1 colectomy, 13 - 16 or cholecystectomy. 7 The discrepancy between the ongoing rapid adoption of robotic surgery and unclear clinical benefit highlights why accurate information on how it is being applied in contemporary surgical practice is necessary.
This study expands on prior work in several ways. We used manually abstracted data from a statewide surgical registry to ensure that our estimates reflect the true incidence of robotic surgery across a wide range of procedures, hospitals, and surgeons. Making further use of these unique data, we estimated how the initiation of robotic surgery within hospitals had broad associations with surgical practice for numerous procedures that differed in complexity, anatomical location, and surgical indications (eg, repair of a hernia vs removal of an organ). This investigation builds on existing literature, which has shown similar associations of an increase in robotic prostatectomy with hospital acquisitions of robotic systems. 17 We also demonstrate that increasing use of robotic surgery changed existing trends toward greater use of laparoscopic surgery. For many common and low-risk procedures, such as cholecystectomy, conventional laparoscopic surgery is already the accepted standard of care. Laparoscopic approaches are also less expensive and can be performed by most general surgeons without robotics. 18 This situation highlights a questionable trend: robotic surgery is replacing conventional laparoscopic approaches for procedures that may not be complex enough to warrant the consideration of an advanced, expensive, and unproven minimally invasive platform.
This study suggests that regulators and the surgical community can take additional steps to monitor the ongoing diffusion of robotic surgery and ensure that this trend does not lead to diminished patient safety. Because accurate data are necessary to inform the creation of appropriate safeguards, the FDA and the Centers for Medicare & Medicaid Services should consider providing coverage for robotic surgery with provisions for evidence development. 19 This process has been previously used by the Centers for Medicare & Medicaid Services to create registries of patients treated with new and unproven surgical technologies (eg, carotid artery stenting). Use of these provisions would facilitate greater understanding of how robotic procedures are being used in real-world practice. Akin to postmarket surveillance of pharmaceuticals, such provisions would also create a common data resource from which the comparative safety and effectiveness of robotic operations can be evaluated by numerous investigators.
This action would also allow hospitals, which provide credentials to perform robotic surgery, to better understand where sufficient evidence suggests plausible benefit. At present, surgeons are largely able to use robotic surgery for any procedure at their professional discretion. As has been shown in the FDA warning and through prior studies, this discretionary use may place patients at risk for poor outcomes. 3 Facilitating transparency around the allocation of robotic surgery would allow patients to make better collaborative decisions with their surgeons. After all, for many of the procedures we report in this study, little to no evidence suggests that robotic surgery increases patient safety or treatment effectiveness compared with other approaches.
Our results should be interpreted within the context of several limitations. Our clinical registry only captures data from Michigan and therefore may not be generalizable to the country as a whole. However, the MSQC represents a heterogeneous group of hospitals, surgeons, and practice settings. Furthermore, we report on the most common general surgery procedures performed in the United States. Changes in patient factors, such as obesity, may influence trends in procedure choice. Our estimates may therefore be limited by a lack of adjustment for patient characteristics. That said, adjusting for patient factors may introduce its own biases because no clinical consensus exists around how robotic procedures should be allocated. Much of this decision-making is based on case-by-case surgeon assessments and clinical nuance not captured in any registry. Our results are consistent across multiple different procedures, which also suggests that these trends are independent of unique clinical domains or disease processes. Our study is unable to account for how other nonclinical factors, such as marketing, may influence the adoption of robotic surgery. However, others have found that the chances of receiving robotic surgery were 2- to 5-fold greater in highly competitive vs noncompetitive health care markets. 20 Moreover, evidence suggests that hospitals immediately begin advertising their acquisition of robotic surgical services through web-based and conventional health system marketing campaigns. 21 These data are complementary to ours and suggest that the greatest forces driving robotic surgery adoption may be the technological imperative and economic pressures experienced by hospitals in certain health care markets.
This study found that robotic surgery is rapidly diffusing across a broad range of common general surgical procedures. Trends toward greater use of the robotic platform appeared to occur rapidly after hospitals begin performing robotic surgery and were associated with a decrease in the use of established minimally invasive techniques, such as laparoscopic surgery. This trend was consistent across numerous specific procedures, even those for which conventional laparoscopic surgery is already considered standard of care and for which robotic surgery offers little theoretical clinical benefit to the patient. These findings highlight a need to continually monitor the diffusion of robotic surgery to ensure that enthusiasm for a new technology does not outpace the evidence needed to use it in the most effective clinical contexts.
Accepted for Publication: November 14, 2019.
Published: January 10, 2020. doi:10.1001/jamanetworkopen.2019.18911
Open Access: This is an open access article distributed under the terms of the CC-BY License . © 2020 Sheetz KH et al. JAMA Network Open .
Corresponding Author: Kyle H. Sheetz, MD, MSc, Center for Healthcare Outcomes & Policy, University of Michigan School of Medicine, 2800 Plymouth Rd, NCRC Bldg 16, Room 100N-11, Ann Arbor, MI 48109 ( [email protected] ).
Author Contributions: Drs Sheetz and Dimick had full access to all the data in the study and take responsibility for the integrity of the data and the accuracy of the data analysis.
Concept and design: All authors.
Acquisition, analysis, or interpretation of data: All authors.
Drafting of the manuscript: All authors.
Critical revision of the manuscript for important intellectual content: Sheetz, Dimick.
Statistical analysis: All authors.
Obtained funding: Dimick.
Administrative, technical, or material support: Dimick.
Supervision: Dimick.
Conflict of Interest Disclosures: Dr Dimick reported receiving personal fees from ArborMetrix, Inc, outside the submitted work and being an equity owner of ArborMetrix, Inc. No other disclosures were reported.
Funding/Support: This study was supported grants 2T32HS000053-27 (Dr Sheetz) and R01HS023597 (Dr Dimick) from the Agency for Healthcare Research and Quality and grant R01AG039434 from the National Institute on Aging, National Institutes of Health.
Role of the Funder/Sponsor: The sponsors had no role in the design and conduct of the study; collection, management, analysis, and interpretation of the data; preparation, review, or approval of the manuscript; or the decision to submit the manuscript for publication.
- Register for email alerts with links to free full-text articles
- Access PDFs of free articles
- Manage your interests
- Save searches and receive search alerts
- Open access
- Published: 20 January 2022
Robotic surgery in emergency setting: 2021 WSES position paper
- Nicola de’Angelis ORCID: orcid.org/0000-0002-1211-4916 1 , 2 ,
- Jim Khan 3 ,
- Francesco Marchegiani 4 ,
- Giorgio Bianchi 1 ,
- Filippo Aisoni 1 ,
- Daniele Alberti 5 ,
- Luca Ansaloni 6 ,
- Walter Biffl 7 ,
- Osvaldo Chiara 8 ,
- Graziano Ceccarelli 9 ,
- Federico Coccolini 10 ,
- Enrico Cicuttin 10 ,
- Mathieu D’Hondt 11 ,
- Salomone Di Saverio 12 ,
- Michele Diana 13 , 14 ,
- Belinda De Simone 15 ,
- Eloy Espin-Basany 16 ,
- Stefan Fichtner-Feigl 17 ,
- Jeffry Kashuk 18 ,
- Ewout Kouwenhoven 19 ,
- Ari Leppaniemi 20 ,
- Nassiba Beghdadi 1 , 2 ,
- Riccardo Memeo 21 ,
- Marco Milone 22 ,
- Ernest Moore 23 ,
- Andrew Peitzmann 24 ,
- Patrick Pessaux 25 , 26 , 27 ,
- Manos Pikoulis 28 ,
- Michele Pisano 29 ,
- Frederic Ris 30 ,
- Massimo Sartelli 31 ,
- Giuseppe Spinoglio 32 ,
- Michael Sugrue 33 ,
- Edward Tan 34 ,
- Paschalis Gavriilidis 35 ,
- Dieter Weber 36 ,
- Yoram Kluger 37 &
- Fausto Catena 38
World Journal of Emergency Surgery volume 17 , Article number: 4 ( 2022 ) Cite this article
11k Accesses
40 Citations
9 Altmetric
Metrics details
Robotics represents the most technologically advanced approach in minimally invasive surgery (MIS). Its application in general surgery has increased progressively, with some early experience reported in emergency settings. The present position paper, supported by the World Society of Emergency Surgery (WSES), aims to provide a systematic review of the literature to develop consensus statements about the potential use of robotics in emergency general surgery.
This position paper was conducted according to the WSES methodology. A steering committee was constituted to draft the position paper according to the literature review. An international expert panel then critically revised the manuscript. Each statement was voted through a web survey to reach a consensus.
Ten studies (3 case reports, 3 case series, and 4 retrospective comparative cohort studies) have been published regarding the applications of robotics for emergency general surgery procedures. Due to the paucity and overall low quality of evidence, 6 statements are proposed as expert opinions. In general, the experts claim for a strict patient selection while approaching emergent general surgery procedures with robotics, eventually considering it for hemodynamically stable patients only. An emergency setting should not be seen as an absolute contraindication for robotic surgery if an adequate training of the operating surgical team is available. In such conditions, robotic surgery can be considered safe, feasible, and associated with surgical outcomes related to an MIS approach. However, there are some concerns regarding the adoption of robotic surgery for emergency surgeries associated with the following: (i) the availability and accessibility of the robotic platform for emergency units and during night shifts, (ii) expected longer operative times, and (iii) increased costs. Further research is necessary to investigate the role of robotic surgery in emergency settings and to explore the possibility of performing telementoring and telesurgery, which are particularly valuable in emergency situations.
Conclusions
Many hospitals are currently equipped with a robotic surgical platform which needs to be implemented efficiently. The role of robotic surgery for emergency procedures remains under investigation. However, its use is expanding with a careful assessment of costs and timeliness of operations. The proposed statements should be seen as a preliminary guide for the surgical community stressing the need for reevaluation and update processes as evidence expands in the relevant literature.
Robotics represents the most technologically advanced approach in minimally invasive surgery (MIS). Its application has progressively gained acceptance in several surgical fields, being routinely used for elective urology, gynecology, digestive, and hepato-bilio-pancreatic surgery [ 1 , 2 , 3 , 4 , 5 , 6 , 7 , 8 ]. Conversely, robotic surgery in the emergency setting has not been explored, although some early experience has been reported in the literature [ 9 , 10 , 11 , 12 ]. Consequently, the issue regarding the role and potential applications of robotics for emergency procedures remains open. However, it deserves to be continuously monitored and updated in the future as evidence would emerge.
Project rationale and design
The present position paper is supported by the World Society of Emergency Surgery (WSES) and aims to provide a systematic review of the literature investigating the use of robotics in emergency general surgery to develop consensus statements based on the currently available evidence and practice. The present document should be seen as a preliminary guide for the surgical community stressing the need for reevaluation and update processes as evidence expands in the relevant literature.
For the purpose of this WSES position paper, the organizing committee (composed of Fausto Catena, Nicola de’Angelis, and Jim Khan) constituted a steering committee (made up of 16 experts), who had the task of drafting the present position paper, and an international expert panel composed of 21 experts who were asked to critically revise the manuscript and position statements. The position paper was conducted according to the WSES methodology [ 13 ]. We shall present the systematic review of the literature and provide the derived statements upon which a consensus was reached, specifying the quality of the supporting evidence and suggesting future research directions.
Systematic review
Review question, selection criteria, and search strategy.
The systematic review of the literature was performed following the Cochrane Collaboration specific protocol [ 14 ] and was reported according to the Preferred Reporting Items for Systematic Reviews and Meta-Analyses (PRISMA) statement [ 15 ].
The focus question was the following: what are the applications and outcomes of robotics for general surgery in emergency settings?
Studies reporting the use of a robotic surgical platform to manage general surgery emergencies and urgencies were searched in the following databases on June 30, 2021: MEDLINE (through PubMed), Embase, and the Cochrane Library. A specific research query was formulated for each database, using the following keywords and MeSH terms: emergency, emergency surgery, emergency setting, urgent, robotic surgery, robotic, robotics, robot-assisted, minimally invasive surgery, and minimally invasive surgery procedures.
According to the PICOS format, the following items were used as selection criteria for articles emerging from the literature search:
P, population: adult patients requiring surgery in emergent/urgent settings.
I, intervention: robotic or robot-assisted general surgery intervention.
C, comparisons: laparoscopy or open surgery or no comparison.
O, outcome(s): operative and postoperative surgical outcomes.
S, study design: due to the expected paucity of studies on the topic, all types of comparative study, but also case series and case reports were considered aiming to provide the most exhaustive picture of the current evidence and practice in robotic emergency general surgery.
The research was limited to studies published in English.
The literature search and selection were performed by two independent reviewers (GB and FM), who also screened the reference list of the selected articles to potentially include additional studies. First, all records from merged searches were reviewed for relevance concerning title and abstract. Records were removed when both reviewers excluded them. Otherwise, the disagreement was resolved via discussion or with the intervention of a tiebreaker (NdeA). Both reviewers then performed an independent full-text analysis, which allowed to finally include or exclude the preselected article.
Data extraction and synthesis
Data extraction was performed by filling in an electronic spreadsheet, which included the following items: first author’s name, year of publication, scientific journal, type of study, number of patients, pathological state requiring surgical intervention, type of surgical intervention, surgical approach, operative surgical outcomes, and postoperative surgical outcomes. The risk of bias in the selected studies was assessed by using validated systems according to the type of study design [ 16 , 17 , 18 ].
Literature search and selection
The initial search yielded 3767 results; after removing duplicates, 3662 articles were screened for eligibility based on title and abstract, and 31 articles were retrieved for a full-text evaluation. A total of 10 studies fulfilled the selection criteria and were finally included in the review (Fig. 1 ).
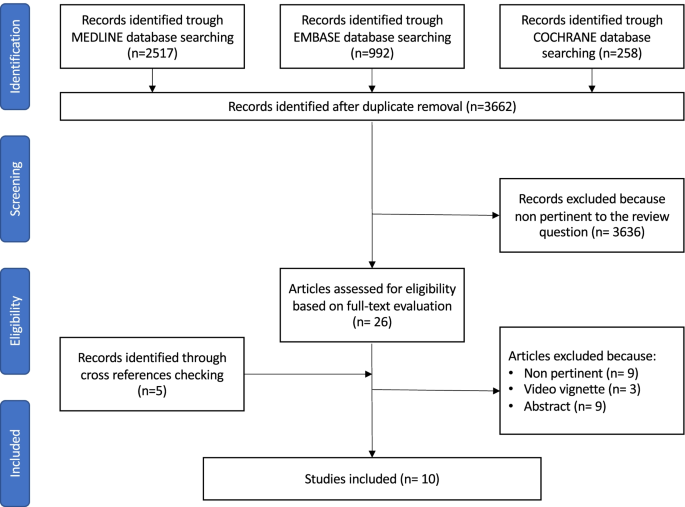
Flowchart of the literature search and selection
Study characteristics
The selected 10 studies were published between 2012 and 2021. They consisted of 5 cohort studies and 5 case reports conducted in Europe ( n = 3) and North America ( n = 7). The characteristics of the examined studies are summarized in Table 1 . Overall, they considered 279 patients.
Three studies reported interventions of colorectal surgery [ 9 , 10 , 19 ], two studies reported on hiatal hernia surgery [ 20 , 21 ], two studies reported on gallbladder surgery [ 22 , 23 ], two studies reported on bariatric surgery [ 12 , 24 ], and one study reported on abdominal wall surgery [ 25 ]. Only one case was a cancer-related emergency [ 10 ].
Qualitative synthesis of the literature
Robotics in emergency colorectal surgery
An early preliminary report of an emergent robotic repair of a colonic iatrogenic perforation was published by Pedraza et al. in 2012 [ 19 ]. The authors showed that such a procedure was feasible and successful. In 2014, Felli et al. [ 10 ] described the case of an 86-year old woman who underwent a robotic right colectomy for a bleeding ascending colon neoplasia. The surgery was uneventful and the reported postoperative outcomes were excellent. More recently, Anderson et al. [ 9 ] published a matched case–control study focusing on the use of robotics for urgent subtotal colectomies in patients presenting with ulcerative colitis. The results showed similar short-term outcomes for robotic and laparoscopic approaches.
Robotics in emergency hiatal hernia surgery
Over the last years, two groups published their early experience with robotic surgery for emergency hiatal hernia repair. In a case series of 3 patients undergoing robotic surgery for complicated giant hiatal hernia, Ceccarelli et al. [ 21 ] showed that postoperative outcomes were good. The authors suggested that the potential advantages of robotics over a conventional laparoscopic approach were mainly related to the surgeon’s comfort and precision during the intervention. Hosein et al. [ 20 ] performed a cohort-based analysis using data from the 2015–2017 Vizient clinical database, which included inpatient data from over 300 hospitals in the USA. Trend analysis demonstrated that laparoscopy was the most common approach in emergency hiatal hernia repair, representing 64.09% of cases, followed by the open (30.38%) and the robotic approach (5.53%). Concerning operative and postoperative outcomes, a trend was also observed for better outcomes in case of MIS (laparoscopy or robotic) hiatal hernia repair as compared to open surgery.
Robotics in emergency gallbladder surgery
In 2016, Kubat et al. [ 22 ] published a retrospective case series of 76 elective and 74 urgent robotic single-site cholecystectomies. The authors reported good perioperative outcomes, concluding that this approach was safe and efficient. In 2019, Milone et al. [ 23 ] described a case series of 3 patients who underwent robotic cholecystectomy for acute cholecystitis. The reported perioperative outcomes were excellent and the authors recommended the introduction of robotics in emergency settings in order to validate their preliminary results.
Robotics in emergency bariatric surgery
The first report of robotic emergency surgery after complicated robotic biliopancreatic diversion with duodenal switch was published by Sudan et al. in 2012 [ 24 ]. The robotic approach was preferred over open surgery in the management of postoperative complications in order to preserve the benefits of the previous MIS approach. The authors highlighted how the adoption of the robotic platform was useful in a patient in order to identify the damage and to repair it. More recently, Robinson et al. [ 12 ] published a retrospective cohort study comparing emergent laparoscopic and robotic gastrojejunal ulcer repair. The authors showed that in-room-to-surgery-start time was significantly reduced in the robotic group. Additionally, perioperative outcomes were in favor of the robotic approach, although not significantly different. However, robotic surgery was significantly more expensive than laparoscopy.
Robotics in emergency abdominal wall surgery
In 2020, Kudsi et al. [ 25 ] published an article on the perioperative and mid-term outcomes of 34 patients who underwent emergency robotic ventral hernia repair with different techniques between 2013 and 2019. With a 20.5% rate of minor postoperative complications (Clavien-Dindo grades I-II), a 11.7% rate of major postoperative complications (Clavien-Dindo grades III-IV), and only one (2.9%) patient experiencing hernia recurrence, the authors concluded that robotic ventral hernia repair was associated with promising results and overall feasibility in emergency settings, to be tested in further long-term follow-up studies.
Evaluation of the quality of evidence
Five out of 10 selected studies were retrospective cohort studies and were evaluated according to the NOS [ 18 ]. Two studies received a score of 8/9 [ 9 , 12 ], one study was graded 7/9 [ 20 ], and two studies had a score of 6/9 [ 22 , 25 ] (Table 2 ). The remaining studies were evaluated according to the tool described by Murad et al. [ 16 ]. All studies received a score of 6/8 [ 10 , 19 , 21 , 23 , 24 ] (Table 3 ).
Position statements
Following a comprehensive literature review and the summary of current scientific evidence on the applications of robotics for emergency general surgery procedures, the following position statements (PS) were put forward. For each statement, the supporting literature, the level of evidence, and the strength of the consensus are indicated. The level of evidence is classified according to the GRADE system ( https://training.cochrane.org/introduction-grade ). For each statement, the consensus was assessed through a web survey (by means of a Google Form) open to all members of the steering committee and panel of experts and to the members of the Board of Governors of the WSES. If a statement reached < 70% of agreement, it was rediscussed via email or videoconference, modified, and resubmitted to the experts’ vote until a consensus was reached.
The experts involved were also asked to describe their current practice. The great majority (82.6%) worked in a hospital equipped with a robotic surgical platform. However, the access to the robotic surgical system for emergency procedures appeared to be limited, with difficult availability (39.1%) only during the day (13%), or not available at all (43.5%).
PS-1. Robotic surgery in emergency settings is highly dependent on the surgeon’s experience and should only be performed in an appropriately equipped operating room with trained nursing staff.
Supporting literature
Robotic surgery requires a high level of technical expertise when compared to open or even laparoscopic surgery. A complete specialized training is required to be proficient in performing standardized surgical interventions associated with acceptable operative and postoperative outcomes [ 26 ]. In a recent article, Thomas et al. [ 27 ] analyzed the robotic colorectal surgery activity of a tertiary colorectal unit and concluded that success relies on a structured training curriculum, a dedicated surgical team, the institution’s support, and many other variables in addition to the training at the robotic console itself. The adoption of the robot in the emergency setting does not change the rules of the game. Rather, it enhances the need for a safe and efficient strategy starting from the standardization of the robotic platform setting and docking, up to the execution of the surgical procedure. In order to successfully perform emergency cases with a robotic system, the on-call surgical team must be adequately trained with robotic technology. As reported by Robinson et al. [ 12 ] in a case series of 24 robotic emergency bariatric surgeries, which were compared to 20 laparoscopic procedures, the surgeon who adopted the robotic approach was the same in all cases. It is the proof that a specific attitude of the operator is fundamental. However, it also highlights the need for a “can do” attitude from the entire surgical team [ 28 ]. The importance of the shared viewpoint is reinforced by Sudan et al. [ 24 ] who described the adoption of the robotic platform during the night and during the weekend in order for the staff to be comfortable with this technology. In addition, proper team work and communication in such a challenging workspace are required [ 29 ] as much as the completion of the learning curve for the entire surgical team [ 30 ]. The ideal operating room team in an emergency setting should be made up of the first operating surgeon with an extensive expertise in robotic surgery, an assisting surgeon familiar with the robotic technology, and a scrub nurse dedicated to the robotic program. All team members should work in a simulation environment before starting a robotic emergency surgery program.
Limitations linked to the adoption of robotic surgery in emergency settings are related to the time required for robotic setting and docking and the accessibility of the robotic platform for emergency surgical units. Concerning the time issue, Robinson et al. [ 12 ] reported that, when the entire team is appropriately trained and prepared, the in-room-to-surgery-start time is reduced and has no significant impact on the overall duration of the scheduled emergency procedure. However, in this study, the authors highlighted how the majority of the staff were familiar with the robotic technology, and there were no limitations to its accessibility. This may not be the case for all emergency care units, and trained nursing staff may not be always available during night shifts. A good coordination between the hospital administration, the surgeons, and the staff is the key point to have an efficient and extensive organization for the use of robotic technology, also in emergency surgery scenarios.
Level of evidence: case reports and case series → expert opinion
Strength of consensus (based on the survey evaluation): 100%
PS-2. Robotic surgery in emergency settings may be considered in highly selected clinically stable patients only.
Due to the very limited evidence in the literature and the consensus that robotic surgery required a high level of expertise for the operating surgeon and the entire surgical team, particularly if performed in emergency settings, it should be considered for clinically stable patients only.
A recent review [ 31 ] on the anesthetic aspects of robotic surgery suggested that when the surgical team gains confidence, even more complex operations or patients with comorbidities can be considered candidates for the robotic approach. A precise preoperative assessment based on a case-by-case evaluation, and multidisciplinary decision-making are crucial to guarantee the choice of the most indicated surgical strategy. Even if a comprehensive preoperative assessment is not always possible in emergency situations, a careful patient selection is advised in order not to expose frail or unstable patients to longer emergency procedures or unnecessary complications related to the surgical technique.
Indeed, in unstable patients or patients with cardiopulmonary comorbidities, the adoption of MIS with the need for carbon dioxide insufflation may result in a higher intra-abdominal pressure and hypercarbia with metabolic and respiratory changes which may be deleterious [ 32 ]. Osagiede et al. [ 11 ] showed that the presence of a metastatic disease and the higher number of comorbidities negatively influenced the adoption of MIS in emergency colorectal cancer surgery. Likewise, Arnold et al. [ 33 ] demonstrated that the adoption of MIS is confined to physiologically clinically stable patients while those with abdominal gross contamination or severe infectious processes are more prone to undergo open surgery. Despite this selection bias, when the results are corrected for preoperative risk factors, the adoption of laparoscopy is associated with a reduced wound infection rate, risk of death, and length of hospital stay.
Recently, emergency laparoscopy was evaluated as a valid approach to the treatment of perforated diverticulitis with generalized peritonitis [ 34 ], iatrogenic colonoscopy perforations [ 35 ], and perforated peptic ulcers [ 36 ]. In addition, in simple cases of adhesive small bowel obstruction, a laparoscopic approach may be beneficial despite the considerable risk of conversion to open surgery and the higher probability of bowel injuries [ 37 ]. In all of the abovementioned pathological states, the prerequisite for a safe minimally invasive treatment is the selection of a stable patient.
In terms of anesthetic management in emergency settings, the robotic approach can be considered as an alternative to laparoscopy because it does not change the risk exposure but it may be associated with longer operative times if the surgical team is not properly trained. Additional costs must also be considered. Further studies are necessary in order to clarify the future role of a low pressure pneumoperitoneum in emergency robotic surgery [ 38 ].
Strength of consensus: 94.6%
PS-3. Robotic surgery may be considered in challenging situations, which are foreseen as a reason for conversion to open surgery if operating in laparoscopy.
The available literature suggests that the main potential advantages of robotic surgery over laparoscopy are related to suturing and dissection. In case of emergency robotic surgery, the published studies described the following procedural steps: hiatoplasties [ 20 , 21 ], ventral suturing or mesh fixations [ 25 ], colonic suturing [ 19 ], duodenal stump suturing [ 24 ], strictureplasty [ 24 ], and dissection of inflamed gallbladder [ 22 , 23 ] or colon [ 9 ]. All of these tasks are particularly challenging in laparoscopic surgery and they often lead to conversion to open surgery, which can also be a source of postoperative complications [ 39 , 40 ]. The technological advances of the robotic surgical platform, such as deep magnification, 3D stereoscopic vision, a stable field with elimination of physiological tremors, motion scaling, and improved ergonomics as compared to laparoscopy, may contribute to facilitate the performance of some difficult procedural steps and reduce the risk of conversion. However, this remains to be proven, especially for surgical interventions performed in emergency settings.
Level of evidence: case reports and case series → expert opinion.
Strength of consensus: 83.8% (based on the survey evaluation)
PS-4. In a near future, robotic surgery may offer the advantage of telementoring and telesurgery, which could be useful to promote a safe and standardized application of robotics, also in low-volume centers or specific environments.
One of the limitations of laparoscopic surgery is the absence of telementoring during a difficult procedure. Even if communicating systems dedicated to telementoring are available, no opportunity for the direct control of movements is present in laparoscopy. In robotic surgery, an in-person mentoring can be performed if a second robotic console is present in the hospital (such as telestration or tele-assisted surgery). In a near future, it can be expected to perform telementoring during elective and emergency robotic procedures. After the first transatlantic robot-assisted surgery performed by Jacques Marescaux in 2001 [ 41 ], the surgical community was waiting for a routine use of telesurgery which, however, was not feasible due to technical limitations. Today, thanks to the evolution of telecommunications, namely fifth generation (5G) networks, there is a growing opportunity for a surgeon with a proven expertise in the field to remotely operate on a distant patient [ 42 , 43 ]. A digital connection with a reference center which can evaluate the case, suggest a solution, and eventually manage the surgical situation if need be, represents a powerful tool, especially in emergency settings. Indeed, in emergency surgery where a maximal experience improves outcomes, it would be beneficial to have a mentor observing and remotely participating in the intervention. Additionally, this technology could be applied to provide surgical care to rural areas, to establish surgical collaborations, and to eliminate the shortage of surgeons. This is also applicable for specific environments, such as in the space station, where an emergency medical condition has to be managed by a trained component of the crew, or close to a battlefield, where the surgeon may operate at a safe distance, or again at the bottom of the ocean [ 44 ]. Telesurgery could well be an option in such situations.
However, these applications conceal some limitations in terms of global network development, legal and ethical issues, costs, and cyber security. These issues are under examination. However, despite the current skepticism, it is unquestionable that robotic surgery can have a pivotal role in developing telemedicine and telesurgery [ 45 , 46 ].
Strength of consensus: 89.2% (based on the survey evaluation)
PS-5. The use of robotic surgery for unscheduled and urgent operations needs to be implanted without creating scheduling conflicts in the occupation of the operating room. Moreover, the increased costs need to be justified in the context of an efficient implementation of robotic surgery. Currently, the availability and accessibility of the robotic platforms for emergency care surgical units are very limited.
A consistently growing number of hospitals, mainly tertiary care and university-based hospitals, are acquiring a robotic surgical platform in order to satisfy daily requests and advertise the most advanced technology. The robotic platform is often shared between different specialties, subsequently limited in terms of availability for a single surgical field and not adaptable to changing schedules. In this perspective, several reports suggested that the use of the robotic surgical platform by experienced teams could be prolonged to night hours and even to the weekend. This approach was called “after hours” by Sudan et al. [ 24 ], whose report aimed to highlight the potential of a robotic system which is available 24 h/7 days per week. The availability of the platform during the night shift could potentially favor a more cost-effective use of the robotic system. However, this remains very limited and, as previously highlighted, a proper attitude and excellent training of the entire team are key to guarantee surgical proficiency and efficiently implement robotic surgery for emergency procedures.
Concerns for the adoption of robotics for emergency surgeries also persist in relation to the increased costs that a robotic surgical procedure implies also need to be justified in the context of an efficient implementation of robotic surgery.
PS-6. The development of new modular robotic platforms may contribute to increase the applications of robotic surgery in emergency settings.
The surgical marketplace was recently enhanced with several different robotic platforms either approved for human use, such as the CMR Versius (Cambridge Medical Robotics, Cambridge, UK) and the Distalmotion Dexter (Distalmotion, Epalinges, Switzerland) or under approval, such as the Medtronic Hugo (Medtronic Inc., Minneapolis, USA). Most of them share the opportunity of switching from a conventional laparoscopic setting to a robot-assisted one. This key point, which could be less relevant in elective surgery, should be carefully considered when approaching emergency surgery. In fact, when no specific port placement is required, the surgeon can simply use a different approach depending on the procedural step and on his/her own ability. In addition, these robotic platforms offer an improved vision with advanced near-infrared imaging, not routinely available in laparoscopic surgery. The objective evaluation of tissue anatomy or perfusion could limit the surgical bias in emergency settings by mitigating the personal opinion [ 47 , 48 ].
In the future, advances in surgical technologies will offer multiple new opportunities, which are currently under development, like hyperspectral imaging [ 49 ] and robotic single-port surgery [ 50 ]. Their potential applications and outcomes in emergency surgery need to be evaluated and updated once evidence is available.
Strength of consensus: 94.6% (based on the survey evaluation)
Research agenda
The experts recognized that there is a substantial lack of evidence to support the use of robotic surgery for emerging general surgery procedures. For this reason, a research agenda has been proposed.
Observational (cohort study, case–control) and interventional studies are anticipated to investigate the applications and outcomes of robotic surgery in emergency settings and to compare them with those obtained with laparoscopy and open surgery.
Future studies should evaluate patient preferences considering patient-related outcome measures (PROMs), including pain evaluation and mid-/long-term quality of life.
Future studies should evaluate the cost-effectiveness of robotic surgery implementation in emergency settings at hospital level (e.g., scheduling conflict alleviation) and at the level of the healthcare system (e.g., length of hospital stay, productivity losses, reimbursement systems).
Future studies should evaluate the applicability of the robotic surgical platforms to perform telementoring and telesurgery, which are theoretically promising technologies to expand the applications of robotic surgery.
With the aim to enrich the available evidence and fill knowledge gaps, the WSES plans to launch an open registry on emergency robotic general surgery. The WSES calls for an international participation, which is essential to gather sufficient data and obtain generalizable results.
The establishment of a dedicated registry is also mandatory to perform a deep analysis on this technique, in order to define the following: characteristics of the patient candidate for emergency robotic procedures, operative and postoperative outcomes, PROMs, minimum requisites in terms of personnel and equipment, cost-effectiveness, and ethical issues.
Hospitals that are currently equipped with a robotic surgical platform need to implement it efficiently. The role of robotic surgery for emergency procedures remains under investigation. However, its use is expanding despite the lack of evidence-based guidelines. In this scenario, the WSES wished to provide this position paper to the surgical community. This position paper summarizes the current evidence and practice and proposes consensus statements to be reevaluated and updated as the evidence in the supporting literature emerges. For now, the experts recommend a strict patient selection while approaching emergent general surgery procedures with robotics. However, an emergency setting should not be seen as a contraindication for robotic surgery if adequate training of the operating surgical team is available. When such prerequisites are met, robotic surgery can be considered safe and feasible, and surgical outcomes related to an MIS approach are expected. Finally, the application of the robotic surgical platform may grow with improvements in telementoring and telesurgery, which are particularly valuable in emergency settings.

Availability of data and materials
There are no data from individual authors that reach the criteria for availability.
Abbreviations
- Minimally invasive surgery
Newcastle–Ottawa Scale
Patient-related outcome measures
World Society of Emergency Surgery
Yeo HL, Isaacs AJ, Abelson JS, Milsom JW, Sedrakyan A. Comparison of open, laparoscopic, and robotic colectomies using a large national database: outcomes and trends related to surgery center volume. Dis Colon Rectum. 2016;59:535–42. https://doi.org/10.1097/dcr.0000000000000580 .
Article PubMed Google Scholar
Asbun HJ, Moekotte AL, Vissers FL, Kunzler F, Cipriani F, Alseidi A, et al. The Miami International evidence-based guidelines on minimally invasive pancreas resection. Ann Surg. 2020;271:1–14. https://doi.org/10.1097/sla.0000000000003590 .
Jung M, Morel P, Buehler L, Buchs NC, Hagen ME. Robotic general surgery: current practice, evidence, and perspective. Langenbecks Arch Surg. 2015;400:283–92. https://doi.org/10.1007/s00423-015-1278-y .
Article CAS PubMed Google Scholar
Hanly EJ, Talamini MA. Robotic abdominal surgery. Am J Surg. 2004;188:19s–26s. https://doi.org/10.1016/j.amjsurg.2004.08.020 .
Felder SI, Ramanathan R, Russo AE, Jimenez-Rodriguez RM, Hogg ME, Zureikat AH, et al. Robotic gastrointestinal surgery. Curr Probl Surg. 2018;55:198–246. https://doi.org/10.1067/j.cpsurg.2018.07.001 .
Article PubMed PubMed Central Google Scholar
Liu R, Wakabayashi G, Palanivelu C, Tsung A, Yang K, Goh BKP, et al. International consensus statement on robotic pancreatic surgery. Hepatobiliary Surg Nutr. 2019;8:345–60. https://doi.org/10.21037/hbsn.2019.07.08 .
George EI, Brand TC, LaPorta A, Marescaux J, Satava RM. Origins of robotic surgery: from skepticism to standard of care. JSLS. 2018;22:e2018.00039. https://doi.org/10.4293/jsls.2018.00039 .
de’Angelis N, Notarnicola M, Martínez-Pérez A, Memeo R, Charpy C, Urciuoli I, et al. Robotic versus laparoscopic partial mesorectal excision for cancer of the high rectum: a single-center study with propensity score matching analysis. World J Surg. 2020;44:3923–35. https://doi.org/10.1007/s00268-020-05666-0 .
Anderson M, Lynn P, Aydinli HH, Schwartzberg D, Bernstein M, Grucela A. Early experience with urgent robotic subtotal colectomy for severe acute ulcerative colitis has comparable perioperative outcomes to laparoscopic surgery. J Robot Surg. 2020;14:249–53. https://doi.org/10.1007/s11701-019-00968-5 .
Felli E, Brunetti F, Disabato M, Salloum C, Azoulay D, de’Angelis N. Robotic right colectomy for hemorrhagic right colon cancer: a case report and review of the literature of minimally invasive urgent colectomy. World J Emerg Surg. 2014;9:32. https://doi.org/10.1186/1749-7922-9-32 .
Osagiede O, Spaulding AC, Cochuyt JJ, Naessens JM, Merchea A, Crandall M, et al. Factors associated with minimally invasive surgery for colorectal cancer in emergency settings. J Surg Res. 2019;243:75–82. https://doi.org/10.1016/j.jss.2019.04.089 .
Robinson TD, Sheehan JC, Patel PB, Marthy AG, Zaman JA, Singh TP. Emergent robotic versus laparoscopic surgery for perforated gastrojejunal ulcers: a retrospective cohort study of 44 patients. Surg Endosc. 2021. https://doi.org/10.1007/s00464-021-08447-5 .
Bala M, Kashuk J, Moore EE, Catena F, Leppaniemi A, Ansaloni L, et al. Establishing position papers by the WSES. World J Emerg Surg. 2018;13:1. https://doi.org/10.1186/s13017-018-0163-8 .
Article CAS PubMed PubMed Central Google Scholar
Higgins JPT, Thomas J, Chandler J, Cumpston M, Li T, Page MJ, et al. Cochrane handbook for systematic reviews of interventions. In: Cochrane handbook for systematic reviews of interventions: Cochrane Book Series. 2008.
Moher D, Liberati A, Tetzlaff J, Altman DG. Preferred reporting items for systematic reviews and meta-analyses: the PRISMA statement. BMJ. 2009;339: b2535. https://doi.org/10.1136/bmj.b2535 .
Murad MH, Sultan S, Haffar S, Bazerbachi F. Methodological quality and synthesis of case series and case reports. BMJ Evid Based Med. 2018;23:60–3. https://doi.org/10.1136/bmjebm-2017-110853 .
Stang A. Critical evaluation of the Newcastle-Ottawa scale for the assessment of the quality of nonrandomized studies in meta-analyses. Eur J Epidemiol. 2010;25:603–5. https://doi.org/10.1007/s10654-010-9491-z .
Wells GA, Shea B, O'Connell D, Peterson J, Welch V, Losos M, et al. The Newcastle-Ottawa Scale (NOS) for assessing the quality of nonrandomised studies in meta-analyses. 2011. http://www.ohri.ca/programs/clinical_epidemiology/oxford.asp .
Pedraza R, Ragupathi M, Martinez T, Haas EM. Robotic-assisted laparoscopic primary repair of acute iatrogenic colonic perforation: case report. Int J Med Robot. 2012;8:375–8. https://doi.org/10.1002/rcs.1447 .
Hosein S, Carlson T, Flores L, Armijo PR, Oleynikov D. Minimally invasive approach to hiatal hernia repair is superior to open, even in the emergent setting: a large national database analysis. Surg Endosc. 2021;35:423–8. https://doi.org/10.1007/s00464-020-07404-y .
Ceccarelli G, Pasculli A, Bugiantella W, De Rosa M, Catena F, Rondelli F, et al. Minimally invasive laparoscopic and robot-assisted emergency treatment of strangulated giant hiatal hernias: report of five cases and literature review. World J Emerg Surg. 2020;15:37. https://doi.org/10.1186/s13017-020-00316-1 .
Kubat E, Hansen N, Nguyen HP, Wren SM, Eisenberg D. Urgent and elective robotic single-site cholecystectomy: analysis and learning curve of 150 consecutive cases. J Laparoendosc Adv Surg Tech A. 2016;26:185–91. https://doi.org/10.1089/lap.2015.0528 .
Milone M, Vertaldi S, Bracale U, D’Ambra M, Cassese G, Manigrasso M, et al. Robotic cholecystectomy for acute cholecystitis: three case reports. Medicine (Baltimore). 2019;98: e16010. https://doi.org/10.1097/MD.0000000000016010 .
Article CAS Google Scholar
Sudan R, Desai SS. Emergency and weekend robotic surgery are feasible. J Robot Surg. 2012;6:263–6. https://doi.org/10.1007/s11701-011-0289-0 .
Kudsi OY, Bou-Ayash N, Chang K, Gokcal F. Perioperative and midterm outcomes of emergent robotic repair of incarcerated ventral and incisional hernia. J Robot Surg. 2021;15:473–81.
Panteleimonitis S, Popeskou S, Aradaib M, Harper M, Ahmed J, Ahmad M, et al. Implementation of robotic rectal surgery training programme: importance of standardisation and structured training. Langenbecks Arch Surg. 2018;403:749–60. https://doi.org/10.1007/s00423-018-1690-1 .
Thomas A, Altaf K, Sochorova D, Gur U, Parvaiz A, Ahmed S. Effective implementation and adaptation of structured robotic colorectal programme in a busy tertiary unit. J Robot Surg. 2020. https://doi.org/10.1007/s11701-020-01169-1 .
Schuessler Z, Scott Stiles A, Mancuso P. Perceptions and experiences of perioperative nurses and nurse anaesthetists in robotic-assisted surgery. J Clin Nurs. 2020;29:60–74. https://doi.org/10.1111/jocn.15053 .
Kanji F, Catchpole K, Choi E, Alfred M, Cohen K, Shouhed D, et al. Work-system interventions in robotic-assisted surgery: a systematic review exploring the gap between challenges and solutions. Surg Endosc. 2021;35:1976–89. https://doi.org/10.1007/s00464-020-08231-x .
Smith AL, Scott EM, Krivak TC, Olawaiye AB, Chu T, Richard SD. Dual-console robotic surgery: a new teaching paradigm. J Robot Surg. 2012;7:113–8. https://doi.org/10.1007/s11701-012-0348-1 .
Ashrafian H, Clancy O, Grover V, Darzi A. The evolution of robotic surgery: surgical and anaesthetic aspects. Br J Anaesth. 2017;119:i72–84.
Sharma KC, Brandstetter RD, Brensilver JM, Jung LD. Cardiopulmonary physiology and pathophysiology as a consequence of laparoscopic surgery. Chest. 1996;110:810–5.
Arnold M, Elhage S, Schiffern L, Lauren Paton B, Ross SW, Matthews BD, et al. Use of minimally invasive surgery in emergency general surgery procedures. Surg Endosc. 2020;34:2258–65.
Sartelli M, Weber DG, Kluger Y, Ansaloni L, Coccolini F, Abu-Zidan F, et al. 2020 update of the WSES guidelines for the management of acute colonic diverticulitis in the emergency setting. World J Emerg Surg. 2020;15:32. https://doi.org/10.1186/s13017-020-00313-4 .
de’Angelis N, Di Saverio S, Chiara O, Sartelli M, Martínez-Pérez A, Patrizi F, et al. 2017 WSES guidelines for the management of iatrogenic colonoscopy perforation. World J Emerg Surg. 2018;24:5.
Article Google Scholar
Tarasconi A, Coccolini F, Biffl WL, Tomasoni M, Ansaloni L, Picetti E, et al. Perforated and bleeding peptic ulcer: WSES guidelines. World J Emerg Surg. 2020. https://doi.org/10.1186/s13017-019-0283-9 .
ten Broek RPG, Krielen P, Di Saverio S, Coccolini F, Biffl WL, Ansaloni L, et al. Bologna guidelines for diagnosis and management of adhesive small bowel obstruction (ASBO): 2017 update of the evidence-based guidelines from the world society of emergency surgery ASBO working group. World J Emerg Surg. 2017;2018:13.
Google Scholar
Celarier S, Monziols S, Célérier B, Assenat V, Carles P, Napolitano G, et al. Low-pressure versus standard pressure laparoscopic colorectal surgery (PAROS trial): a phase III randomized controlled trial. Br J Surg. 2021. https://doi.org/10.1093/bjs/znab069 .
Granero L, Cienfuegos JA, Baixauli J, Pastor C, Sánchez Justicia C, Valentí V, et al. Predictive risk factors for postoperative complications and its impact on survival in laparoscopic resection for colon cancer. Surg Laparosc Endosc Percutan Tech. 2021. https://doi.org/10.1097/SLE.0000000000000936 .
Payá-Llorente C, Domingo-del Pozo C, Gonzálvez-Guardiola P, Santarrufina-Martínez S, Pareja-Ibars E, Martínez-Pérez A. Conversion to open surgery during laparoscopic common bile duct exploration: predictive factors and impact on the perioperative outcomes. HPB (Oxford). 2021. https://doi.org/10.1016/j.hpb.2021.05.009 .
Marescaux J, Leroy J, Gagner M, Rubino F, Mutter D, Vix M, et al. Transatlantic robot-assisted telesurgery. Nature. 2001;413:379–80.
Tian W, Fan M, Zeng C, Liu Y, He D, Zhang Q. Telerobotic spinal surgery based on 5G network: the first 12 cases. Neurospine. 2020;17:114–20.
Zheng J, Wang Y, Zhang J, Guo W, Yang X, Luo L, et al. 5G ultra-remote robot-assisted laparoscopic surgery in China. Surg Endosc. 2020;34:5172–80.
Reichenbach M, Frederick T, Cubrich L, Bircher W, Bills N, Morien M, et al. Telesurgery with miniature robots to leverage surgical expertise in distributed expeditionary environments. Mil Med. 2017;182:316–21. https://doi.org/10.7205/milmed-d-16-00176 .
Mohan A, Wara UU, Arshad Shaikh MT, Rahman RM, Zaidi ZA. Telesurgery and robotics: an improved and efficient era. Cureus. 2021;13:e14124.
PubMed PubMed Central Google Scholar
Anvari M, Manoharan B, Barlow K. From telementorship to automation. J Surg Oncol. 2021;124:246–9.
Bronikowski D, Lombardo D, DeLa’O C, Szoka N. Robotic subtotal cholecystectomy in a geriatric acute care surgery patient with super obesity. Case Rep Surg. 2021;2021:9992622.
Liot E, Assalino M, Buchs NC, Schiltz B, Douissard J, Morel P, et al. Does near-infrared (NIR) fluorescence angiography modify operative strategy during emergency procedures? Surg Endosc. 2018;32:4351–6.
Barberio M, Felli E, Seyller E, Longo F, Chand M, Gockel I, et al. Quantitative fluorescence angiography versus hyperspectral imaging to assess bowel ischemia: a comparative study in enhanced reality. Surgery. 2020;168:178–84.
Klazura G, Sims T, Rojnica M, Koo N, Lobe T. Single port robotic splenectomy for pyruvate kinase deficiency in a five-year-old patient, a case report of a surgical first. Int J Surg Case Rep. 2021;84: 106122. https://doi.org/10.1016/j.ijscr.2021.106122 .
Download references
Acknowledgements
The authors are grateful to Guy Temporal and Christopher Burel, professionals in medical English proofreading, for their valuable help.
No funding or resources was received for the preparation of this article. The authors received a WSES institutional waiver for this publication.
Author information
Authors and affiliations.
Unit of Digestive, Hepatobiliary, and Pancreatic Surgery, CARE Department, Henri Mondor University Hospital (AP-HP), Créteil, France
Nicola de’Angelis, Giorgio Bianchi, Filippo Aisoni & Nassiba Beghdadi
Faculty of Medicine, University of Paris Est, UPEC, Créteil, France
Nicola de’Angelis & Nassiba Beghdadi
Department of Colorectal Surgery, Queen Alexandra Hospital, University of Portsmouth, Southwick Hill Road, Cosham, Portsmouth, UK
First Surgical Clinic, Department of Surgical Oncological and Gastroenterological Sciences, University of Padua, Padua, Italy
Francesco Marchegiani
Department of Pediatric Surgery, Spedali Civili Children’s Hospital of Brescia, Brescia, BS, Italy
Daniele Alberti
General Surgery, San Matteo University Hospital, Pavia, Italy
Luca Ansaloni
Division of Trauma and Acute Care Surgery, Scripps Memorial Hospital La Jolla, La Jolla, CA, USA
Walter Biffl
General Surgery and Trauma Team, ASST Niguarda Milano, University of Milano, Milan, Italy
Osvaldo Chiara
General Surgery, San Giovanni Battista Hospital, USL Umbria 2, Foligno, Italy
Graziano Ceccarelli
General, Emergency and Trauma Department, Pisa University Hospital, Pisa, Italy
Federico Coccolini & Enrico Cicuttin
Department of Digestive and Hepatobiliary/Pancreatic Surgery, Groeninge Hospital, Kortrijk, Belgium
Mathieu D’Hondt
Department of Surgery, Cambridge University Hospital, NHS Foundation Trust, Cambridge, UK
Salomone Di Saverio
Digestive and Endocrine Surgery, Nouvel Hôpital Civil, University of Strasbourg, Strasbourg, France
Michele Diana
IRCAD, Research Institute Against Digestive Cancer, Strasbourg, France
Department of General and Metabolic Surgery, Poissy and Saint-Germain-en-Laye Hospitals, Poissy, France
Belinda De Simone
Department of General Surgery, Hospital Valle de Hebron, Universitat Autonoma de Barcelona, Barcelona, Spain
Eloy Espin-Basany
Department of General and Visceral Surgery, Medical Center University of Freiburg, Freiburg, Germany
Stefan Fichtner-Feigl
Department of Surgery, Tel Aviv University, Sackler School of Medicine, Tel Aviv, Israel
Jeffry Kashuk
Department of Surgery, Hospital Group Twente ZGT, Almelo, Netherlands
Ewout Kouwenhoven
Department of Gastrointestinal Surgery, University of Helsinki and Helsinki University Hospital, Helsinki, Finland
Ari Leppaniemi
Unit of Hepato-Pancreato-Biliary Surgery, General Regional Hospital “F. Miulli”, Acquaviva delle Fonti, Bari, Italy
Riccardo Memeo
Department of Clinical Medicine and Surgery, “Federico II” University of Naples, Naples, Italy
Marco Milone
Ernest E Moore Shock Trauma Center at Denver Health, University of Colorado, Denver, CO, USA
Ernest Moore
University of Pittsburgh School of Medicine, Pittsburgh, PA, USA
Andrew Peitzmann
Visceral and Digestive Surgery, Nouvel Hôpital Civil, University of Strasbourg, Strasbourg, France
Patrick Pessaux
Institute for Image-Guided Surgery, IHU Strasbourg, Strasbourg, France
Institute of Viral and Liver Disease, INSERM U1110, Strasbourg, France
3Rd Department of Surgery, Attikon General Hospital, National and Kapodistrian University of Athens (NKUA), Athens, Greece
Manos Pikoulis
1St General Surgery Unit, Department of Emergency, ASST Papa Giovanni Hospital Bergamo, Bergamo, Italy
Michele Pisano
Division of Digestive Surgery, University Hospitals of Geneva and Medical School, Geneva, Switzerland
Frederic Ris
Department of Surgery, Macerata Hospital, Macerata, Italy
Massimo Sartelli
IRCAD Faculty Member Robotic and Colorectal Surgery-IRCAD, Strasbourg, France
Giuseppe Spinoglio
Department of Surgery, Letterkenny University Hospital, Donegal, Ireland
Michael Sugrue
Department of Surgery, Trauma Surgery, Radboud University Medical Center, Nijmegen, Netherlands
Department of HBP Surgery, University Hospitals Coventry and Warwickshire NHS Trust, Clifford Bridge Road, Coventry, CV2 2DX, UK
Paschalis Gavriilidis
Department of Trauma Surgery, Royal Perth Hospital, Perth, Australia
Dieter Weber
Department of General Surgery, Rambam Healthcare Campus, Haifa, Israel
Yoram Kluger
Department of General and Emergency Surgery, Bufalini Hospital-Level 1 Trauma Center, Cesena, Italy
Fausto Catena
You can also search for this author in PubMed Google Scholar
Contributions
GB, FM, and NdeA conducted the systematic review of the literature and wrote the first draft of the manuscript. All authors were involved in the statement evaluation and consensus process. All authors critically reviewed the manuscript and approved the final version. All authors read and approved the final manuscript.
Corresponding author
Correspondence to Nicola de’Angelis .
Ethics declarations
Ethics approval and consent to participate.
Not applicable.
Consent for publication
Competing interests.
P Pessaux declared that he received consulting fees from 3M and Integra and has stock-options of Virtualisurg. E Kouwenhoven is proctor for Intuitive Surgical. M Sugrue received consulting fee with 3M, Smith and Nephew and Novus Scientific. G Spinoglio received honoraria as proctor for Intuitive Surgical. F. Ris reports research funding from Quantgene, personal fees from Arthrex, Stryker, Hollister, Fresenius Kabi and Distal Motion, outside the submitted work. E Espin Bsany received honoraria as proctor for Intuitive Surgical. JS Khan is a proctor for Intuitive Surgical. All other authors have no conflicts of interest to declare in relation to the matter of this publication.
Additional information
Publisher's note.
Springer Nature remains neutral with regard to jurisdictional claims in published maps and institutional affiliations.
Rights and permissions
Open Access This article is licensed under a Creative Commons Attribution 4.0 International License, which permits use, sharing, adaptation, distribution and reproduction in any medium or format, as long as you give appropriate credit to the original author(s) and the source, provide a link to the Creative Commons licence, and indicate if changes were made. The images or other third party material in this article are included in the article's Creative Commons licence, unless indicated otherwise in a credit line to the material. If material is not included in the article's Creative Commons licence and your intended use is not permitted by statutory regulation or exceeds the permitted use, you will need to obtain permission directly from the copyright holder. To view a copy of this licence, visit http://creativecommons.org/licenses/by/4.0/ . The Creative Commons Public Domain Dedication waiver ( http://creativecommons.org/publicdomain/zero/1.0/ ) applies to the data made available in this article, unless otherwise stated in a credit line to the data.
Reprints and permissions
About this article
Cite this article.
de’Angelis, N., Khan, J., Marchegiani, F. et al. Robotic surgery in emergency setting: 2021 WSES position paper. World J Emerg Surg 17 , 4 (2022). https://doi.org/10.1186/s13017-022-00410-6
Download citation
Received : 15 October 2021
Accepted : 27 November 2021
Published : 20 January 2022
DOI : https://doi.org/10.1186/s13017-022-00410-6
Share this article
Anyone you share the following link with will be able to read this content:
Sorry, a shareable link is not currently available for this article.
Provided by the Springer Nature SharedIt content-sharing initiative
- Emergency surgery
- Robotic surgery
- General surgery
World Journal of Emergency Surgery
ISSN: 1749-7922
- Submission enquiries: [email protected]
The emerging role of robotics in plastic and reconstructive surgery: a systematic review and meta-analysis
- Open access
- Published: 15 June 2024
- Volume 18 , article number 254 , ( 2024 )
Cite this article
You have full access to this open access article
- Laura Awad 1 , 2 , 3 ,
- Benedict Reed 1 , 2 ,
- Edward Bollen 1 ,
- Benjamin J. Langridge 1 , 2 , 3 ,
- Sara Jasionowska 1 , 2 ,
- Peter E. M. Butler 1 , 2 , 3 &
- Allan Ponniah 1 , 2
195 Accesses
Explore all metrics
The role of robotics has grown exponentially. There is an active interest amongst practitioners in the transferability of the potential benefits into plastic and reconstructive surgery; however, many plastic surgeons report lack of widespread implementation, training, or clinical exposure. We report the current evidence base, and surgical opportunities, alongside key barriers, and limitations to overcome, to develop the use of robotics within the field. This systematic review of PubMed, Medline, and Embase has been conducted in accordance with the Preferred Reporting Items for Systematic Reviews and Meta-Analyses (PROSPERO (ID: CRD42024524237). Preclinical, educational, and clinical articles were included, within the scope of plastic and reconstructive surgery. 2, 181, articles were screened; 176 articles met the inclusion criteria across lymph node dissection, flap and microsurgery, vaginoplasty, craniofacial reconstruction, abdominal wall reconstruction and transoral robotic surgery (TOR). A number of benefits have been reported including technical advantages such as better visualisation, improved precision and accuracy, and tremor reduction. Patient benefits include lower rate of complications and quicker recovery; however, there is a longer operative duration in some categories. Cost presents a significant barrier to implementation. Robotic surgery presents an exciting opportunity to improve patient outcomes and surgical ease of use, with feasibility for many subspecialities demonstrated in this review. However, further higher quality comparative research with careful case selection, which is adequately powered, as well as the inclusion of cost-analysis, is necessary to fully understand the true benefit for patient care, and justification for resource utilisation.
Similar content being viewed by others
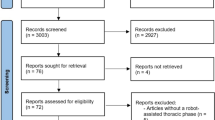
Short-term outcomes of robot-assisted versus conventional minimally invasive esophagectomy for esophageal cancer: a systematic review and meta-analysis of 18,187 patients
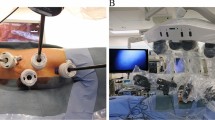
Introduction of a new surgical robot platform “hinotori™” in an institution with established da Vinci surgery™ for digestive organ operations
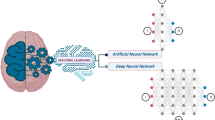
The usefulness of artificial intelligence in breast reconstruction: a systematic review
Avoid common mistakes on your manuscript.
Introduction
The role of robotics has grown exponentially. Robotic surgery, also known as robotic-assisted surgery, allows for complex minimally invasive surgical procedures to be completely or part-performed with a mechanical system consisting of articulating arms, typically controlled at a separate console by the surgeon.
The Da Vinci Surgical Robotic System (Intuitive Surgical, Sunnyvale, CA, USA), has been widely implemented in various surgical specialities, such as general surgery, urology, and gynaecology, within 66 countries. A recent systematic review of laparoscopic and robotic surgery found comparable or improved complication rates with robotic surgery, with reduced recovery time and length of stay [ 1 ].
Robotic consoles can offer accuracy, and precision, as well as minimally invasive access to difficult areas, with improved visualisation. Surgeons have better ergonomic performance, with a reduction in mental and physical workload [ 2 ]. Additionally, wireless connection broadens opportunities within telesurgery to facilitate remote operating [ 3 ].
The application of robotic surgery in clinical plastic and reconstructive practice is yet to be well established [ 4 ]. There is an active interest amongst practitioners in the transferability of these potential benefits into a speciality that works in collaboration with many surgical disciplines; however, many plastic surgeons report lack widespread implementation or exposure [ 5 ]. Whilst Da Vinci Surgical Robotic System (Intuitive Surgical, Sunnyvale, CA, USA) is the most well-known resource, MUSA Microsure (Science Park Eindhoven, Netherlands) and Symani Surgical System (Medical Microinstruments, Italy) are competitors in the market, particularly for use within microsurgery (Fig. 1 ).
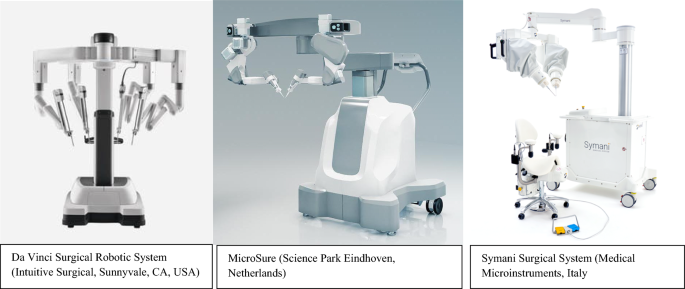
Robotic equipment utilised within clinical practice. All three are controlled by a separate master console
Microsurgery is an area which requires high precision, excellent magnified visualisation, and tremor reduction. Whilst robotic surgery may exceed in these domains, the impact of loss of haptic feedback requires investigation. There are other potential barriers within the widespread implementation of robotics and robotic-assisted surgery within plastic and reconstructive surgery such as the financial incurrence and sparce training opportunities [ 5 ].
The aims of this systematic review are to assess the feasibility of robotic surgery within plastic and reconstructive surgery and review the barriers and limitations to clinical implementation and training.
This systematic review has been conducted in accordance with the Preferred Reporting Items for Systematic Reviews and Meta-Analyses (PRISMA) [ 6 ]. Methodology was designed a priori, and this review is registered with PROSPERO (ID: CRD42024524237).
A literature search of PubMed, Medline and Embase for publications within the past 10 years was conducted by author L.A. Additional articles found through reference screening were included. Titles and abstracts were screened by two independent authors (B.R and E.B), with discrepancies for inclusion reviewed by a third author independently (L.A). This review includes all study types such as randomised controlled trials (RCT), prospective cohort, retrospective cohort, case series/reports, case–control, cross-sectional studies and preclinical studies.
Eligibility criteria
Articles were accepted for inclusion using the following criteria:
Patients/populations who have undergone robotic surgery for reconstruction or oncological resection, within the scope of plastic and reconstructive surgery.
Adults and children
Articles which described robotic procedures within the scope of plastic and reconstructive surgery
Preclinical and educational studies within the scope of robotic plastic and reconstructive surgery including animal, synthetic and cadaveric models.
Articles were excluded from this review using the following criteria:
Articles pertaining to robotic surgery outside the scope of plastic and reconstructive surgery.
Inguinal hernia repair
Articles not available in English language
Articles published prior to 2013.
Search strategy
Search strategy employed is described below. Key words and subject headings were combined using Boolean logic and refined with consensus from all authors:
Micro* OR reconstruct* OR flap OR nerve OR anastomosis OR abdominal wall OR pelvic floor OR supermicrosurg* OR head and neck OR oral OR oropharyngeal OR vaginoplasty OR breast OR nasal OR plastic
Data metrics
Data were tabulated into a predetermined Excel spreadsheet by authors LA and E.B [ 7 ]. This was subsequently refined following a pilot collection with a random sample of papers. Articles upon paper review which were deemed not suitable for inclusion were discussed with an independent third party (B.L). Data items obtained included article characteristics (title, author, year, journal, impact factor, type of study, multicentre/single centre), demographics (number of participants, gender, age, control), procedure (subspeciality, specific task, robot, ports, location of ports), and outcomes (operative duration, length of Stay, blood loss, peri-operative complications, long-term outcomes, follow-up duration, learning curve, and cost).
Risk of bias
Risk of bias was assessed by authors LA and E.B. RCT’s were reviewed using Cochrane’s risk of bias tool (RoB 2) [ 8 ]. Non-randomised trials was assessed using Cochrane’s ROBINS-I tool [ 9 ]. The Joan Briggs Institute Critical Appraisal Checklist for Case Series and the Joan Briggs Institute Critical Appraisal Checklist for Case Reports was used to review case series and case reports, respectively. [ 10 , 11 ] A report of bias is included in the appendices.
Data synthesis
Narrative synthesis, and quantitative analysis was performed where possible. Descriptive analysis of continuous data is represented with ranges, mean values, or overall rate. Categorical data is presented with percentage prevalence. Subcategories are defined by subspeciality and procedure.
Study characteristics were tabulated and compared against planned subgroups to determine their suitability for each synthesis. Nonparametric data were analysed using a Wilcoxon test or an unpaired T test. Forrest plots were constructed, (in subcategories with article number > 5, where possible), using odds ratios for dichotomous and continuous outcomes and heterogeneity tested for using Chi-square and I 2 test. Statistical analysis was performed using RevMan Software [ 12 ].
The literature search yielded a total of 2181 articles (Fig. 2 ). Following abstract screening, a total of 176 articles were included in this systematic review. A total of 149 clinical articles were found (Table 1 ). A total of 11 preclinical articles were included (Two of which also included clinical data) (Table 2 ) [ 13 , 14 , 15 , 16 , 17 , 18 , 19 , 20 , 21 , 22 , 23 ]. A total of 18 educational articles were included (Table 2 ) [ 24 , 25 , 26 , 27 , 28 , 29 , 30 , 31 , 32 , 33 , 34 , 35 , 36 , 37 , 38 , 39 , 40 , 41 ].
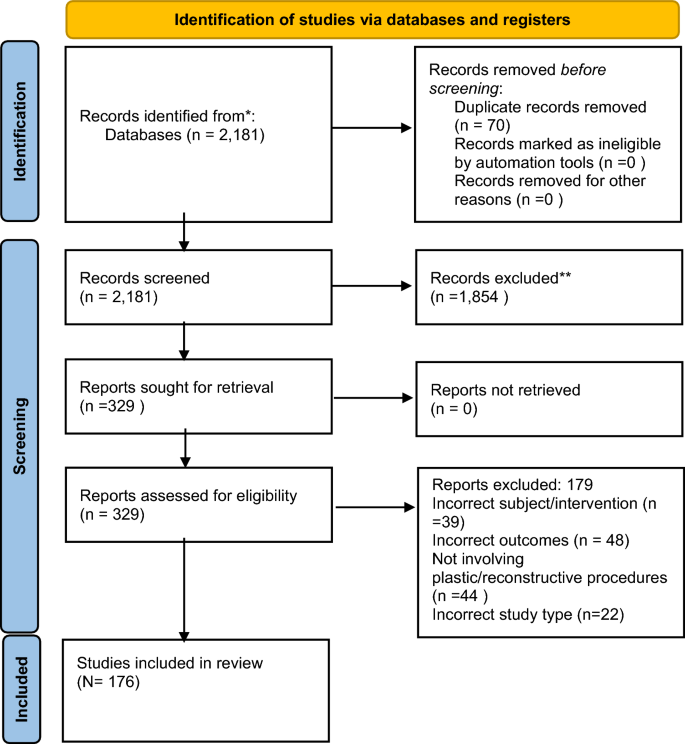
PRISMA flow diagram of the literature search for robotics in plastic and reconstructive surgery
Clinical articles were subcategorised by subspeciality (Fig. 3 ). A total of 11 articles described robotic lymph node dissection [ 13 , 42 , 43 , 44 , 45 , 46 , 47 , 48 , 49 , 50 , 51 ]. A total of 21 articles described robotic pedicled or free flap harvest [ 52 , 53 , 54 , 55 , 56 , 57 , 58 , 59 , 60 , 61 , 62 , 63 , 64 , 65 , 66 , 67 , 68 , 69 , 70 , 71 , 72 ]. A total of eight articles described robotic flap pedicle or vessel dissection [ 73 , 74 , 75 , 76 , 77 , 78 , 79 , 80 ]. 16 articles detailed robotic free flap inset or anastomosis (vessel, nerve and lymphovascular) [ 34 , 81 , 82 , 83 , 84 , 85 , 86 , 87 , 88 , 89 , 90 , 91 , 92 , 93 , 94 , 95 ]. Two articles described robotic craniofacial techniques (mandibular contouring) [ 96 , 97 ]. One cohort study described a robotic cleft palate surgery [ 98 ]. One case report described robotic nerve decompression [ 99 ]. Three articles described vaginoplasty/gender reassignment robotic techniques [ 100 , 101 , 102 ]. A total of 28 articles described ventral abdominal wall reconstruction and hernia repair [ 103 , 104 , 105 , 106 , 107 , 108 , 109 , 110 , 111 , 112 , 113 , 114 , 115 , 116 , 117 , 118 , 119 , 120 , 121 , 122 , 123 , 124 , 125 , 126 , 127 , 128 , 129 , 130 ]. A total of 18 articles pertained to robotic mastectomy [ 56 , 72 , 131 , 132 , 133 , 134 , 135 , 136 , 137 , 138 , 139 , 140 , 141 , 142 , 143 , 144 , 145 , 146 ]. Finally, a total of 43 articles described transoral robotic surgery (TOR) [ 81 , 147 , 148 , 149 , 150 , 151 , 152 , 153 , 154 , 155 , 156 , 157 , 158 , 159 , 160 , 161 , 162 , 163 , 164 , 165 , 166 , 167 , 168 , 169 , 170 , 171 , 172 , 173 , 174 , 175 , 176 , 177 , 178 , 179 , 180 , 181 , 182 , 183 , 184 , 185 , 186 , 187 , 188 ].
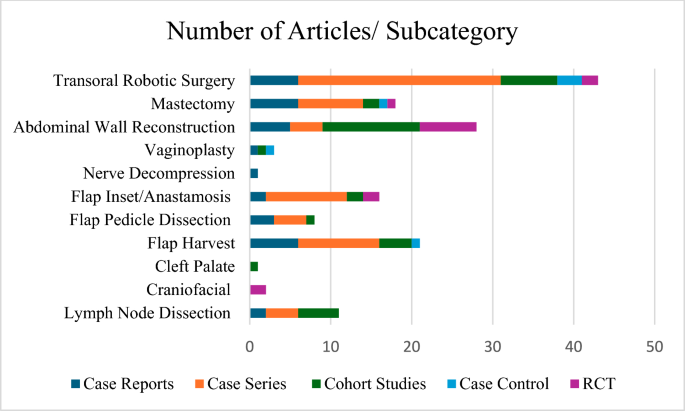
Total number of articles in each subcategory within the scope of robotic plastic and reconstructive surgery
Peri-operative outcomes
Lymph node dissection.
Reported length of stay, complications, and recurrence (of disease) are displayed in Table 3 . Six articles found the average operative time to be higher for robotic surgery (Table 1 ). The peri-operative complication rate was found to be comparable, within the reported studies. The average length of stay was shorter for robotic surgery; however, only two articles reported length of stay for conventional lymph node dissection ( P = 0.46).
Pedicled and free flap harvest
Peri-operative outcomes regarding pedicled and free flap harvest are reported in Table 4 . Average harvest time is higher in the robotic group, although not this was not statistically significant. Average length of stay within comparative studies is lower in the robotic group; however, overall results show a comparable length of stay with conventional surgery. Overall, average complication rates are lower than conventional approaches; however, not statistically significant within comparative studies ( P = 0.061).
Microsurgery
Peri-operative outcomes for flap pedicle dissection, flap inset, and microsurgical anastomosis are shown in Table 5 . No comparative studies were found for pedicle dissection, with majority of articles pertaining to deep inferior epigastric perforator (DIEP) pedicle dissection. Anastomosis time was found to be longer for robotic surgery; however, docking time was not reported in any studies. There was a comparable rate of overall complications. Only three non-comparative studies reviewed length of stay, with the average being 7.1 days.
Peri-operative outcomes regarding nipple-sparing mastectomy are shown in Table 6 . Operative time was found to be comparable overall; however, this included reconstruction time. Overall length of stay was comparable between open and robotic groups. Overall rate of complication was lower in robotic nipple-sparing mastectomy ( P = 0.0007) (Fig. 4 ).
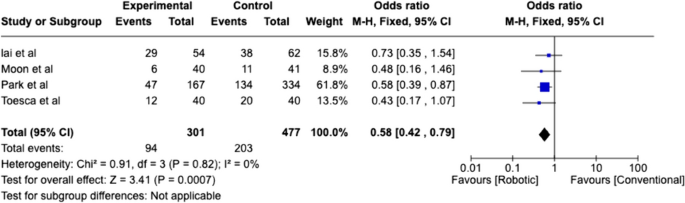
Weighted analysis of comparative studies reviewing complication rate of robotic nipple sparing mastectomy with conventional nipple sparing mastectomy
Abdominal wall
Outcomes regarding abdominal wall reconstruction are collated in Table 7 . Separate comparisons are demonstrated between robotic versus laparoscopic, and robotic versus open repair. Weighted analysis of comparative robotic versus laparoscopic studies found high heterogeneity (85%), and favours robotic surgery with reduced complications ( P = 0.02) (Fig. 5 ). Robotic surgery had fewer complications when compared with open surgery ( P = 0.0001), with lower heterogeneity (Fig. 6 ).
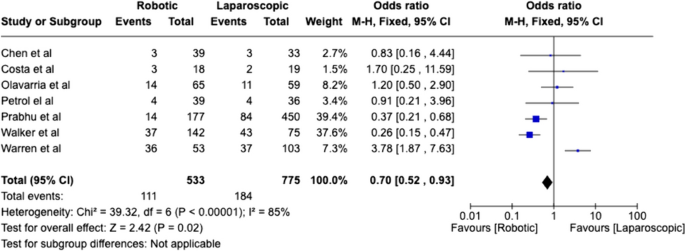
Weighted analysis of comparative studies reviewing complication rate between robotic abdominal wall reconstruction and laparoscopic abdominal wall reconstruction
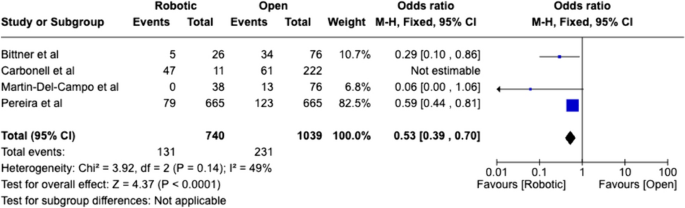
Weighted analysis of comparative studies reviewing complication rates in robotic versus open abdominal wall reconstruction
Length of stay was shorter for robotic surgery in comparison to both groups, however, was only statistically significant for robotic versus open ( P = 0.017). Overall operative time was higher for robotic surgery but was not statistically significant within laparoscopic and open subgroups.
Transoral robotic surgery
TOR operative outcomes are reported in Table 8 . Length of stay was shorter for robotic surgery; however, this was not statistically significant. A statistically significant lower rate of complications is found for robotic surgery in comparison to open surgery ( P = 0.033). Disease-free survival was higher within the robotic cohort; however, this was not found to be statistically significant.
Operative time was variable, and few conclusions can be drawn (Table 1 ). Lee et al. reported a longer duration compared to transoral resection; however, White et al., found a shorter duration for excision of recurrent oropharyngeal SCC [ 151 , 154 ]. White et al., also found a better rate of negative margins with robotic surgery [ 154 ]. Hammoudi et al. found no difference in procedure duration for resection of primary SCC [ 158 ].
Post-operative outcomes
Patient-reported outcomes and long-term outcomes are reported in Table 1 . The quality and standard of assessment varied greatly. Patient satisfaction was reported in three (27%) lymph node (neck) dissection articles, all of which found better scores compared to open with regard to cosmesis and scarring [ 42 , 43 , 44 ]. Lin et al. found comparable results for patient satisfaction and pain for mandibular contouring [ 96 ].
Flap/microsurgery
High patient satisfaction for latissimus dorsi muscle flap harvests were reported in three articles; one cohort study found significantly higher BREAST-Q scores than open [ 54 , 66 , 70 ]. 31% of flap inset or anastomosis articles reported post-operative outcomes other than complications [ 84 , 85 , 87 , 91 , 93 ]. Van Mulken et al. reported robotic lymphovascular anastomosis to have comparable lymph ICF scores to conventional microsurgery. Miyamoto et al. and Chen et al. reported successful patient outcomes of nerve grafts (sympathetic trunk reconstruction and nerve to deltoid) [ 84 , 93 ]. Two articles detailing pedicle dissection of DIEP flaps reported favourable outcomes, and no hernias; however, there are no comparative results [ 73 , 77 ].
Abdominal wall reconstruction
Patient-reported outcome measures (PROMs) were described in 8 (28.6%) articles of abdominal wall reconstruction. Three articles reviewed pain with VAS scores and found no difference (2 RCT’s) or less pain at 1 month/1 year (prospective cohort) [ 116 , 120 , 123 ]. Kakela et al. found comparable PROMs (SF-36) with laparoscopic surgery, with high scores for emotional status and social function for robotic surgery. Three articles found no difference between robotic and laparoscopic surgery in reported patient outcomes, including functional status [ 120 , 122 , 126 ]. One RCT found higher HERqLess scores for robotic versus laparoscopic ventral mesh hernia repair [ 118 ].
One RCT compared robotic extraperitoneal versus intraperitoneal onlay mesh (IPOM) for ventral hernia repair and found that IPOM had significantly higher HerQLess scores at 1 year follow-up.
A total of four (22.2%) of mastectomy articles reported patient qualitative outcomes. Two articles reported high scores for cosmetic satisfaction with minimal scarring, whilst one case control study found significantly higher scores in a cosmetic outcome questionnaire than open surgery, with better scarring and a better position of the nipple–areolar complex [ 132 , 133 , 134 , 143 ]. One RCT documented significantly higher satisfaction within the BREAST-Q questionnaire for robotic surgery [ 144 ].
Three TOR studies reported a lower rate of tracheostomies in the peri-operative period, as well as a lower requirement and durations of nasogastric feeding/PEG feeding [ 154 , 155 , 158 ].
Two studies found significantly higher 3-year disease-free survival with robotic surgery in HPV negative patients, and comparable rates of survival for HPV positive patients for oropharyngeal SCC primary resection [ 157 , 173 ]. This was echoed by Lee et al., in which robotic surgery had a higher overall and disease-free survival rate at 2 years for lateral oropharyngectomy as treatment for tonsillar cancer [ 151 ]. White et al. found a higher rate of 2-year disease-free survival for open surgery to treat recurrent oropharyngeal SCC (T1-T4) [ 155 ].
Two articles evaluated patient outcomes through the Head and Neck Cancer Inventory (HCNI); Durmus et al. reported patients to have highly functional quality of life within their case series of carcinoma of unknown primary resection [ 156 ]. Sethia et al. found comparable outcomes for robotic oropharyngeal resection with and without adjuvant therapy [ 175 ]. Lee et al. also reported no difference in VHI and MDADI scores between open and robotic lateral oropharyngectomy for tonsillar cancer [ 151 ].
Gundlapalli et al. reported a higher procedural cost for their case report of a robotic-assisted DIEP breast reconstruction of $16,000 versus $14,000. There were no other articles which reported cost within robotic flap harvest or microsurgery.
Lai et al. reported a higher cost for robotic nipple-sparing mastectomy in comparison to conventional treatment of $10, 877 versus $5,702 [ 143 ].
Within the subcategory of abdominal wall reconstruction three articles (11%) reported cost. Olavarria et al. found robotic patients had an increased total cost for 90 days of care in comparison to laparoscopic ventral mesh hernia repair in their RCT ($15, 865 robotic versus $12, 955) [ 116 ]. In addition to this, a separate RCT found that whilst the cost of reusables was comparable between robotic and laparoscopic ventral hernia repair, the total cost was significantly higher for robotic patients due to the overall operative time (Cost ratio of 1.13 robotic versus laparoscopic 0.97 P = 0.03) [ 125 ]. In contrast a retrospective cohort study found whilst the procedure costs were higher for robotic surgery, the overall cost of patient care was shorter because of reduced length of hospital stay (robotic $13, 943 versus $19, 532, P = 0.07) [ 109 ].
Two TOR articles reported cost (4.7%). Chung et al. found that overall cost was significantly lower for robotic pharyngectomy ($20,706 versus $29,365) and posterior partial glossectomy ($19, 091 versus $23,414), whilst anterior partial glossectomy demonstrated no difference in the total cost of procedure between TOR and conventional approaches ($22,111 versus $21,376) [ 155 ]. Hammoudi et al. reported higher costs for robotic oropharyngeal SCC resection; however, the overall cost accounting for duration of hospital stay was significantly less ($20,885 vs $27,926) [ 158 ].
Learning curve
Learning curve was reported in clinical studies as changes in operative time (Table 1 ). Three abdominal wall reconstruction articles commented that skin-to-skin operating time decreased throughout their cohort [ 112 , 116 , 120 ]. Muysoms et al. analysed operative time for 41 transabdominal retromuscular hernia repairs, and commented that the decrease was largely contributed to by improved efficacy in the dissection aspect of the procedure [ 112 ]. Olavarria et al. reported a training exposure of 50 cases, through simulation and cadaveric models, prior to performing ventral hernia repairs was necessary to ensure optimal clinical practice [ 116 ]. A total of four mastectomy articles reported operative time to decrease with as clinical exposure increased, including a decrease in docking time [ 132 , 133 , 134 , 139 , 142 ]. Lai et al. achieved an average time for nipple-sparing mastectomy of 100 min, in a series of 39 patients [ 142 ].
Van Mulken et al. reported robotic microvascular anastomosis to require a longer time to complete; however, a steep learning curve resulted in a reduction in this [ 87 ]. Barbon et al. also reported a steep learning curve for anastomosis with time taken to complete being comparable to hand-sewn operative time, with the quickest robotic anastomosis taking around 10 min (Table 2 ) [ 89 ].
Selber et al. also reported a steep learning curve in surgical trainees over five sessions, followed by gradual improvement [ 29 ]. Two training models in microvascular anastomosis reported a plateau in learning curve of robotic anastomosis by expert surgeons on synthetic silicone vessels and rat vessels to be 5 and 8 attempts, respectively [ 16 , 17 ]. Beier et al. developed a 4-week training programme with synthetic 1 and 2 mm vessels, in which 10 successful anastomosis were deemed to be the benchmark for skill acquisition before progression to clinical practice [ 34 ].
Surgical ease of use
Robotic surgery offers several mechanical advantages to aid surgical performance. Many authors commented upon improved visibility with higher 3-dimensional resolution, magnification, and lighting, allowing for depth of field perception and a 360° view of a cavity [ 54 , 132 ].
The Da Vinci robotic arms have 7° of freedom which allow for higher dexterity and greater range of motion, optimising the user’s ability to dissect the surgical plane and increasing access to difficult anatomical areas [ 137 ].
Insufflation was found to be useful attribute for nipple-sparing mastectomy [ 131 , 135 , 141 ]. Through a single small incision approach, Toesca et al. reported easy identification of structures such as intercostal perforators which contribute to nipple–areolar complex survival and flap survival, and better view of the surgical plane [ 132 ]. The use of carbon dioxide helped to reduce bleeding and perform better haemostasis [ 132 ]. There was a higher surgical challenge with larger ptotic breasts [ 136 ]. Motion scaling, and tremor filtration provides high precision and stability; this was also found to be advantageous for flap and microsurgery [ 77 , 132 , 137 ].
The robotic technique of pedicle dissection of the DIEP flap minimizes incision of the anterior rectus muscles and provides improved dexterity and motion; however, due to the space occupation of the robot and the console it may be challenging for two surgical teams to work simultaneously, thus potentially increasing operative duration [ 73 , 77 , 80 ].
Robotic equipment also eliminates haptic feedback; however, users have reported that they were able to compensate effectively for this by relying on visual cues and felt able to complete the vessel and lymphovascular anastomosis without difficulty [ 83 , 85 , 90 ].
Feng et al. reviewed tremor during microsurgery, based on instrument tip movement and found that this was significantly lower in robotic surgery in an ex vivo model [ 14 ]. Furthermore, in a simulation model of 1 mm synthetic vessels, robotic anastomosis was performed with greater precision (measured in suture distance and angulation) when compared with manual approaches for 40 expert surgeons and 20 novices [ 17 ].
This study demonstrates feasibility and safety of robotic surgery within plastic and reconstructive surgery in several subcategories. There are clear benefits to the surgeon, as described above, with improved access to difficult areas, tremor reduction and motion scaling, and improved ergonomic efficiency [ 2 ].
These attributes are particularly useful in cavity surgery and could create opportunities to complete challenging procedures which could not be accessed through an open approach due to narrow openings, such as nasopharyngeal resection and microvascular reconstruction, or where there may be a high risk of complications, or prolonged recovery time associated with conventional open approaches.
One example of this is TOR, whereby access and exposure is often obtained through techniques with higher morbidity, such as mandible splitting, leading to specific complications and expectations for recovery outside of the intended resection. Furthermore, although DIEP flap harvest can be regarded as having more superficial access, Tsai et al. found the anterior rectus sheath incision for pedicle dissection to be significantly smaller than conventional approaches, and thus less invasive [ 79 ]. It is not yet clear if this translates to reduced hernia occurrence post-operatively.
As interest within microsurgery grows, Da Vinci, and other companies such as Symani Surgical Systems and Microsure, have created an instrument portfolio that is well adapted to this field. Literature shows these tools can perform vessel, nerve and lymphovascular anastomosis with non-inferior outcomes to conventional approaches. Improved surgical ergonomics has allowed end-to-end anastomosis of 1 mm diameter vessels as reported in preclinical studies, with higher ease [ 16 ]. Whilst nerve repair can be performed robotically, there is lack of substantial evidence or comparison to conventional approaches. Whilst this approach is more minimally invasive, further research to determine the overall benefit, safety and cost would be beneficial.
Loss of haptic feedback is often considered to be disadvantage of robotic surgery. Surgeons have reported a compensation for this by relying on visual cues which has not impacted their performance. Further research could assess how easily a surgeon may adapt to the loss of true haptic feedback, as well as looking into the incorporation of haptic feedback into robotic instruments.
Single port access is highly advantageous for breast surgery including resection and reconstruction. Quicker docking can reduce operative time and the smaller incision offers a better cosmetic outcome with reduced scarring [ 101 ].
The high precision and accuracy of robotic surgery, could improve patient care, reflected in the lower rate of complications reported, reduced blood loss, reduced post-operative pain, as well as the comparable or reduced length of recovery. Whilst operative time is reported to be higher for robotics, many centres have shown a learning curve in adapting to new techniques.
There is a paucity of data evaluating patient reported outcomes within the literature. Outcomes within case series/case reports were often reported anecdotally, without use of validated or quantitative assessment tools. However, several articles have reported high patient satisfaction with regard to cosmetic outcome and scarring. Robotic neck dissection approach has been performed with a smaller retro-auricular incision.
Furthermore, robotic latissimus dorsi muscle flap harvest and radial forearm flap harvest can offer reduced scarring through a more minimally invasive approach, resulting in absence of long scars, on the back and forearm, respectively. Whilst this is the case, compared to open techniques, insufflation with reduced scarring, can also be achieved with an endoscopic approach. A comparison of the benefits to the surgeon and patient between endoscopic and robotic-assisted technique would be valuable to ascertain the true benefit of robotic assistance in this procedure. Some patients may require incorporation of skin within an LD flap for example in salvage procedures, or delayed reconstruction of the irradiated breast. The quality of coverage at the recipient site may be insufficient to accommodate the optimal reconstructive outcome, with particular importance of the integrity of the lower pole. In these circumstances, robotic surgery may present few advantages for LD flap harvest, and thus patient selection is important.
Patients undergoing robotic nipple-sparing mastectomy and reconstruction have also reported a higher scar satisfaction, with the use of a single incision in the axilla, in which multiple robotic arms can be used. There is a clear benefit to procedures in which access can move towards less invasive approaches, and robotic surgery within breast reconstruction and lymph node dissection are promising avenues for future research.
The rate of hernia recurrence within abdominal wall reconstruction is challenging to ascertain given the variable and often short length of follow-up reported within the literature. The mean length of follow-up within this subcategory is 9 months (0.25–33.6 months).
There is a high variance of histopathology within the transoral robotic surgery subcategory, as well as tumour location, stage of disease, and patient demographics. Few conclusions can be drawn between the comparative studies given the variability. However, the results reported, suggest that TOR results in non-inferior patient outcomes in comparison to conventional approaches.
Cost is poorly reported within the literature. Cost-analysis of robotic reconstructive procedures to review total cost of patient care would be beneficial in ascertaining the economic barriers that prevent the implementation of robotic within clinical practice in this speciality. Reasons suggested for higher cost include the initial purchase of robotic equipment, and prolonged operative duration utilising resources [ 118 ].
However, several articles within abdominal wall reconstruction and TOR, have reviewed the total cost of patient care, and found that the overall financial burden is significantly less than conventional approaches after accounting for length of hospital stay. This could be because of fewer complications, and reduced pain with a minimally invasive approach [ 109 , 155 , 158 ]. Chung et al. also reported reduced requirement of tracheostomies, nasogastric feeding, and percutaneous endoscopic gastrostomy (PEG) feeding, which could account for a decrease in overall consumables cost. Several articles have also described a learning curve throughout their studies, reflected in a shorter operative duration, which could have an impact for cost incurred. The cost of training surgeons, and theatre teams to use robotic equipment should also be accounted for.
Whilst the initial cost may be high for robotic surgery, the overall cost may be offset by the reduction in complication rate, and reduced length of stay. It is important to delineate when and where the cost of robotics, including resource utilisation, is balanced by proven improved patient outcomes in order to implement this effectively in future practice.
All studies which report a learning curve in this review, do so indirectly, as a reduction in operative time [ 142 ]. Whilst a reduction in the time taken to perform the procedure can be seen as an improvement in skill acquisition, duration of surgery can be affected by various factors in clinical practice including team efficiency and education. Standardised training for skill acquisition with appropriate measures of assessment in a controlled setting will aid in understanding the number of procedures required to achieve clinical competency in each subspeciality. global evaluative assessment of robotic skills (GEARS), and structured assessment of robotic microsurgery skills (SARMS), have been used as objective quantitative assessment tools in this field.
Training should also encompass theatre staff, as set up time including robot docking, change of arms, and equipment troubleshooting can be optimised to reduce burden and improve patient care [ 133 ]. Prolonged operative duration incurs significant resource utilisation including time, cost, equipment, and staff. Barbon et al. was able to demonstrate a steep learning curve in microvascular anastomosis to achieve an anastomotic time which was comparable with conventional approaches [ 89 ].
Vierstraete et al. describe the current training pathway of abdominal wall reconstruction and ventral hernia repair and found in their experience of posterior component separation that there was a gradual reduction in operative time until the surgical team reached their ‘comfort zone’ at around 20–25 cases. Depending on the frequency with which this procedure is performed, it may take a long period of time for the surgeon to reach that level of experience [ 189 ].
Other limitations
This report shows technical feasibility of robotic surgery; however, many articles are a relatively low level of evidence, with a high prevalence of case reports and case series. This review presents small sample sizes and as such, statistical analysis is likely to be underpowered, impeding ability to present true statistical significance. Whilst this study can suggest non-inferiority of robotic surgery, patient advantages remain to be clearly demonstrated.
There is a lack reported of long-term outcomes and formal PROMs, with variable follow-up duration. Due to large heterogeneity of the data and variance within patient selection, and outcomes reported, particularly within transoral robotic surgery, we have been unable to perform a weighted analysis for most subcategories, which would provide a more powerful comparison.
Conclusions
This literature review demonstrates technical feasibility of robotics in plastic and reconstructive surgery. High cosmetic satisfaction is reported with minimally invasive approaches. Operative time is higher than conventional approaches, although steep learning curves are reported, and this may contribute to a higher initial cost. Overall cost may be offset with improved patient outcomes within TOR and abdominal wall reconstruction; however, further reporting of cost and cost-effectiveness is necessary. Technical advantages can potentially translate to improvements in complication rate, and a faster recovery time, with non-inferior patient outcomes reported, with thoughtful case selection. However clearer evidence to support improved outcomes within the field, particularly in comparison with laparoscopic surgery, is required to justify the financial incurrence and demand on resources. Robotic surgery could play an exciting role within plastic surgery, and future research should focus on robotic training, as well as producing higher quality comparative clinical research, which is adequately powered, to fully understand the true benefit for patient care.
Data availability
No datasets were generated or analysed during the current study.
Kawka M, Fong Y, Gall TMH. Laparoscopic versus robotic abdominal and pelvic surgery: a systematic review of randomised controlled trials. Surg Endosc [Internet]. 2023 Sep 1 [cited 2024 Apr 6];37(9):6672. Available from: https://pubmed.ncbi.nlm.nih.gov/37442833/ . Accessed 14 Mar 2024
Moore LJ, Wilson MR, McGrath JS, Waine E, Masters RSW, Vine SJ (2015) Surgeons’ display reduced mental effort and workload while performing robotically assisted surgical tasks, when compared to conventional laparoscopy. Surg Endosc 29(9):2553–2560
Article PubMed Google Scholar
Struk S, Qassemyar Q, Leymarie N, Honart J-F, Alkhashnam H, De Fremicourt K et al (2018) The ongoing emergence of robotics in plastic and reconstructive surgery. Annales de Chirurgie Plastique Esthétique 63(2):105–112
Article CAS Google Scholar
Dobbs TD, Cundy O, Samarendra H, Khan K, Whitaker IS (2017) A systematic review of the role of robotics in plastic and reconstructive surgery—from inception to the future. Front Surg 15:4
Google Scholar
Jimenez C, Stanton E, Sung C, Wong AK (2022) Does plastic surgery need a rewiring? A survey and systematic review on robotic-assisted surgery. JPRAS Open 33:76–91
Article PubMed PubMed Central Google Scholar
Page MJ, McKenzie JE, Bossuyt PM, Boutron I, Hoffmann TC, Mulrow CD et al (2021) The PRISMA 2020 statement: an updated guideline for reporting systematic reviews. The BMJ 29:372
Microsoft Corporation (2018) Microsoft Excel [Internet]. Available from: https://office.microsoft.com/excel . Accessed 14 Mar 2024
Sterne JAC, Savović J, Page MJ, Elbers RG, Blencowe NS, Boutron I et al (2019) RoB 2: a revised tool for assessing risk of bias in randomised trials. BMJ 28:l4898
Article Google Scholar
Sterne JA, Hernán MA, Reeves BC, Savović J, Berkman ND, Viswanathan M, et al (2016) ROBINS-I: a tool for assessing risk of bias in non-randomized. BMJ 355:i4919. https://doi.org/10.1136/bmj.i4919
Munn Z, Barker T, Moola S, Tufanaru C, Stern C, McArthur A et al (2020) Methodological quality of case series studies: an introduction to the JBI critical appraisal tool. JBI Evidence Synthesis 18(10):2127–2133
PubMed Google Scholar
Moola S, Munn Z, Tufanaru C, Aromataris E, Sears K, Sfetcu R, Currie M, Qureshi R, Mattis P, Lisy K, Mu P-F (2017) Systematic reviews of etiology and risk. In: Aromataris E, Munn Z (eds) Joanna Briggs Institute reviewer's manual. The Joanna Briggs Institute, 2017. Available from https://reviewersmanual.joannabriggs.org/
Review Manager (RevMan) [Computer program]. Version 7.2.0. The Cochrane Collaboration, 2024. Available at revman.cochrane.org
Lee J, Park HS, Lee H, Lee K, Han DH, Lee DW (2020) Axillary lymph node dissection using a robotic surgical system: initial experience. J Surg Oncol 122(6):1252–1256
Feng AL, Razavi CR, Lakshminarayanan P, Ashai Z, Olds K, Balicki M et al (2017) The robotic ENT microsurgery system: a novel robotic platform for microvascular surgery. Laryngoscope 127(11):2495–2500
van Mulken TJM, Schols RM, Qiu SS, Brouwers K, Hoekstra LT, Booi DI et al (2018) Robotic (super) microsurgery: feasibility of a new master-slave platform in an in vivo animal model and future directions. J Surg Oncol 118(5):826–831
Malzone G, Menichini G, Innocenti M, Ballestín A (2023) Microsurgical robotic system enables the performance of microvascular anastomoses: a randomized in vivo preclinical trial. Sci Rep 13(1):14003
Article CAS PubMed PubMed Central Google Scholar
Ballestin A, Malzone G, Menichini G, Lucattelli E, Innocenti M, Ballestín A, et al (2022) New Robotic System with Wristed Microinstruments Allows Precise Reconstructive Microsurgery: Preclinical Study. Ann Surg Oncol 29(12):7859–7867
Zhu J-H, Deng J, Liu X-J, Wang J, Guo Y-X, Guo C-B (2016) Prospects of robot-assisted mandibular reconstruction with fibula flap: comparison with a computer-assisted navigation system and freehand technique. J Reconstr Microsurg 32(9):661–669
Manrique OJ, Bustos SS, Mohan AT, Nguyen M-D, Martinez-Jorge J, Forte AJ et al (2020) Robotic-assisted DIEP flap harvest for autologous breast reconstruction: a comparative feasibility study on a cadaveric model. J Reconstr Microsurg 36(05):362–368
Sánchez A, Rodríguez O, Jara G, Sánchez R, Vegas L, Rosciano J et al (2018) Robot-assisted surgery and incisional hernia: a comparative study of ergonomics in a training model. J Robot Surg 12(3):523–527
Chen MM, Orosco RK, Lim GC, Holsinger FC (2018) Improved transoral dissection of the tongue base with a next-generation robotic surgical system. Laryngoscope 128(1):78–83
Tay G, Tan H, Nguyen TK, Phee SJ, Iyer NG (2018) Use of the EndoMaster robot-assisted surgical system in transoral robotic surgery: A cadaveric study. Int J Med Robot Comput Assist Surg 14(4):e1930
Friedrich DT, Dürselen L, Mayer B, Hacker S, Schall F, Hahn J et al (2018) Features of haptic and tactile feedback in TORS-a comparison of available surgical systems. J Robot Surg 12(1):103–108
Leijte E, de Blaauw I, Rosman C, Botden SMBI (2020) Assessment of validity evidence for the RobotiX robot assisted surgery simulator on advanced suturing tasks. BMC Surg 20(1):183
De Groote R, Puliatti S, Amato M, Mazzone E, Rosiello G, Farinha R, et al (2022) Proficiency-based progression training for robotic surgery skills training: a randomized clinical trial. Larcher A Decoene J, Tuyten T, D’Hondt M, Hubert N, Chatzopoulos C, De Troyer B UP, Group JE working group on robot-assisted surgery of the EA of U, the ERUS Education Working, editors. BJU Int 30(4):528–535
Liverneaux PA, Hendriks S, Selber JC, Parekattil SJ (2013) Robotically assisted microsurgery: development of basic skills course. Arch Plast Surg 40(04):320–326
Perez M, Perrenot C, Tran N, Hossu G, Felblinger J, Hubert J (2013) Prior experience in micro-surgery may improve the surgeon’s performance in robotic surgical training. Int J Med Robot Comput Assist Surg 9(3):351–358
Alrasheed T, Liu J, Hanasono MM, Butler CE, Selber JC (2014) Robotic Microsurgery: validating an assessment tool and plotting the learning curve. Plast Reconstr Surg 134(4):794–803
Article CAS PubMed Google Scholar
Selber J, Alrasheed T (2014) Robotic microsurgical training and evaluation. Semin Plast Surg 28(01):005–010
Willems JIP, Shin AM, Shin DM, Bishop AT, Shin AY (2016) A comparison of robotically assisted microsurgery versus manual microsurgery in challenging situations. Plast Reconstr Surg 137(4):1317–1324
Clarke NS, Price J, Boyd T, Salizzoni S, Zehr KJ, Nieponice A et al (2018) Robotic-assisted microvascular surgery: skill acquisition in a rat model. J Robot Surg 12(2):331–336
van Mulken TJM, Boymans CAEM, Schols RM, Cau R, Schoenmakers FBF, Hoekstra LT et al (2018) Preclinical experience using a new robotic system created for microsurgery. Plast Reconstr Surg 142(5):1367–1376
Yang M, Peng J, Wang X, Lei H, Li X, Yang K (2022) Reinforcing the effect of microsurgery practice during robotic suturing skill acquisition. Int J Medi Robot Comput Assist Surg. https://doi.org/10.1002/rcs.2350
Beier JP, Hackenberg S, Boos AM, Modabber A, Duong Dinh TA, Hölzle F (2023) First series of free flap reconstruction using a dedicated robotic system in a multidisciplinary microsurgical center. Plast Reconstr Surg Glob Open 11(9):e5240
Louis V, Chih-Sheng L, Chevallier D, Selber JC, Xavier F, Liverneaux PA (2018) A porcine model for robotic training harvest of the rectus abdominis muscle. Annales de Chirurgie Plastique Esthétique 63(2):113–116
Thomaier L, Orlando M, Abernethy M, Paka C, Chen CCG (2017) Laparoscopic and robotic skills are transferable in a simulation setting: a randomized controlled trial. Surg Endosc 31(8):3279–3285
Orlando MS, Thomaier L, Abernethy MG, Chen CCG (2017) Retention of laparoscopic and robotic skills among medical students: a randomized controlled trial. Surg Endosc 31(8):3306–3312
Jacob MO, Karatassas A, Hewett P, Guirgis M, Hensman C, Catterwell R (2023) The use of a porcine model to teach advanced abdominal wall dissection techniques. Surg Endosc 37(12):9684–9689
Lee J, Park HS, Lee DW, Song SY, Yu J, Ryu JM et al (2021) From cadaveric and animal studies to the clinical reality of robotic mastectomy: a feasibility report of training program. Sci Rep 11(1):21032
Bur AM, Gomez ED, Newman JG, Weinstein GS, O’Malley BW, Rassekh CH et al (2017) Evaluation of high-fidelity simulation as a training tool in transoral robotic surgery. Laryngoscope 127(12):2790–2795
Zhang N, Sumer BD (2013) Transoral robotic surgery: simulation-based standardized training. JAMA Otolaryngol Head Neck Surg 139(11):1111
Kim WS, Byeon HK, Park YM, Ha JG, Kim ES, Koh YW et al (2015) Therapeutic robot-assisted neck dissection via a retroauricular or modified facelift approach in head and neck cancer: a comparative study with conventional transcervical neck dissection. Head Neck 37(2):249–254
Tae K, Ji YB, Song CM, Jeong JH, Cho SH, Lee SH (2014) Robotic selective neck dissection by a postauricular facelift approach: comparison with conventional neck dissection. Otolaryngol Head Neck Surg 150(3):394–400
Kim WS, Jittreetat T, Nam W, Sannikorn P, Choi EC, Koh YW (2015) Reconstruction of the segmental mandibular defect using a retroauricular or modified face-lift incision with an intraoral approach in head and neck cancer. Acta Otolaryngol 135(5):500–506
Du J, Mo H, Fan L, Jiang J (2017) Robot-assisted internal mammary lymph chain excision for breast cancer: a case report. Medicine 96(35):e7894–e7894
Lira RB, Chulam TC, de Carvalho GB, Schreuder WH, Koh YW, Choi EC et al (2018) Retroauricular endoscopic and robotic versus conventional neck dissection for oral cancer. J Robot Surg 12(1):117–129
Melly L, Jansens J-L, Kalscheuer G, Belhaj A, Rondelet B (2018) Robotic lymphadenectomy of an internal mammary lymph node metastasis. Acta Chir Belg 118(5):320–321
Qingqing H, Jian Z, Dayong Z, Ziyi F, Luming Z, Peng Z et al (2018) Robot-assisted internal mammary lymph node chain dissection for breast cancer. Clin Breast Cancer 18(4):e441–e445
Singh A, Jaipuria J, Goel A, Shah S, Bhardwaj R, Baidya S et al (2018) Comparing outcomes of robotic and open inguinal lymph node dissection in patients with carcinoma of the penis. J Urol 199(6):1518–1525
Paek SH, Lee HA, Kwon H, Kang KH, Park SJ (2020) Comparison of robot-assisted modified radical neck dissection using a bilateral axillary breast approach with a conventional open procedure after propensity score matching. Surg Endosc 34(2):622–627
Song R-Y, Sohn HJ, Paek SH, Kang KH (2020) The first report of robotic bilateral modified radical neck dissection through the bilateral axillo-breast approach for papillary thyroid carcinoma with bilateral lateral neck metastasis. Surg Laparosc Endosc Percutan Tech 30(3):e18-22
Pedersen J, Song DH, Selber JC (2014) Robotic, intraperitoneal harvest of the rectus abdominis muscle. Plast Reconstr Surg 134(5):1057–1063
Clemens M, Kronowitz S, Selber J (2014) Robotic-assisted latissimus dorsi harvest in delayed-immediate breast reconstruction. Semin Plast Surg 28(01):020–025
Chung J-H, You H-J, Kim H-S, Lee B-I, Park S-H, Yoon E-S (2015) A novel technique for robot assisted latissimus dorsi flap harvest. J Plast Reconstr Aesthet Surg 68(7):966–972
Lai H-W, Chen S-T, Lin S-L, Lin Y-L, Wu H-K, Pai S-H et al (2018) Technique for single axillary incision robotic assisted quadrantectomy and immediate partial breast reconstruction with robotic latissimus dorsi flap harvest for breast cancer: a case report. Medicine 97(27):e11373–e11373
Houvenaeghel G, Bannier M, Rua S, Barrou J, Heinemann M, Knight S et al (2019) Robotic breast and reconstructive surgery: 100 procedures in 2-years for 80 patients. Surg Oncol 31:38–45
Ozkan OO, Ozkan OO, Cinpolat A, Arici C, Bektas G, Can Ubur M et al (2019) Robotic harvesting of the omental flap: a case report and mini-review of the use of robots in reconstructive surgery. J Robot Surg 13(4):539–443
Houvenaeghel G, El Hajj H, Schmitt A, Cohen M, Rua S et al (2020) Robotic-assisted skin sparing mastectomy and immediate reconstruction using latissimus dorsi flap a new effective and safe technique: a comparative study. Surg Oncol 35:406–411
Fouarge A, Cuylits N (2020) From open to robotic-assisted latissimus dorsi muscle flap harvest. Plast Reconstr Surg Glob Open 8(1):e2569
Frey JD, Yu JW, Cohen SM, Zhao LC, Choi M, Levine JP (2020) Robotically assisted omentum flap harvest: a novel, minimally invasive approach for vascularized lymph node transfer. Plast Reconstr Surg Glob Open 8(4):e2505
Haverland R, Rebecca AM, Hammond J, Yi J (2021) A case series of robot-assisted rectus abdominis flap harvest for pelvic reconstruction: a single institution experience. J Minim Invasive Gynecol 28(2):245–248
Moon KC, Yeo HD, Yoon ES, Lee BI, Park SH, Chung JH et al (2020) Robotic-assisted latissimus dorsi muscle flap for autologous chest reconstruction in Poland syndrome. J Plast Reconstr Aesthet Surg 73(8):1506–1513
Winocour S, Tarassoli S, Chu CK, Liu J, Clemens MW, Selber JC (2020) Comparing outcomes of robotically assisted latissimus dorsi harvest to the traditional open approach in breast reconstruction. Plast Reconstr Surg 146(6):1221–1225
Asaad M, Pisters LL, Klein GT, Adelman DM, Oates SD, Butler CE et al (2021) Robotic rectus abdominis muscle flap following robotic extirpative surgery. Plast Reconstr Surg 148(6):1377–1381
Day SJ, Dy B, Nguyen M-D (2021) Robotic omental flap harvest for near-total anterior chest wall coverage: a potential application of robotic techniques in plastic and reconstructive surgery. BMJ Case Rep 14(2):e237887
Joo OY, Song SY, Lew DH, Park HS, Lee DW (2021) Robotic harvest of a latissimus dorsi flap using a single-port surgical robotic system in breast reconstruction. Arch Plast Surg 48(06):577–582
Cheon JH, Kim HE, Park SH, Yoon ES (2022) Ten-year experience of robotic latissimus muscle flap reconstructive surgery at a single institution. J Plast Reconstr Aesthet Surg 75(10):3664–3672
Davila AA, Goldman J, Kleban S, Lyons M, Brosious J, Bardakcioglu O et al (2022) Reducing complications and expanding use of robotic rectus abdominis muscle harvest for pelvic reconstruction. Plast Reconstr Surg 150(1):190–195
Hwang Y-J, Chung J-H, Lee H-C, Park S-H, Yoon E-S (2022) Single-port transaxillary robot-assisted latissimus dorsi muscle flap reconstruction for poland syndrome: concomitant application of robotic system to contralateral augmentation mammoplasty. Arch Plast Surg 49(03):373–377
Eo PS, Kim H, Lee JS, Lee J, Park HY, Yang JD (2023) Robot-assisted latissimus dorsi flap harvest for partial breast reconstruction: comparison with endoscopic and conventional approaches. Aesthet Surg J 44(1):38–46
Shin S-W, Kim H, Nam W, Kim HJ, Cha I-H, Koh YW et al (2023) Robot-assisted radial forearm free flap harvesting: a propensity score-matched case–control study. J Robot Surg 17(4):1429–1434
Lai H-W, Lin S-L, Chen S-T, Lin Y-L, Chen D-R, Pai S-S et al (2018) Robotic nipple sparing mastectomy and immediate breast reconstruction with robotic latissimus dorsi flap harvest—technique and preliminary results. J Plast Reconstr Aesthet Surg 71(10):e59-61
Gundlapalli VS, Ogunleye AA, Scott K, Wenzinger E, Ulm JP et al (2018) Robotic-assisted deep inferior epigastric artery perforator flap abdominal harvest for breast reconstruction: a case report. Microsurgery 38(6):702–705
Choi JH, Song SY, Park HS, Kim CH, Kim JY, Lew DH et al (2021) Robotic DIEP flap harvest through a totally extraperitoneal approach using a single-port surgical robotic system. Plast Reconstr Surg 148(2):304–307
Bishop SN, Asaad M, Liu J, Chu CK, Clemens MW, Kapur SS et al (2022) Robotic harvest of the deep inferior epigastric perforator flap for breast reconstruction: a case series. Plast Reconstr Surg 149(5):1073–1077
Daar DA, Anzai LM, Vranis NM, Schulster ML, Frey JD, Jun M et al (2022) Robotic deep inferior epigastric perforator flap harvest in breast reconstruction. Microsurgery 42(4):319–325
Dayaratna N, Ahmadi N, Mak C, Dusseldorp JR (2023) Robotic-assisted deep inferior epigastric perforator (DIEP) flap harvest for breast reconstruction. ANZ J Surg 93(4):1072–1074
Wittesaele W, Vandevoort M (2022) Implementing the Robotic deep inferior epigastric perforator Flap in daily practice: a series of 10 cases. J Plast Reconstr Aesthet Surg 75(8):2577–2583
Tsai C-Y, Kim B-S, Kuo W-L, Liu K-H, Chang TN-J, Cheong DC-F et al (2023) Novel port placement in robot-assisted DIEP flap harvest improves visibility and bilateral DIEP access: early controlled cohort study. Plast Reconstr Surg 152(4):590–595
Zanaty M, Atluri S, Taylor B, Choutka O (2023) Novel use of robotically harvested internal thoracic artery in high-flow cerebral bypass. World Neurosurg 178:52
Hans S, Jouffroy T, Veivers D, Hoffman C, Girod A, Badoual C et al (2013) Transoral robotic-assisted free flap reconstruction after radiation therapy in hypopharyngeal carcinoma: report of two cases. Eur Arch Otorhinolaryngol 270(8):2359–2364
Song HG, Yun IS, Lee WJ, Lew DH, Rah DK (2013) Robot-assisted free flap in head and neck reconstruction. Arch Plast Surg 40(04):353–358
Lai C-S, Chen I-C, Liu S-A, Lu C-T, Yen J-H, Song D-Y (2015) Robot-assisted free flap reconstruction of oropharyngeal cancer–a preliminary report. Ann Plast Surg 74:S105–S108
Miyamoto H, Leechavengvongs S, Atik T, Facca S, Liverneaux P (2014) Nerve transfer to the deltoid muscle using the nerve to the long head of the triceps with the da Vinci robot: six cases. J Reconstr Microsurg 30(06):375–380
Tsai Y-C, Liu S-A, Lai C-S, Chen Y-W, Lu C-T, Yen J-H et al (2017) Functional outcomes and complications of robot-assisted free flap oropharyngeal reconstruction. Ann Plast Surg 78(3):S76-82
Lai C, Lu C, Liu S, Tsai Y, Chen Y, Chen I (2019) Robot-assisted microvascular anastomosis in head and neck free flap reconstruction: preliminary experiences and results. Microsurgery 39(8):715–720
van Mulken TJM, Schols RM, Scharmga AMJ, Winkens B, Cau R, Schoenmakers FBF et al (2020) First-in-human robotic supermicrosurgery using a dedicated microsurgical robot for treating breast cancer-related lymphedema: a randomized pilot trial. Nat Commun 11(1):757
Chang TN, Daniel BW, Hsu AT, Chen LW, Sung CW, Chuang DC et al (2021) Reversal of thoracic sympathectomy through robot-assisted microsurgical sympathetic trunk reconstruction with sural nerve graft and additional end-to-side coaptation of the intercostal nerves: a case report. Microsurgery 41(8):772–776
Barbon C, Grünherz L, Uyulmaz S, Giovanoli P, Lindenblatt N (2022) Exploring the learning curve of a new robotic microsurgical system for microsurgery. JPRAS Open 34:126–133
Lindenblatt N, Grünherz L, Wang A, Gousopoulos E, Barbon C, Uyulmaz S et al (2022) Early experience using a new robotic microsurgical system for lymphatic surgery. Plast Reconstr Surg Glob Open 10(1):e4013
van Mulken TJM, Wolfs JAGN, Qiu SS, Scharmga AMJ, Schols RM, van Weezelenburg MAS et al (2022) One-year outcomes of the first human trial on robot-assisted lymphaticovenous anastomosis for breast cancer-related lymphedema. Plast Reconstr Surg 149(1):151–161
Besmens IS, Politikou O, Giovanoli P, Calcagni M, Lindenblatt N (2024) Robotic microsurgery in extremity reconstruction - experience with a novel robotic system. Surg Innov 31(1):42–47
Chen LW-Y, Chang TN-J, Lee C-P, Sung CW-H, Cheng C, Chang K-H et al (2023) Robotic sympathetic trunk reconstruction for compensatory sweating after thoracic sympathectomy. JTCVS Tech 21:251–258
Innocenti M, Malzone G, Menichini G (2023) First-in-human free flap tissue reconstruction using a dedicated microsurgical robotic platform. Plast Reconstr Surg 151(5):1078–1082
Weinzierl A, Barbon C, Gousopoulos E, von Reibnitz D, Giovanoli P, Grünherz L et al (2023) Benefits of robotic-assisted lymphatic microsurgery in deep anatomical planes. JPRAS Open 37:145–154
Lin L, Sun M, Xu C, Gao Y, Xu H, Yang X et al (2022) Assessment of robot-assisted mandibular contouring surgery in comparison with traditional surgery: a prospective, single-center. Randomized Controlled Trial Aesthet Surg J 42(6):567–579
Lin L, Zhao Z, Han W, Sun M, Zhang Z, Kim BS et al (2023) Advances in robot-assisted surgery for facial bone contouring surgery. J Craniofac Surg 34(2):813–816
Téblick S, Ruymaekers M, de Casteele E, Boudewyns A, Nadjmi N, Teblick S et al (2023) The effect of soft palate reconstruction with the da Vinci robot on middle ear function in children: an observational study. Int J Oral Maxillofac Surg 52(9):931–938
Bruyere A, Hidalgo Diaz JJ, Vernet P, Salazar Botero S, Facca S, Liverneaux P-A (2016) Technical feasibility of robot-assisted minimally-invasive neurolysis of the lateral cutaneous nerve of thigh: about a case. Ann Chir Plast Esthet 61(6):872–876
Boztosun A, Olgan S (2016) Robotic sigmoid vaginoplasty in an adolescent girl with mayer-rokitansky-kuster-hauser syndrome. Female Pelvic Med Reconstr Surg 22(5):e32–e35
Dy GW, Jun MS, Blasdel G, Bluebond-Langner R, Zhao LC (2021) Outcomes of gender affirming peritoneal flap vaginoplasty using the da vinci single port versus xi robotic systems. Eur Urol 79(5):676–683
Blasdel G, Kloer C, Parker A, Shakir N, Zhao LC, Bluebond-Langner R (2023) Genital hypoplasia before gender-affirming vaginoplasty: does the robotic peritoneal flap method create equivalent vaginal canal outcomes? Plast Reconstr Surg 151(4):867–874
Chen YJ, Huynh D, Nguyen S, Chin E, Divino C, Zhang L (2017) Outcomes of robot-assisted versus laparoscopic repair of small-sized ventral hernias. Surg Endosc 31(3):1275–1279
Bittner JG 4th, Alrefai S, Vy M, Mabe M, Del Prado PAR, Clingempeel NL (2018) Comparative analysis of open and robotic transversus abdominis release for ventral hernia repair. Surg Endosc 32(2):727–734
Gonzalez A, Escobar E, Romero R, Walker G, Mejias J, Gallas M et al (2017) Robotic-assisted ventral hernia repair: a multicenter evaluation of clinical outcomes. Surg Endosc 31(3):1342–1349
Jamshidian M, Stanek S, Sferra J, Jamil T (2018) Robotic repair of symptomatic Spigelian hernias: a series of three cases and surgical technique review. J Robot Surg 12(3):557–560
Prabhu AS, Dickens EO, Copper CM, Mann JW, Yunis JP, Phillips S et al (2017) Laparoscopic vs robotic intraperitoneal mesh repair for incisional hernia: an Americas Hernia Society Quality Collaborative Analysis. J Am Coll Surg 225(2):285–293
Wang SC, Singh TP (2017) Robotic repair of a large abdominal intercostal hernia: a case report and review of literature. J Robot Surg 11(2):271–274
Warren JA, Cobb WS, Ewing JA, Carbonell AM (2017) Standard laparoscopic versus robotic retromuscular ventral hernia repair. Surg Endosc 31(1):324–332
Carbonell AM, Warren JA, Prabhu AS, Ballecer CD, Janczyk R et al (2018) Reducing length of stay using a robotic-assisted approach for retromuscular ventral hernia repair: a comparative analysis from the americas hernia society quality collaborative. Ann Surg 267(2):210–217
Martin-Del-Campo LA, Weltz AS, Belyansky I, Novitsky YW (2018) Comparative analysis of perioperative outcomes of robotic versus open transversus abdominis release. Surg Endosc 32(2):840–845
Muysoms F, Van Cleven S, Pletinckx P, Ballecer C, Ramaswamy A (2018) Robotic transabdominal retromuscular umbilical prosthetic hernia repair (TARUP): observational study on the operative time during the learning curve. Hernia 22(6):1101–1111
Walker PA, May AC, Cherla DV, Santillan MR et al (2018) Multicenter review of robotic versus laparoscopic ventral hernia repair: is there a role for robotics. Surg Endosc 32(4):1901–1905
Kudsi OY, Gokcal F (2019) Robotic approach to modified Sugarbaker parastomal hernia repair by performing transversus abdominis release—a video vignette. Colorectal Dis 21(7):854–855
Kudsi OY, Chang K, Bou-Ayash N, Gokcal F (2020) Transabdominal (TA) versus totally extraperitoneal (TEP) robotic retromuscular ventral hernia repair: a propensity score matching analysis. Surg Endosc 34(8):3550–3559
Olavarria OA, Bernardi K, Shah SK, Wilson TD, Wei S, Pedroza C et al (2020) Robotic versus laparoscopic ventral hernia repair: multicenter, blinded randomized controlled trial. BMJ 370:m2457–m2457
Mudyanadzo TA, Hunter JD 3rd, Rider PF, Richards WO (2020) An evaluation of robotic ventral hernia repair. Am Surg 86(1):e45–e46
Petro CC, Zolin S, Krpata D, Alkhatib H, Tu C, Rosen MJ et al (2021) Patient-reported outcomes of robotic vs laparoscopic ventral hernia repair with intraperitoneal mesh: the PROVE-IT randomized clinical trial. JAMA Surg 156(1):22–29
Bergholz D, Obi JR, Grossman R, Rasul TF (2021) Robotic repair of an acquired abdominal intercostal hernia. CRSLS : MIS case reports from SLS. 8(4):e2021.00061
Dhanani NH, Olavarria OA, Holihan JL, Shah SK, Wilson TD, Loor MM et al (2021) Robotic versus laparoscopic ventral hernia repair: one-year results from a prospective, multicenter. Blinded Randomized Controlled Trial Ann Surg 273(6):1076–1080
Rayman S, Yuori M, Jacob R, Ephraim K, Mohammad A, Lior S et al (2021) Transabdominal Preperitoneal (TAPP) for the Treatment of Spigelian hernias. JSLS 25(2):e2021.00024
Costa TN, Abdalla RZ, Tustumi F, Junior UR, Cecconello I (2023) Robotic-assisted compared with laparoscopic incisional hernia repair following oncologic surgery: short- and long-term outcomes of a randomized controlled trial. J Robot Surg 17(1):99–107
Kakela P, Mustonen K, Rantanen T, Paajanen H, Käkelä P, Mustonen K et al (2023) Robotic versus hybrid assisted ventral hernia repair: a prospective one-year comparative study of clinical outcomes. Acta Chir Belg 123(4):411–417
Kudsi OY, Gokcal F, Bou-Ayash N, Watters E, Pereira X, Lima DL et al (2022) A comparison of outcomes between class-II and class-III obese patients undergoing robotic ventral hernia repair: a multicenter study. Hernia 26(6):1531–1539
Petro CC, Thomas JD, Tu C, Krpata DM, Beffa LR, Rosen MJ et al (2022) Robotic vs laparoscopic ventral hernia repair with intraperitoneal mesh: 1-year exploratory outcomes of the PROVE-IT randomized clinical trial. J Am Coll Surg 234(6):1160–1165
Pereira X, Lima DL, Huang L-C, Salas-Parra R, Shah P, Malcher F et al (2023) Robotic versus open lateral abdominal hernia repair: a multicenter propensity score matched analysis of perioperative and 1-year outcomes. Hernia 27(2):293–304
Shimada G, Matsubara T, Sanbonmatsu M, Nakabayashi R, Miyachi Y, Taketa T et al (2023) The first case of robotic-assisted transabdominal retrorectus repair for incisional hernia in Japan. Asian J Endosc Surg 16(2):305–311
Dhanani NH, Lyons NB, Olavarria OA, Bernardi K, Holihan JL, Shah SK et al (2023) Robotic versus laparoscopic ventral hernia repair: two-year results from a prospective, multicenter. Blinded Randomized Clinical Trial Ann Surg 278(2):161–165
Lima DL, Alcabes A, Viscarret V, Nogueira R, Malcher F (2023) Incarcerated epiploic appendix in a spigelian hernia treated by robotic-assisted surgery. CRSLS MIS Case Rep SLS 10(2):e2023.00008
Petro CC, Maskal SM, Renton DB, Yunis JP, Meara MP, Diaz K et al (2023) Robotic enhanced-view totally extraperitoneal vs intraperitoneal onlay mesh evaluation: 1-year exploratory outcomes of the REVEAL randomized clinical trial. J Am Coll Surg 237(4):614–620
Sarfati B, Honart J-F, Leymarie N, Rimareix F, Al Khashnam H, Kolb F (2017) Robotic da Vinci Xi-assisted nipple-sparing mastectomy: first clinical report. Breast J 24(3):373–376
Toesca A, Peradze N, Galimberti V, Manconi A, Intra M, Gentilini O et al (2017) Robotic nipple-sparing mastectomy and immediate breast reconstruction with implant: first report of surgical technique. Ann Surg 266(2):e28-30
Toesca A, Peradze N, Manconi A, Galimberti V, Intra M, Colleoni M et al (2017) Robotic nipple-sparing mastectomy for the treatment of breast cancer: feasibility and safety study. The Breast 31:51–56
Lai H-W, Lin S-L, Chen S-T, Chen S-L, Lin Y-L, Chen D-R et al (2018) Robotic nipple-sparing mastectomy and immediate breast reconstruction with gel implant. Plast Reconstr Surg Glob Open 6(6):e1828
Park HS, Kim JH, Lee DW, Song SY, Park S, Kim Il S et al (2018) Gasless Robot-Assisted Nipple-Sparing Mastectomy: A Case Report. J Breast Cancer 21(3):334
Rajappa SK, Kumar R, Garg S, Ram D (2018) Robotic nipple-sparing mastectomy: the first experience from Indian subcontinent. Breast J 24(6):1114–1115
Sarfati B, Struk S, Leymarie N, Honart J-F, Alkhashnam H, Kolb F et al (2018) Robotic nipple-sparing mastectomy with immediate prosthetic breast reconstruction: surgical technique. Plast Reconstr Surg 142(3):624–627
Sarfati B, Struk S, Leymarie N, Honart J-F, Alkhashnam H, Tran de Fremicourt K et al (2018) Robotic prophylactic nipple-sparing mastectomy with immediate prosthetic breast reconstruction: a prospective study. Ann Surg Oncol 25(9):2579–2586
Houvenaeghel G, Bannier M, Rua S, Barrou J, Heinemann M, Van Troy A et al (2019) Breast cancer robotic nipple sparing mastectomy: evaluation of several surgical procedures and learning curve. World J Surg Oncol 17(1):27
Kuo W-L, Huang J-J, Huang Y-T, Chueh L-F, Lee J-T, Tsai H-P et al (2020) Robot-assisted mastectomy followed by immediate autologous microsurgical free flap reconstruction: techniques and feasibility in three different breast cancer surgical scenarios. Clin Breast Cancer 20(1):e1-8
Lai H-W, Chen S-T, Lin S-L, Chen C-J, Lin Y-L, Pai S-H et al (2019) Robotic nipple-sparing mastectomy and immediate breast reconstruction with gel implant: technique, preliminary results and patient-reported cosmetic outcome. Ann Surg Oncol 26(1):42–52
Lai H-W, Wang C-C, Lai Y-C, Chen C-J, Lin S-L, Chen S-T et al (2019) The learning curve of robotic nipple sparing mastectomy for breast cancer: an analysis of consecutive 39 procedures with cumulative sum plot. Eur J Surg Oncol 45(2):125–133
Lai H-W, Chen S-T, Mok CW, Lin Y-J, Wu H-K, Lin S-L et al (2020) Robotic versus conventional nipple sparing mastectomy and immediate gel implant breast reconstruction in the management of breast cancer - a case control comparison study with analysis of clinical outcome, medical cost, and patient-reported cosmetic results. J Plast Reconstr Aesthet Surg 73(8):1514–1525
Toesca A, Sangalli C, Maisonneuve P, Massari G, Girardi A, Baker JL et al (2022) A randomized trial of robotic mastectomy versus open surgery in women with breast cancer or BrCA mutation. Ann Surg 276(1):11–19
Park HS, Lee J, Lai H-W, Park JM, Ryu JM, Lee JE et al (2022) Surgical and oncologic outcomes of robotic and conventional nipple-sparing mastectomy with immediate reconstruction: international multicenter pooled data analysis. Ann Surg Oncol 29(11):6646–6657
Moon J, Lee J, Lee DW, Lee HS, Nam DJ, Kim MJ et al (2021) Postoperative pain assessment of robotic nipple-sparing mastectomy with immediate prepectoral prosthesis breast reconstruction: a comparison with conventional nipple-sparing mastectomy. Int J Med Sci 18(11):2409–2416
Chan JYK, Tsang RK, Eisele DW, Richmon JD (2013) Transoral robotic surgery of the parapharyngeal space: a case series and systematic review. Head Neck 37(2):293–298
Chia SH, Gross ND, Richmon JD (2013) Surgeon experience and complications with Transoral Robotic Surgery (TORS). Otolaryngol Head Neck Surg 149(6):885–892
Durmus K, Rangarajan SV, Old MO, Agrawal A, Teknos TN, Ozer E (2013) Transoral robotic approach to carcinoma of unknown primary. Head Neck 36(6):848–852
Durmus K, Apuhan T, Ozer E (2013) Transoral robotic surgery for retromolar trigone tumours. Acta Otorhinolaryngol Ital 33(6):425–427
CAS PubMed PubMed Central Google Scholar
Lee SY, Park YM, Byeon HK, Choi EC, Kim S-H (2014) Comparison of oncologic and functional outcomes after transoral robotic lateral oropharyngectomy versus conventional surgery for T1 to T3 tonsillar cancer. Head Neck 36(8):1138–1145
Patel SA, Magnuson JS, Holsinger FC, Karni RJ, Richmon JD, Gross ND et al (2013) Robotic surgery for primary head and neck squamous cell carcinoma of unknown site. JAMA Otolaryngol Head Neck Surg 139(11):1203–1211
RK T, WK H, WI W, JY C (2013) Transoral robotic assisted nasopharyngectomy via a lateral palatal flap approach. Laryngoscope 123(9):2180–2183
White H, Ford S, Bush B, Holsinger FC, Moore E, Ghanem T et al (2013) Salvage surgery for recurrent cancers of the oropharynx: comparing TORS with standard open surgical approaches. JAMA Otolaryngol Head Neck Surg 139(8):773–778
Chung TK, Rosenthal EL, Magnuson JS, Carroll WR (2015) Transoral robotic surgery for oropharyngeal and tongue cancer in the United States. Laryngoscope 125(1):140–145
Durmus K, Patwa HS, Gokozan HN, Kucur C, Teknos TN, Agrawal A et al (2014) Functional and quality-of-life outcomes of transoral robotic surgery for carcinoma of unknown primary. Laryngoscope 124(9):2089–2095
Ford SE, Brandwein-Gensler M, Carroll WR, Rosenthal EL, Magnuson JS (2014) Transoral robotic versus open surgical approaches to oropharyngeal squamous cell carcinoma by human papillomavirus status. Otolaryngol Head Neck Surg 151(4):606–611
Hammoudi K, Pinlong E, Kim S, Bakhos D, Morinière S (2015) Transoral robotic surgery versus conventional surgery in treatment for squamous cell carcinoma of the upper aerodigestive tract. Head Neck 37(9):1304–1309
van Loon JWL, Smeele LE, Hilgers FJM, van den Brekel MWM (2015) Outcome of transoral robotic surgery for stage I-II oropharyngeal cancer. Eur Arch Otorhinolaryngol 272(1):175–183
de Almeida JR, Li R, Magnuson JS, Smith RV, Moore E, Lawson G et al (2015) Oncologic outcomes after transoral robotic surgery: a multi-institutional study. JAMA Otolaryngol Head Neck Surg 141(12):1043–1051
Dabas S, Dewan A, Ranjan R, Dewan AK, Puri A, Shah SH et al (2015) Transoral robotic surgery in management of oropharyngeal cancers: a preliminary experience at a tertiary cancer centre in India. Int J Clin Oncol 20(4):693–700
Mercante G, Masiello A, Sperduti I, Cristalli G, Pellini R et al (2015) Quality of life and functional evaluation in patients with tongue base tumors treated exclusively with transoral robotic surgery: a 1-year follow-up study. J Craniomaxillofac Surg 43(8):1561–1566
Mockelmann N, Busch C-J, Munscher A, Knecht R, Lorincz BB, Möckelmann N et al (2015) Timing of neck dissection in patients undergoing transoral robotic surgery for head and neck cancer. Eur J Surg Oncol 41(6):773–778
Razafindranaly V, Lallemant B, Aubry K, Moriniere S, Vergez S, De ME et al (2016) Clinical outcomes with transoral robotic surgery for supraglottic squamous cell carcinoma: experience of a French evaluation cooperative subgroup of GETTEC. Head Neck 38:E1097–E1101
Smith RV, Schiff BA, Garg M, Haigentz M (2015) The impact of transoral robotic surgery on the overall treatment of oropharyngeal cancer patients. Laryngoscope 125:S1-15
Aubry K, Vergez S, de Mones E, Moriniere S, Choussy O, Malard O et al (2016) Morbidity and mortality revue of the French group of transoral robotic surgery: a multicentric study. J Robot Surg 10(1):63–67
Fujiwara K, Fukuhara T, Kitano H, Fujii T, Koyama S, Yamasaki A et al (2016) Preliminary study of transoral robotic surgery for pharyngeal cancer in Japan. J Robot Surg 10(1):11–17
Granell J, Alonso A, Garrido L, Gutierrez-Fonseca R (2016) Transoral fully robotic dissection of a parapharyngeal hemangioma. J Craniofac Surg 27(7):1806–1807
Duek I, Amit M, Sviri GE, Gil Z (2017) Combined endoscopic transcervical-transoral robotic approach for resection of parapharyngeal space tumors. Head Neck 39(4):786–790
Frenkel CH, Yang J, Zhang M, Altieri MS, Telem DA, Samara GJ (2017) Compared outcomes of concurrent versus staged transoral robotic surgery with neck dissection. Otolaryngol Head Neck Surg 157(5):791–797
Gorphe P, Von Tan J, El Bedoui S, Hartl DM, Auperin A, Qassemyar Q et al (2017) Early assessment of feasibility and technical specificities of transoral robotic surgery using the da Vinci Xi. J Robot Surg 11(4):455–461
Lallemant B, Moriniere S, Ceruse P, Lebalch M, Aubry K, Hans S et al (2017) Transoral robotic surgery for squamous cell carcinomas of the posterior pharyngeal wall. Eur Arch Otorhinolaryngol 274(12):4211–4216
Mahmoud O, Sung K, Civantos FJ, Thomas GR, Samuels MA (2018) Transoral robotic surgery for oropharyngeal squamous cell carcinoma in the era of human papillomavirus. Head Neck 40(4):710–721
Rubek N, Channir HI, Charabi BW, Lajer CB, Kiss K, Nielsen HU et al (2017) Primary transoral robotic surgery with concurrent neck dissection for early stage oropharyngeal squamous cell carcinoma implemented at a Danish head and neck cancer center: a phase II trial on feasibility and tumour margin status. Eur Arch Otorhinolaryngol 274(5):2229–2237
Sethia R, Yumusakhuylu AC, Ozbay I, Diavolitsis V, Brown NV, Zhao S et al (2018) Quality of life outcomes of transoral robotic surgery with or without adjuvant therapy for oropharyngeal cancer. Laryngoscope 128(2):403–411
Alessandrini M, Pavone I, Micarelli A, Caporale C (2018) Transoral robotic surgery for the base of tongue squamous cell carcinoma: a preliminary comparison between da Vinci Xi and Si. J Robot Surg 12(3):417–423
Doazan M, Hans S, Morinière S, Lallemant B, Vergez S, Aubry K et al (2018) Oncologic outcomes with transoral robotic surgery for supraglottic squamous cell carcinoma: results of the French Robotic Surgery Group of GETTEC. Head Neck 40(9):2050–2059
Li H, Torabi SJ, Park HS, Yarbrough WG, Mehra S, Choi R et al (2019) Clinical value of transoral robotic surgery: nationwide results from the first 5 years of adoption. Laryngoscope 129(8):1844–1855
Scott-Wittenborn N, Jackson RS (2018) Intraoperative imaging during minimally invasive transoral robotic surgery using near-infrared light. Am J Otolaryngol 39(2):220–222
Hardy BM, LoSavio P, Al-Khudari S (2020) Transoral robotic resection of recurrent neopharyngeal squamous cell carcinoma. Ear Nose Throat J 99(5):303–304
Nichols AC, Theurer J, Prisman E, Read N, Berthelet E, Tran E et al (2019) Radiotherapy versus transoral robotic surgery and neck dissection for oropharyngeal squamous cell carcinoma (ORATOR): an open-label, phase 2, randomised trial. Lancet Oncol 20(10):1349–1359
Petruzzi G, Zocchi J, Moretto S, Pichi B, Cristalli G, Mercante G et al (2019) Transoral robotic retropharyngeal lymph node dissection in a recurrent head and neck carcinoma. Head Neck 41(11):4051–4053
Holcomb AJ, Richmon JD (2021) Transoral robotic salvage oropharyngectomy with submental artery island flap reconstruction. Head Neck 43(2):E13–E19
Kubik MW, Channir HI, Rubek N, Kim S, Ferris RL, von Buchwald C et al (2021) TORS base-of-tongue mucosectomy in human papilloma virus-negative carcinoma of unknown primary. Laryngoscope 131(1):78–81
Sano D, Shimizu A, Tateya I, Fujiwara K, Mori T, Miyamoto S et al (2021) Treatment outcomes of transoral robotic and non-robotic surgeries to treat oropharyngeal, hypopharyngeal, and supraglottic squamous cell carcinoma: a multi-center retrospective observational study in Japan. Auris Nasus Larynx 48(3):502–510
D’Andrea G, Bordenave L, Nguyen F, Tao Y, Paleri V, Temam SS et al (2022) A prospective longitudinal study of quality of life in robotic-assisted salvage surgery for oropharyngeal cancer. Eur J Surg Oncol 48(6):1243–1250
Nichols AC, Theurer J, Prisman E, Read N, Berthelet E, Tran E et al (2022) Randomized trial of radiotherapy versus transoral robotic surgery for oropharyngeal squamous cell carcinoma: long-term results of the ORATOR trial. J Clin Oncol 40(8):866–875
De Virgilio A, Pellini R, Cammaroto G, Sgarzani R, De Vito A et al (2023) Trans oral robotic surgery for oropharyngeal cancer: a multi institutional experience. Eur J Surg Oncol 49(9):106945
Vierstraete M, Simons M, Borch K, de Beaux A, East B, Reinpold W et al (2022) Description of the current da Vinci ® training pathway for robotic abdominal wall surgery by the European Hernia Society. J Abdom Wall Surg 30:1
Download references
There was no funding allocated for this research.
Author information
Authors and affiliations.
Charles Wolfson Centre of Reconstructive Surgery, University College London, Royal Free Hospital, London, UK
Laura Awad, Benedict Reed, Edward Bollen, Benjamin J. Langridge, Sara Jasionowska, Peter E. M. Butler & Allan Ponniah
Department of Plastic Surgery, Royal Free Hospital, London, UK
Laura Awad, Benedict Reed, Benjamin J. Langridge, Sara Jasionowska, Peter E. M. Butler & Allan Ponniah
Department of Surgery and Interventional Sciences, University College London, Royal Free Hospital, London, UK
Laura Awad, Benjamin J. Langridge & Peter E. M. Butler
You can also search for this author in PubMed Google Scholar
Contributions
Authors Contributions are detailed below Design: L.A, B.R, E.B, B.L, S.J, P.B, A.P Literature Search/Screening: L.A, B.R, E.B, B.L Data Collection : L.A, E.B, B.R Data Analysis: L.A, B.R Manuscript Writing : L.A, B.R, S.J, B.L, PB, AP Tables and Figures: S.J, B.L, B.R, LA Risk of Bias: E.B, L.A Editing : L.A, B.R, E.B, B.L, S.J, P.B, A.P All authors have reviewed the final submission.
Corresponding author
Correspondence to Laura Awad .
Ethics declarations
Conflict of interest.
There are no conflicts of interest to declare.
Additional information
Publisher's note.
Springer Nature remains neutral with regard to jurisdictional claims in published maps and institutional affiliations.
Supplementary Information
Below is the link to the electronic supplementary material.
Supplementary file1 (PNG 749 KB)
Supplementary file2 (png 2249 kb), supplementary file3 (pdf 26 kb), supplementary file4 (pdf 15 kb), rights and permissions.
Open Access This article is licensed under a Creative Commons Attribution 4.0 International License, which permits use, sharing, adaptation, distribution and reproduction in any medium or format, as long as you give appropriate credit to the original author(s) and the source, provide a link to the Creative Commons licence, and indicate if changes were made. The images or other third party material in this article are included in the article's Creative Commons licence, unless indicated otherwise in a credit line to the material. If material is not included in the article's Creative Commons licence and your intended use is not permitted by statutory regulation or exceeds the permitted use, you will need to obtain permission directly from the copyright holder. To view a copy of this licence, visit http://creativecommons.org/licenses/by/4.0/ .
Reprints and permissions
About this article
Awad, L., Reed, B., Bollen, E. et al. The emerging role of robotics in plastic and reconstructive surgery: a systematic review and meta-analysis. J Robotic Surg 18 , 254 (2024). https://doi.org/10.1007/s11701-024-01987-7
Download citation
Received : 19 April 2024
Accepted : 19 May 2024
Published : 15 June 2024
DOI : https://doi.org/10.1007/s11701-024-01987-7
Share this article
Anyone you share the following link with will be able to read this content:
Sorry, a shareable link is not currently available for this article.
Provided by the Springer Nature SharedIt content-sharing initiative
- Robotic assisted
- Plastic and reconstructive surgery
- Find a journal
- Publish with us
- Track your research
Information
- Author Services
Initiatives
You are accessing a machine-readable page. In order to be human-readable, please install an RSS reader.
All articles published by MDPI are made immediately available worldwide under an open access license. No special permission is required to reuse all or part of the article published by MDPI, including figures and tables. For articles published under an open access Creative Common CC BY license, any part of the article may be reused without permission provided that the original article is clearly cited. For more information, please refer to https://www.mdpi.com/openaccess .
Feature papers represent the most advanced research with significant potential for high impact in the field. A Feature Paper should be a substantial original Article that involves several techniques or approaches, provides an outlook for future research directions and describes possible research applications.
Feature papers are submitted upon individual invitation or recommendation by the scientific editors and must receive positive feedback from the reviewers.
Editor’s Choice articles are based on recommendations by the scientific editors of MDPI journals from around the world. Editors select a small number of articles recently published in the journal that they believe will be particularly interesting to readers, or important in the respective research area. The aim is to provide a snapshot of some of the most exciting work published in the various research areas of the journal.
Original Submission Date Received: .
- Active Journals
- Find a Journal
- Proceedings Series
- For Authors
- For Reviewers
- For Editors
- For Librarians
- For Publishers
- For Societies
- For Conference Organizers
- Open Access Policy
- Institutional Open Access Program
- Special Issues Guidelines
- Editorial Process
- Research and Publication Ethics
- Article Processing Charges
- Testimonials
- Preprints.org
- SciProfiles
- Encyclopedia
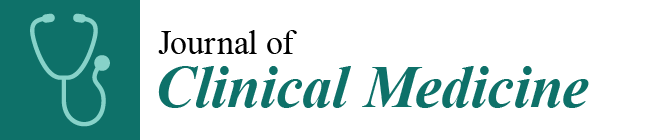
Article Menu
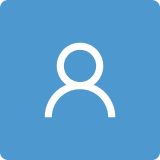
- Subscribe SciFeed
- Recommended Articles
- Google Scholar
- on Google Scholar
- Table of Contents
Find support for a specific problem in the support section of our website.
Please let us know what you think of our products and services.
Visit our dedicated information section to learn more about MDPI.
JSmol Viewer
Incidence, timing, and pattern of atypical recurrence after minimally invasive surgery for urothelial carcinoma.
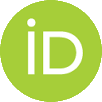
1. Introduction
2. materials and methods, 2.1. literature search strategy, 2.2. inclusion and exclusion criteria, 2.3. data extraction and synthesis, 3. evidence synthesis, 3.1. definition of ar, 3.2. incidence, pattern, and timing of ars, 3.3. surgery-related risk factors.
Author | Type of Study | Study Period | Patients (n) | Procedure | Follow-Up Period (Median (Min–Max or IQR) Months) | Total Recurrences (n (%)) | Atypical Recurrences (n (%)) | Atypical Recurrences Sites (n (%)) | Time from Surgery to AR (Median (Min–Max or IQR) Months) | Survival from Surgery (Median (Min–Max or IQR) Months) |
---|---|---|---|---|---|---|---|---|---|---|
Simone [ ] | Prospective | 2003–2006 | 40 | LRC | 60 | 5 (12) | 1 (2) | Port sites: 1 (2) | N/A | N/A |
Collins [ ] | Retrospective | 2003–2015 | 717 | RARC | 24 | 182 (25) | 7 (1) | Peritoneal: 5 (1) Port sites: 2 (0) | N/A | N/A |
Gandaglia [ ] | Retrospective | 2004–2014 | 155 | RARC | 42 (33.2–50.7) | 83 (54) | 3 (2) | Peritoneal: 3 (2) | N/A | N/A |
Nguyen [ ] | Retrospective | 2001–2015 | 310 | RARC | 24 (14–51) | 81 (26) | 13 (4) | Peritoneal: 13 (4) | 11 (3–17) | N/A |
Tan [ ] | Retrospective | 2011–2014 | 90 | RARC | 16.1 (11.2–27.0) | 17 (19) | 3 (3) | Peritoneal: 2 (2) Port sites: 1 (1) | 8.5 (4.1–16.1) | N/A |
Hussein [ ] | Retrospective | 2003–2016 | 1380 | RARC | 24 | 305 (22) | 22 (2) | Peritoneal: 17 (1) Port sites: 5 (1) | 3 | N/A |
Bochner [ ] | Prospective | 2010–2013 | 60 | RARC | 59 (47–71) | 20 (33) | 5 (8) | Peritoneal: 5 (8) Stoma site: 5 (8) | N/A | N/A |
Niegisch [ ] | Retrospective | 2008–2016 | 89 | RARC | 32 (23–39) | 10 (11) | 1 (1) | Peritoneal: 1 (1) | N/A | N/A |
Venkatramani [ ] | Prospective | N/A | 150 | RARC | 36 | 39 (26) | 2 (1) | Peritoneal: 2 (1) | N/A | N/A |
Kubota [ ] | Retrospective | 2007–2018 | 63 | LRC/RARC | 29 | 17 (27) | 7 (11) | Peritoneal: 6 (10) Port sites: 1 (2) Soft tissues: 2 (3) | 5.5 (2.6–11.8) | 9.3 (3.3–25.2) |
Author (Year) | Type of Study | Study Period | Patients (n) | Procedure | Follow-Up Period (Median (Min–Max or IQR) Months) | Total Recurrences (n (%)) | Atypical Recurrences (n (%)) | Atypical Recurrences Sites (Number (%)) | Time from Surgery to AR (Median (Min–Max or IQR) Months) | Survival from Surgery (Median (Min–Max or IQR) Months) |
---|---|---|---|---|---|---|---|---|---|---|
Manabe [ ] | Retrospective | 2000–2004 | 58 | LRNU | N/A | 30 (52) | 1 (2) | Port sites: 1 (2) | 6,4 | N/A |
Ariane [ ] | Retrospective | 1995–2010 | 150 | LRNU | 27 (10–48) | 21 (14) | 3 (2) | Port sites: 3 (2) | N/A | N/A |
Carrion [ ] | Retrospective | 2007–2012 | 117 | LRNU | 20 (3–97) | 36 (31) | 8 (7) | Peritoneal: 5 (4) Subcutaneous: 2 (2) Abdominal wall: 2 (2) Port sites: 2 (2) | N/A | 2.9 (1.5–33.4) |
Kang [ ] | Retrospective | 2013–2018 | 240 | LRNU | 12.6 (3–45) | N/A | 4 (2) | Port sites: 4 (2) | 4.3 (1–8) | 7 (2–17) |
De Groote [ ] | Retrospective | 2008–2017 | 78 | RARNU | 15 | 22 (28) | 1 (1) | Peritoneal: 1 (1) | 7 (4–7) | N/A |
Morselli [ ] | Retrospective | 2008–2019 | 47 | LRNU | 89.3 | 18 (38) | 3 (6) | Peritoneal: 3 (6) | 3.5 (3–4) | N/A |
Kanno [ ] | Retrospective | 2002–2020 | 283 | LRNU | 31 | N/A | 14 (5) | Retroperitoneal: 12 (4) Port sites: 5 (2) | N/A | 9.9 |
Franco [ ] | Retrospective | 2015–2023 | 1935 | LRNU (779) RARNU (1156) | 28 (14–48) | 624 (32) | 49 (3) | Retroperitoneal: 32 (2) Port sites: 6 (0) Peritoneal: 11 (1) | N/A | N/A |
3.4. Tumor-Related Risk Factors
3.5. limitations, 4. conclusions, author contributions, institutional review board statement, informed consent statement, data availability statement, conflicts of interest.
- Witjes, J.A.; Compérat, E.; Cowan, N.C.; Santis, M.D.; Gakis, G.; Lebrét, T.; Ribal, M.J.; Sherif, A.; Heijden, A.G. Van Der EAU Guidelines on Muscle Invasive and Metastatic Bladder Cancer. Eur. Assoc. Urol. 2024 , 65 , 778–792. [ Google Scholar ] [ CrossRef ] [ PubMed ]
- Rouprêt, M.; Gontero, P.; Birtle, A.; Compérat, E.M.; Dominguez-Escrig, J.L.; Liedberg, F.; Mariappan, P.; Masson-Lecomte, A.; Mostafid, A.H.; Van Rhijn, B.W.G.; et al. EAU Guidelines on Upper Urinary Tract Urothelial Carcinoma ; EAU Guidelines Office: Arnhem, The Netherlands, 2024; ISBN 978-94-92671-23-3. [ Google Scholar ]
- Rai, B.P.; Bondad, J.; Vasdev, N.; Adshead, J.; Lane, T.; Ahmed, K.; Khan, M.S.; Dasgupta, P.; Guru, K.; Chlosta, P.L.; et al. Robotic versus open radical cystectomy for bladder cancer in adults. Cochrane Database Syst. Rev. 2019 , 4 , CD011903. [ Google Scholar ] [ CrossRef ] [ PubMed ]
- Rouprêt, M.; Smyth, G.; Irani, J.; Guy, L.; Davin, J.-L.; Saint, F.; Pfister, C.; Wallerand, H.; Rozet, F. Oncological risk of laparoscopic surgery in urothelial carcinomas. World J. Urol. 2009 , 27 , 81–88. [ Google Scholar ] [ CrossRef ] [ PubMed ]
- Ong, A.M.; Bhayani, S.B.; Pavlovich, C.P. Trocar site recurrence after laparoscopic nephroureterectomy. J. Urol. 2003 , 170 , 1301. [ Google Scholar ] [ CrossRef ] [ PubMed ]
- Simone, G.; Papalia, R.; Guaglianone, S.; Ferriero, M.; Leonardo, C.; Forastiere, E.; Gallucci, M. Laparoscopic versus Open Nephroureterectomy: Perioperative and Oncologic Outcomes from a Randomised Prospective Study. Eur. Urol. 2009 , 56 , 520–526. [ Google Scholar ] [ CrossRef ] [ PubMed ]
- Döbrönte, Z.; Wittmann, T.; Karácsony, G. Rapid development of malignant metastases in the abdominal wall after laparoscopy. Endoscopy 1978 , 10 , 127–130. [ Google Scholar ] [ CrossRef ] [ PubMed ]
- Kubota, M.; Kokubun, H.; Yamaguchi, R.; Murata, S.; Makita, N.; Suzuki, I.; Suzuki, R.; Abe, Y.; Tohi, Y.; Tsutsumi, N.; et al. Atypical oncologic failure after laparoscopic and robot-assisted radical cystectomy at a single institution. Int. J. Clin. Oncol. 2020 , 25 , 1385–1392. [ Google Scholar ] [ CrossRef ] [ PubMed ]
- Tan, W.S.; Sridhar, A.; Ellis, G.; Lamb, B.; Goldstraw, M.; Nathan, S.; Hines, J.; Cathcart, P.; Briggs, T.; Kelly, J. Analysis of open and intracorporeal robotic assisted radical cystectomy shows no significant difference in recurrence patterns and oncological outcomes. Urol. Oncol. Semin. Orig. Investig. 2016 , 34 , 257.e1–257.e9. [ Google Scholar ] [ CrossRef ] [ PubMed ]
- Kanno, T.; Kobori, G.; Ito, K.; Nakagawa, H.; Takahashi, T.; Koterazawa, S.; Takaoka, N.; Somiya, S.; Haitani, T.; Nagahama, K.; et al. Atypical Oncologic Failure After Laparoscopic Radical Nephroureterectomy in a Japanese Multicenter Study. J. Endourol. 2023 , 37 , 793–800. [ Google Scholar ] [ CrossRef ]
- Franco, A.; Ditonno, F.; Antonelli, A.; Wu, Z.; Wang, L.; Finati, M. Atypical Sites of Recurrence after Nephroureterectomy: A Contemporary Analysis from the ROBUUST 2.0 Registry ; Antarctic Press: San Antonio, TX, USA, 2024. [ Google Scholar ]
- Carrion, A.; Huguet, J.; García-Cruz, E.; Izquierdo, L.; Mateu, L.; Musquera, M.; Ribal, M.J.; Alcaraz, A. Intraoperative prognostic factors and atypical patterns of recurrence in patients with upper urinary tract urothelial carcinoma treated with laparoscopic radical nephroureterectomy. Scand. J. Urol. 2016 , 50 , 305–312. [ Google Scholar ] [ CrossRef ]
- Collins, J.W.; Hosseini, A.; Adding, C.; Nyberg, T.; Koupparis, A.; Rowe, E.; Perry, M.; Issa, R.; Schumacher, M.C.; Wijburg, C.; et al. Early Recurrence Patterns Following Totally Intracorporeal Robot-assisted Radical Cystectomy: Results from the EAU Robotic Urology Section (ERUS) Scientific Working Group [figure presented]. Eur. Urol. 2017 , 71 , 723–726. [ Google Scholar ] [ CrossRef ] [ PubMed ]
- Hussein, A.A.; Saar, M.; May, P.R.; Wijburg, C.J.; Richstone, L.; Wagner, A.; Wilson, T.; Yuh, B.; Redorta, J.P.; Dasgupta, P.; et al. Early Oncologic Failure after Robot-Assisted Radical Cystectomy: Results from the International Robotic Cystectomy Consortium. J. Urol. 2017 , 197 , 1427–1436. [ Google Scholar ] [ CrossRef ] [ PubMed ]
- Bochner, B.H.; Dalbagni, G.; Marzouk, K.H.; Sjoberg, D.D.; Lee, J.; Donat, S.M.; Coleman, J.A.; Vickers, A.; Herr, H.W.; Laudone, V.P. Randomized Trial Comparing Open Radical Cystectomy and Robot-assisted Laparoscopic Radical Cystectomy: Oncologic Outcomes. Eur. Urol. 2018 , 74 , 465–471. [ Google Scholar ] [ CrossRef ] [ PubMed ]
- Ariane, M.M.; Colin, P.; Ouzzane, A.; Pignot, G.; Audouin, M.; Cornu, J.N.; Albouy, B.; Guillotreau, J.; Neuzillet, Y.; Crouzet, S.; et al. Assessment of oncologic control obtained after open versus laparoscopic nephroureterectomy for upper urinary tract urothelial carcinomas (UUT-UCs): Results from a large french multicenter collaborative study. Ann. Surg. Oncol. 2012 , 19 , 301–308. [ Google Scholar ] [ CrossRef ] [ PubMed ]
- Kang, Q.; Yu, Y.; Yang, B. Incidence of Port Site Metastasis in Laparoscopic Radical Nephroureterectomy: Single-institution Experience. Urology 2019 , 131 , 130–135. [ Google Scholar ] [ CrossRef ] [ PubMed ]
- Niegisch, G.; Nini, A.; Michalski, R.; Henn, A.; Mally, D.; Albers, P.; Rabenalt, R. Comparison of 2-Year Oncological Outcome and Early Recurrence Patterns in Patients with Urothelial Bladder Carcinoma Treated with Open or Robot-Assisted Radical Cystectomy with an Extracorporeal Urinary Diversion. Urol. Int. 2018 , 101 , 224–231. [ Google Scholar ] [ CrossRef ] [ PubMed ]
- Jancke, G.; Aljabery, F.; Gudjonsson, S.; Hosseini, A.; Sörenby, A.; Wiklund, P.; Liedberg, F. Port-site Metastases After Robot-assisted Radical Cystectomy: Is There a Publication Bias? Eur. Urol. 2018 , 73 , 641–642. [ Google Scholar ] [ CrossRef ] [ PubMed ]
- Nguyen, D.P.; Al Hussein Al Awamlh, B.; O’Malley, P.; Khan, F.; Lewicki, P.J.E.; Golombos, D.M.; Scherr, D.S. Factors Impacting the Occurrence of Local, Distant and Atypical Recurrences after Robot-Assisted Radical Cystectomy: A Detailed Analysis of 310 Patients. J. Urol. 2016 , 196 , 1390–1396. [ Google Scholar ] [ CrossRef ] [ PubMed ]
- Manabe, D.; Saika, T.; Ebara, S.; Uehara, S.; Nagai, A.; Fujita, R.; Irie, S.; Yamada, D.; Tsushima, T.; Nasu, Y.; et al. Comparative Study of Oncologic Outcome of Laparoscopic Nephroureterectomy and Standard Nephroureterectomy for Upper Urinary Tract Transitional Cell Carcinoma. Urology 2007 , 69 , 457–461. [ Google Scholar ] [ CrossRef ]
- De Groote, R.; Decaestecker, K.; Larcher, A.; Buelens, S.; De Bleser, E.; D’Hondt, F.; Schatteman, P.; Lumen, N.; Montorsi, F.; Mottrie, A.; et al. Robot-assisted nephroureterectomy for upper tract urothelial carcinoma: Results from three high-volume robotic surgery institutions. J. Robot. Surg. 2020 , 14 , 211–219. [ Google Scholar ] [ CrossRef ]
- Morselli, S.; Vitelli, F.D.; Verrini, G.; Sebastianelli, A.; Campi, R.; Liaci, A.; Spatafora, P.; Barzaghi, P.; Ferrari, G.; Gacci, M.; et al. Comparison of Tumor Seeding and Recurrence Rate After Laparoscopic vs. Open Nephroureterectomy for Upper Urinary Tract Transitional Cell Carcinoma. Front. Surg. 2021 , 8 , 769527. [ Google Scholar ] [ CrossRef ] [ PubMed ]
- Venkatramani, V.; Reis, I.M.; Castle, E.P.; Gonzalgo, M.L.; Woods, M.E.; Svatek, R.S.; Weizer, A.Z.; Konety, B.R.; Tollefson, M.; Krupski, T.L.; et al. Predictors of Recurrence, and Progression-Free and Overall Survival following Open versus Robotic Radical Cystectomy: Analysis from the RAZOR Trial with a 3-Year Followup. J. Urol. 2020 , 203 , 522–529. [ Google Scholar ] [ CrossRef ] [ PubMed ]
- Kazemier, G.; Bonjer, H.J.; Berends, F.J.; Lange, J.F.; Rae, D.; Bowyer, R.; Wharton, R.; Paterson, I. Port site metastases after laparoscopic colorectal surgery for cure of malignancy. Br. J. Surg. 2005 , 82 , 1141–1142. [ Google Scholar ] [ CrossRef ] [ PubMed ]
- Ost, M.C.; Patel, K.P.; Rastinehad, A.R.; Chu, P.Y.; Anderson, A.E.; Smith, A.D.; Lee, B.R. Pneumoperitoneum with carbon dioxide inhibits macrophage tumor necrosis factor-α secretion: Source of transitional-cell carcinoma port-site metastasis, with prophylactic irrigation strategies to decrease laparoscopic oncologic risks. J. Endourol. 2008 , 22 , 105–111. [ Google Scholar ] [ CrossRef ] [ PubMed ]
- Curet, M.J. Port site metastases. Am. J. Surg. 2004 , 187 , 705–712. [ Google Scholar ] [ CrossRef ] [ PubMed ]
- Iavazzo, C.; Gkegkes, I.D. Port-site metastases in patients with gynecological cancer after robot-assisted operations. Arch. Gynecol. Obstet. 2015 , 292 , 263–269. [ Google Scholar ] [ CrossRef ] [ PubMed ]
- Gandaglia, G.; De Groote, R.; Geurts, N.; D’Hondt, F.; Montorsi, F.; Novara, G.; Mottrie, A. Oncologic Outcomes of Robot-Assisted Radical Cystectomy: Results of a High-Volume Robotic Center. J. Endourol. 2016 , 30 , 75–82. [ Google Scholar ] [ CrossRef ] [ PubMed ]
- Bruins, H.M.; Veskimäe, E.; Hernández, V.; Neuzillet, Y.; Cathomas, R.; Compérat, E.M.; Cowan, N.C.; Gakis, G.; Espinós, E.L.; Lorch, A.; et al. The Importance of Hospital and Surgeon Volume as Major Determinants of Morbidity and Mortality After Radical Cystectomy for Bladder Cancer: A Systematic Review and Recommendations by the European Association of Urology Muscle-invasive and Metastatic Bladder Cancer Guideline Panel. Eur. Urol. Oncol. 2020 , 3 , 131–144. [ Google Scholar ] [ CrossRef ] [ PubMed ]
- Sui, W.; Wallis, C.J.D.; Luckenbaugh, A.N.; Barocas, D.A.; Chang, S.S.; Penson, D.F.; Resnick, M.J.; Laviana, A.A. The Impact of Hospital Volume on Short-term and Long-term Outcomes for Patients Undergoing Radical Nephroureterectomy for Upper Tract Urothelial Carcinoma. Urology 2021 , 147 , 135–142. [ Google Scholar ] [ CrossRef ]
- Margulis, V.; Shariat, S.F.; Matin, S.F.; Kamat, A.M.; Zigeuner, R.; Kikuchi, E.; Lotan, Y.; Weizer, A.; Raman, J.D.; Wood, C.G. Outcomes of radical nephroureterectomy: A series from the upper tract urothelial carcinoma collaboration. Cancer 2009 , 115 , 1224–1233. [ Google Scholar ] [ CrossRef ]
- Liu, W.; Sun, L.; Guan, F.; Wang, F.; Zhang, G. Prognostic Value of Lymphovascular Invasion in Upper Urinary Tract Urothelial Carcinoma after Radical Nephroureterectomy: A Systematic Review and Meta-Analysis. Dis. Markers 2019 , 2019 , 7386140. [ Google Scholar ] [ CrossRef ] [ PubMed ]
Click here to enlarge figure
MIBC | UTUC | |
---|---|---|
Oncological safety of laparoscopic and robot-assisted procedures | Yes | Yes (cT1/cT2,cN0,cM0) Unclear (cT3/cT4/N+/M+) |
Incidence of ARs (% range) | 1–11% | 1–7% |
Pattern (% of all ARs) | Peritoneal: 77% Port sites: 13% Stoma site: 7% Soft tissues: 3% | Retroperitoneal: 50% Peritoneal: 23% Port sites: 23% Subcutaneous: 2% Abdominal wall: 2% |
Timing from surgery to AR onset (median range) | 5.5–11 months | 3.5–7 months |
Survival from diagnosis to death (median range) | 9.3 months | 2.9–7 months |
Surgery-Related Risk Factors | Tumor-Related Risk Factors |
---|---|
The statements, opinions and data contained in all publications are solely those of the individual author(s) and contributor(s) and not of MDPI and/or the editor(s). MDPI and/or the editor(s) disclaim responsibility for any injury to people or property resulting from any ideas, methods, instructions or products referred to in the content. |
Share and Cite
Bignante, G.; Manfredi, C.; Lasorsa, F.; Orsini, A.; Licari, L.C.; Bologna, E.; Roadman, D.F.; Amparore, D.; Lucarelli, G.; Schips, L.; et al. Incidence, Timing, and Pattern of Atypical Recurrence after Minimally Invasive Surgery for Urothelial Carcinoma. J. Clin. Med. 2024 , 13 , 3537. https://doi.org/10.3390/jcm13123537
Bignante G, Manfredi C, Lasorsa F, Orsini A, Licari LC, Bologna E, Roadman DF, Amparore D, Lucarelli G, Schips L, et al. Incidence, Timing, and Pattern of Atypical Recurrence after Minimally Invasive Surgery for Urothelial Carcinoma. Journal of Clinical Medicine . 2024; 13(12):3537. https://doi.org/10.3390/jcm13123537
Bignante, Gabriele, Celeste Manfredi, Francesco Lasorsa, Angelo Orsini, Leslie Claire Licari, Eugenio Bologna, Daniel F. Roadman, Daniele Amparore, Giuseppe Lucarelli, Luigi Schips, and et al. 2024. "Incidence, Timing, and Pattern of Atypical Recurrence after Minimally Invasive Surgery for Urothelial Carcinoma" Journal of Clinical Medicine 13, no. 12: 3537. https://doi.org/10.3390/jcm13123537
Article Metrics
Article access statistics, further information, mdpi initiatives, follow mdpi.
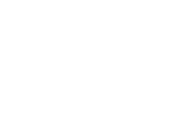
Subscribe to receive issue release notifications and newsletters from MDPI journals
- DOI: 10.1007/s11701-024-02003-8
- Corpus ID: 270198812
Comment on: "A meta‑analysis between robotic hepatectomy and conventional open hepatectomy".
- I. Chen , Li-Chen Chang , K-C Hung
- Published in Journal of Robotic Surgery 1 June 2024
- Medicine, Engineering
5 References
A meta-analysis between robotic hepatectomy and conventional open hepatectomy., impact of intravenous vitamin c as a monotherapy on mortality risk in critically ill patients: a meta-analysis of randomized controlled trials with trial sequential analysis, how to perform and write a trial sequential analysis, impact of intraoperative ketamine on postoperative analgesic requirement following bariatric surgery: a meta-analysis of randomized controlled trials, updated evaluation of endoscopic submucosal dissection versus surgery for early gastric cancer: a systematic review and meta-analysis., related papers.
Showing 1 through 3 of 0 Related Papers
- Share full article
Advertisement
Supported by
Guest Essay
What My Cancer Surgery Taught Me About Immigration

By Glenn Kramon
Mr. Kramon is a lecturer at Stanford Business School.
Wheeled into the operating room last January, staring up at the massive arms of the robot with which a surgeon would remove my cancerous gland, I was hit with an unusual realization: I owe a debt of gratitude to President Lyndon Johnson and the Immigration and Nationality Act of 1965.
Why? Without that legislation, the surgeon who operated on me probably wouldn’t be here. Nor might the doctor who pioneered the procedure. Nor the philanthropist who financed the research. Nor many workers at the company that makes these robots or those at a different company that designed the chips that enable the robot.
As my ordeal with cancer shows, immigration has become critical to our health. Immigrants account for more than a quarter of physicians, surgeons and personal care aides and about a fifth of nursing assistants.
I’m not sure we realize that immigrants help keep us alive: Just look at West Virginia , a state hostile to immigration where aging residents have died before getting off the wait list for home health aides.
While many Americans — including politicians this election year — dwell on stories like the Venezuelan migrant accused of killing a Georgia nursing student, they often forget the critical ways immigration has historically benefited us. A century ago this spring, the United States slammed the door on large sections of the world, and we could be on the verge of doing so again.
That I am so cognizant of the importance of immigrants is the result of two coincidences. The first is that I teach a class on practical writing at Stanford Business School. Frustrated by the cynicism that has pervaded my 3,000 students, many of whom were only teenagers when Donald Trump was elected and are skeptical of the government, I began showing a slide documenting the benefits they enjoy from legislation that originated in the 1960s. For one, many Stanford Business School students — I would guess roughly a quarter — come from families that would not be in this country if not for Mr. Johnson’s Immigration and Nationality Act.
We are having trouble retrieving the article content.
Please enable JavaScript in your browser settings.
Thank you for your patience while we verify access. If you are in Reader mode please exit and log into your Times account, or subscribe for all of The Times.
Thank you for your patience while we verify access.
Already a subscriber? Log in .
Want all of The Times? Subscribe .

An official website of the United States government
The .gov means it’s official. Federal government websites often end in .gov or .mil. Before sharing sensitive information, make sure you’re on a federal government site.
The site is secure. The https:// ensures that you are connecting to the official website and that any information you provide is encrypted and transmitted securely.
- Publications
- Account settings
Preview improvements coming to the PMC website in October 2024. Learn More or Try it out now .
- Advanced Search
- Journal List
- v.239(1); 2004 Jan
Robotic Surgery
Jaydev p. desai.
From the *Department of Mechanical Engineering and Mechanics, Drexel University, Philadelphia and Drexel University College of Medicine, Philadelphia, Pennsylvania.
To review the history, development, and current applications of robotics in surgery.
Background:
Surgical robotics is a new technology that holds significant promise. Robotic surgery is often heralded as the new revolution, and it is one of the most talked about subjects in surgery today. Up to this point in time, however, the drive to develop and obtain robotic devices has been largely driven by the market. There is no doubt that they will become an important tool in the surgical armamentarium, but the extent of their use is still evolving.
A review of the literature was undertaken using Medline. Articles describing the history and development of surgical robots were identified as were articles reporting data on applications.
Several centers are currently using surgical robots and publishing data. Most of these early studies report that robotic surgery is feasible. There is, however, a paucity of data regarding costs and benefits of robotics versus conventional techniques.
Conclusions:
Robotic surgery is still in its infancy and its niche has not yet been well defined. Its current practical uses are mostly confined to smaller surgical procedures.
Robotic surgery is a proliferating new technology despite a paucity of information comparing it with conventional laparoscopy. This article reviews the early data and attempts to give the reader a broad perspective on robotic surgery.
Robotic surgery is a new and exciting emerging technology that is taking the surgical profession by storm. Up to this point, however, the race to acquire and incorporate this emerging technology has primarily been driven by the market. In addition, surgical robots have become the entry fee for centers wanting to be known for excellence in minimally invasive surgery despite the current lack of practical applications. Therefore, robotic devices seem to have more of a marketing role than a practical role. Whether or not robotic devices will grow into a more practical role remains to be seen.
Our goal in writing this review is to provide an objective evaluation of this technology and to touch on some of the subjects that manufacturers of robots do not readily disclose. In this article we discuss the development and evolution of robotic surgery, review current robotic systems, review the current data, discuss the current role of robotics in surgery, and finally we discuss the possible roles of robotic surgery in the future. It is our hope that by the end of this article the reader will be able to make a more informed decision about robotic surgery before “chasing the market.”
BACKGROUND AND HISTORY OF SURGICAL ROBOTS
Since 1921 when Czech playwright Karel Capek introduced the notion and coined the term robot in his play Rossom’s Universal Robots , robots have taken on increasingly more importance both in imagination and reality. 1,2 Robot, taken from the Czech robota , meaning forced labor, has evolved in meaning from dumb machines that perform menial, repetitive tasks to the highly intelligent anthropomorphic robots of popular culture. Although today’s robots are still unintelligent machines, great strides have been made in expanding their utility. Today robots are used to perform highly specific, highly precise, and dangerous tasks in industry and research previously not possible with a human work force. Robots are routinely used to manufacture microprocessors used in computers, explore the deep sea, and work in hazardous environment to name a few. Robotics, however, has been slow to enter the field of medicine.
The lack of crossover between industrial robotics and medicine, particularly surgery, is at an end. Surgical robots have entered the field in force. Robotic telesurgical machines have already been used to perform transcontinental cholecystectomy. 3,4 Voice-activated robotic arms routinely maneuver endoscopic cameras, and complex master slave robotic systems are currently FDA approved, marketed, and used for a variety of procedures. It remains to be seen, however, if history will look on the development of robotic surgery as a profound paradigm shift or as a bump in the road on the way to something even more important.
Paradigm shift or not, the origin of surgical robotics is rooted in the strengths and weaknesses of its predecessors. Minimally invasive surgery began in 1987 with the first laparoscopic cholecystectomy. Since then, the list of procedures performed laparoscopically has grown at a pace consistent with improvements in technology and the technical skill of surgeons. 5 The advantages of minimally invasive surgery are very popular among surgeons, patients, and insurance companies. Incisions are smaller, the risk of infection is less, hospital stays are shorter, if necessary at all, and convalescence is significantly reduced. Many studies have shown that laparoscopic procedures result in decreased hospital stays, a quicker return to the workforce, decreased pain, better cosmesis, and better postoperative immune function. 6–8 As attractive as minimally invasive surgery is, there are several limitations. Some of the more prominent limitations involve the technical and mechanical nature of the equipment. Inherent in current laparoscopic equipment is a loss of haptic feedback (force and tactile), natural hand-eye coordination, and dexterity. Moving the laparoscopic instruments while watching a 2-dimensional video monitor is somewhat counterintuitive. One must move the instrument in the opposite direction from the desired target on the monitor to interact with the site of interest. Hand-eye coordination is therefore compromised. Some refer to this as the fulcrum effect. 9 Current instruments have restricted degrees of motion; most have 4 degrees of motion, whereas the human wrist and hand have 7 degrees of motion. There is also a decreased sense of touch that makes tissue manipulation more heavily dependent on visualization. Finally, physiologic tremors in the surgeon are readily transmitted through the length of rigid instruments. These limitations make more delicate dissections and anastomoses difficult if not impossible. 10 The motivation to develop surgical robots is rooted in the desire to overcome the limitations of current laparoscopic technologies and to expand the benefits of minimally invasive surgery.
From their inception, surgical robots have been envisioned to extend the capabilities of human surgeons beyond the limits of conventional laparoscopy. The history of robotics in surgery begins with the Puma 560, a robot used in 1985 by Kwoh et al to perform neurosurgical biopsies with greater precision. 6,11 Three years later, Davies et al performed a transurethral resection of the prostate using the Puma 560. 12 This system eventually led to the development of PROBOT, a robot designed specifically for transurethral resection of the prostate. While PROBOT was being developed, Integrated Surgical Supplies Ltd. of Sacramento, CA, was developing ROBODOC, a robotic system designed to machine the femur with greater precision in hip replacement surgeries. 1 ROBODOC was the first surgical robot approved by the FDA.
Also in the mid-to-late 1980s a group of researchers at the National Air and Space Administration (NASA) Ames Research Center working on virtual reality became interested in using this information to develop telepresence surgery. 1 This concept of telesurgery became one of the main driving forces behind the development of surgical robots. In the early 1990s, several of the scientists from the NASA-Ames team joined the Stanford Research Institute (SRI). Working with SRI’s other robotocists and virtual reality experts, these scientists developed a dexterous telemanipulator for hand surgery. One of their main design goals was to give the surgeon the sense of operating directly on the patient rather than from across the room. While these robots were being developed, general surgeons and endoscopists joined the development team and realized the potential these systems had in ameliorating the limitations of conventional laparoscopic surgery.
The US Army noticed the work of SRI, and it became interested in the possibility of decreasing wartime mortality by “bringing the surgeon to the wounded soldier—through telepresence.” 1 With funding from the US Army, a system was devised whereby a wounded soldier could be loaded into a vehicle with robotic surgical equipment and be operated on remotely by a surgeon at a nearby Mobile Advanced Surgical Hospital (MASH). This system, it was hoped, would decrease wartime mortality by preventing wounded soldiers from exsanguinating before they reached the hospital. This system has been successfully demonstrated on animal models but has not yet been tested or implemented for actual battlefield casualty care.
Several of the surgeons and engineers working on surgical robotic systems for the Army eventually formed commercial ventures that lead to the introduction of robotics to the civilian surgical community. 1 Notably, Computer Motion, Inc. of Santa Barbara, CA, used seed money provided by the Army to develop the Automated Endoscopic System for Optimal Positioning (AESOP), a robotic arm controlled by the surgeon voice commands to manipulate an endoscopic camera. Shortly after AESOP was marketed, Integrated Surgical Systems (now Intuitive Surgical) of Mountain View, CA, licensed the SRI Green Telepresence Surgery system. This system underwent extensive redesign and was reintroduced as the Da Vinci surgical system. Within a year, Computer Motion put the Zeus system into production.
CURRENT ROBOTIC SURGICAL SYSTEMS
Today, many robots and robot enhancements are being researched and developed. Schurr et al at Eberhard Karls University’s section for minimally invasive surgery have developed a master-slave manipulator system that they call ARTEMIS. 13 This system consists of 2 robotic arms that are controlled by a surgeon at a control console. Dario et al at the MiTech laboratory of Scuola Superiore Sant’Anna in Italy have developed a prototype miniature robotic system for computer-enhanced colonoscopy. 14 This system provides the same functions as conventional colonoscopy systems but it does so with an inchworm-like locomotion using vacuum suction. By allowing the endoscopist to teleoperate or directly supervise this endoscope and with the functional integration of endoscopic tools, they believe this system is not only feasible but may expand the applications of endoluminal diagnosis and surgery. Several other laboratories, including the authors’, are designing and developing systems and models for reality-based haptic feedback in minimally invasive surgery and also combining visual servoing with haptic feedback for robot-assisted surgery. 15–19
In addition to Prodoc, ROBODOC and the systems mentioned above several other robotic systems have been commercially developed and approved by the FDA for general surgical use. These include the AESOP system (Computer Motion Inc., Santa Barbara, CA), a voice-activated robotic endoscope, and the comprehensive master-slave surgical robotic systems, Da Vinci (Intuitive Surgical Inc., Mountain View, CA) and Zeus (Computer Motion Inc., Santa Barbara, CA).
The da Vinci and Zeus systems are similar in their capabilities but different in their approaches to robotic surgery. Both systems are comprehensive master-slave surgical robots with multiple arms operated remotely from a console with video assisted visualization and computer enhancement. In the da Vinci system (Fig. 1) , which evolved from the telepresence machines developed for NASA and the US Army, there are essentially 3 components: a vision cart that holds a dual light source and dual 3-chip cameras, a master console where the operating surgeon sits, and a moveable cart, where 2 instrument arms and the camera arm are mounted. 1 The camera arm contains dual cameras and the image generated is 3-dimensional. The master console consists of an image processing computer that generates a true 3-dimensional image with depth of field; the view port where the surgeon views the image; foot pedals to control electrocautery, camera focus, instrument/camera arm clutches, and master control grips that drive the servant robotic arms at the patient’s side. 6 The instruments are cable driven and provide 7 degrees of freedom. This system displays its 3-dimensional image above the hands of the surgeon so that it gives the surgeon the illusion that the tips of the instruments are an extension of the control grips, thus giving the impression of being at the surgical site.

FIGURE 1. Da Vinci system set up. (Courtesy of Intuitive Surgical Inc., Mountain View, CA)
The Zeus system is composed of a surgeon control console and 3 table-mounted robotic arms (Fig. 2) . The right and left robotic arms replicate the arms of the surgeon, and the third arm is an AESOP voice-controlled robotic endoscope for visualization. In the Zeus system, the surgeon is seated comfortably upright with the video monitor and instrument handles positioned ergonomically to maximize dexterity and allow complete visualization of the OR environment. The system uses both straight shafted endoscopic instruments similar to conventional endoscopic instruments and jointed instruments with articulating end-effectors and 7 degrees of freedom.

FIGURE 2. Zeus system set up. (Courtesy of Computer Motion Inc., Santa Barbara, CA)
ADVANTAGES OF ROBOT-ASSISTED SURGERY
The advantages of these systems are many because they overcome many of the obstacles of laparoscopic surgery (Table 1) . They increase dexterity, restore proper hand-eye coordination and an ergonomic position, and improve visualization (Table 2) . In addition, these systems make surgeries that were technically difficult or unfeasible previously, now possible.
TABLE 1. Advantages and Disadvantages of Conventional Laparoscopic Surgery Versus Robot-Assisted Surgery

TABLE 2. Advantages and Disadvantages of Robot-Assisted Surgery Versus Conventional Surgery

These robotic systems enhance dexterity in several ways. Instruments with increased degrees of freedom greatly enhance the surgeon’s ability to manipulate instruments and thus the tissues. These systems are designed so that the surgeons’ tremor can be compensated on the end-effector motion through appropriate hardware and software filters. In addition, these systems can scale movements so that large movements of the control grips can be transformed into micromotions inside the patient. 6
Another important advantage is the restoration of proper hand-eye coordination and an ergonomic position. These robotic systems eliminate the fulcrum effect, making instrument manipulation more intuitive. With the surgeon sitting at a remote, ergonomically designed workstation, current systems also eliminate the need to twist and turn in awkward positions to move the instruments and visualize the monitor.
By most accounts, the enhanced vision afforded by these systems is remarkable. The 3-dimensional view with depth perception is a marked improvement over the conventional laparoscopic camera views. Also to one’s advantage is the surgeon’s ability to directly control a stable visual field with increased magnification and maneuverability. All of this creates images with increased resolution that, combined with the increased degrees of freedom and enhanced dexterity, greatly enhances the surgeon’s ability to identify and dissect anatomic structures as well as to construct microanastomoses.
DISADVANTAGES OF ROBOT-ASSISTED SURGERY
There are several disadvantages to these systems. First of all, robotic surgery is a new technology and its uses and efficacy have not yet been well established. To date, mostly studies of feasibility have been conducted, and almost no long-term follow up studies have been performed. Many procedures will also have to be redesigned to optimize the use of robotic arms and increase efficiency. However, time will most likely remedy these disadvantages.
Another disadvantage of these systems is their cost. With a price tag of a million dollars, their cost is nearly prohibitive. Whether the price of these systems will fall or rise is a matter of conjecture. Some believe that with improvements in technology and as more experience is gained with robotic systems, the price will fall. 6 Others believe that improvements in technology, such as haptics, increased processor speeds, and more complex and capable software will increase the cost of these systems. 9 Also at issue is the problem of upgrading systems; how much will hospitals and healthcare organizations have to spend on upgrades and how often? In any case, many believe that to justify the purchase of these systems they must gain widespread multidisciplinary use. 9
Another disadvantage is the size of these systems. Both systems have relatively large footprints and relatively cumbersome robotic arms. This is an important disadvantage in today’s already crowded-operating rooms. 9 It may be difficult for both the surgical team and the robot to fit into the operating room. Some suggest that miniaturizing the robotic arms and instruments will address the problems associated with their current size. Others believe that larger operating suites with multiple booms and wall mountings will be needed to accommodate the extra space requirements of robotic surgical systems. The cost of making room for these robots and the cost of the robots themselves make them an especially expensive technology.
One of the potential disadvantages identified is a lack of compatible instruments and equipment. Lack of certain instruments increases reliance on tableside assistants to perform part of the surgery. 6 This, however, is a transient disadvantage because new technologies have and will develop to address these shortcomings.
Most of the disadvantages identified will be remedied with time and improvements in technology. Only time will tell if the use of these systems justifies their cost. If the cost of these systems remains high and they do not reduce the cost of routine procedures, it is unlikely that there will be a robot in every operating room and thus unlikely that they will be used for routine surgeries
CURRENT CLINICAL APPLICATIONS AND EARLY DATA
Several robotic systems are currently approved by the FDA for specific surgical procedures. As mentioned previously, ROBODOC is used to precisely core out the femur in hip replacement surgery. Computer Motion Inc. of Goleta, CA, has 2 systems on the market. One, called AESOP, is a voice-controlled endoscope with 7 degrees of freedom. This system can be used in any laparoscopic procedure to enhance the surgeon’s ability to control a stable image. The Zeus system and the Da Vinci system have been used by a variety of disciplines for laparoscopic surgeries, including cholecystectomies, mitral valve repairs, radical prostatectomies, reversal of tubal ligations, in addition to many gastrointestinal surgeries, nephrectomies, and kidney transplants. The number and types of surgeries being performed with robots is increasing rapidly as more institutions acquire these systems. Perhaps the most notable use of these systems, however, is in totally endoscopic coronary artery grafting, a procedure formerly outside the limitations of laparoscopic technology.
The amount of data being generated on robotic surgery is growing rapidly, and the early data are promising. Many studies have evaluated the feasibility of robot-assisted surgery. One study by Cadiere et al evaluated the feasibility of robotic laparoscopic surgery on 146 patients. 20 Procedures performed with a Da Vinci robot included 39 antireflux procedures, 48 cholecystectomies, 28 tubal reanastomoses, 10 gastroplasties for obesity, 3 inguinal hernia repairs, 3 intrarectal procedures, 2 hysterectomies, 2 cardiac procedures, 2 prostatectomies, 2 artiovenous fistulas, 1 lumbar sympathectomy, 1 appendectomy, 1 laryngeal exploration, 1 varicocele ligation, 1 endometriosis cure, and 1 neosalpingostomy. This study found robotic laparoscopic surgery to be feasible. They also found the robot to be most useful in intra-abdominal microsurgery or for manipulations in very small spaces. They reported no robot related morbidity. Another study by Falcone et al tested the feasibility of robot-assisted laparoscopic microsurgical tubal anastomosis. 31 In this study, 10 patients who had previously undergone tubal sterilization underwent tubal reanastomosis. They found that the 19 tubes were reanastomosed successfully and 17 of the 19 were still patent 6 weeks postoperatively. There have been 5 pregnancies in this group so far. Margossian and Falcone also studied the feasibility of robotic surgery in complex gynecologic surgeries in pigs. 22 In this study, 10 pigs underwent adnexal surgery or hysterectomy using the Zeus robotic system. They found that robotic surgery is safe and feasible for complex gynecologic surgeries. In yet another study by Marescaux et al, the safety and feasibility of telerobotic laparoscopic cholecystectomy was tested in a prospective study of 25 patients undergoing the procedure. 33 Twenty-four of the 25 laparoscopic cholecystectomies were performed successfully, and one was converted to a traditional laparoscopic procedure. This study concluded that robotic laparoscopic cholecystectomy is safe and feasible. Another study by Abbou et al found telerobotic laparoscopic radical prostatectomy to be feasible and safe with dramatically enhanced dexterity. 34
One of the areas where robotic surgery is transforming medicine the most and one of the areas generating the most excitement is minimally invasive cardiac surgery. Several groups have been developing robotic procedures that expand laparoscopic techniques into this previously unexplored territory with encouraging results. Prasad et al successfully constructed left internal thoracic artery (LITA) to left anterior descending (LAD) artery anastomoses on 17 of 19 patients with the use of a robotic system. 21 They conclude that robotically assisted endoscopic coronary bypass surgery showed favorable short-term outcomes with no adverse events and found robotic assistance is an enabling technology that allows surgeons to perform endoscopic coronary anastomoses. Damiano et al conducted a multicenter clinical trial of robotically assisted coronary artery bypass grafting. 35 In this study 32 patients scheduled for primary coronary surgery underwent endoscopic anastomosis of the LITA to LAD. Two-month follow-up revealed a graft patency of 93%. This study concluded that robotic assisted coronary bypass grafting is feasible. In another study, Mohr et al used the Da Vinci system to perform coronary artery bypass grafting on 131 patients and mitral valve repair on 17 patients. 21 They used the robot to perform left internal thoracic artery takedown, LITA-LAD anastomosis in standard sternotomy bypass, and total endoscopic coronary artery bypass grafting LITA-LAD anastomosis on the arrested heart and the beating heart. They found that robotic systems could be used safely in selected patients to perform endoscopic cardiac surgery. Internal thoracic artery takedown is an effective modality, and total endoscopic bypass on an arrested heart is feasible but does not offer a major benefit to the minimally invasive direct approach because cardiopulmonary bypass is still required. Their study suggests that robotic systems have not yet advanced far enough to perform endoscopic closed chest beating heart bypass grafting despite some technical success in 2 of 8 patients. In addition, robotic endoscopic mitral valve repair was successful in 14 of 17 patients. In contrast, several groups in Europe have successfully performed closed-chest, off-pump coronary artery bypass grafting using an endoscopic stabilizer. Kappert and Cichon et al performed 37 off-pump totally endoscopic coronary artery bypass (TECAB) on a beating heart with the Da Vinci system and an endoscopic stabilizer. 32 In this series, they reported a 3.4% rate of conversion to median sternotomy. They concluded that their results promote optimism about further development of TECAB. Another study by Boehm and Reichenspurner et al using a similar stabilizer with the Zeus system had similar results and conclusions about TECAB. 33 Interestingly, a study by Cisowski and Drzewiecki in Poland compared percutaneous stenting with endoscopic coronary artery bypass grafting in patients with single-vessel disease. In this series of 100 patients percutaneous stenting resulted in restenosis in 6% and 12% at 1 and 6 months, respectively, compared with 2% at 6 months in the endoscopic bypass group. 34
Another use for robotic systems being investigated is pediatric laparoscopic surgery. Currently, laparoscopic pediatric surgery is limited by an inability to perform precise anastomoses of 2 to 15 millimeters. 35 Although laparoscopic techniques may be used to treat infants with intestinal atresia, choledochal cysts, biliary atresia, and esophageal atresia, it is not the standard approach because of the technical difficulties. To evaluate the feasibility of robotic systems in pediatric minimally invasive surgery, Hollands and Dixey developed a study where enteroenterostomy, hepaticojejunostomy, and portoentorostomy were performed on piglets. 30 They found all the procedure to be technically feasible with the Zeus robotic system. The study concludes that robotic-assisted laparoscopic techniques are technically feasible in pediatric surgery and may be of benefit in treating various disorders in term and preterm infants. More recently, Hollands and Dixey devised a study using 10 piglets to develop the procedure and evaluate the feasibility of performing a robot-assisted esophagoesophagostomy. In this study, robot-assisted and thoracoscopic approaches were evaluated and compared for leak, narrowing, caliber, mucosal approximation, as well as anesthesia, operative, anastomotic, and robotic set-up times. They found that the robot-assisted approach is feasible. They also discerned no statistically significant difference between the 2 approaches based on the above variables. 31
Despite many studies showing the feasibility of robotic surgery, there is still much to be desired. More high-quality clinical trials need to be performed and much more experience needs to be obtained before the full potential of these systems can be realized.
PRACTICAL USES OF SURGICAL ROBOTS TODAY
In today’s competitive healthcare market, many organizations are interested in making themselves “cutting-edge” institutions with the most advanced technological equipment and the very newest treatment and testing modalities. Doing so allows them to capture more of the healthcare market. Acquiring a surgical robot is in essence the entry fee into marketing an institution’s surgical specialties as “the most advanced.” It is not uncommon, for example, to see a photo of a surgical robot on the cover of a hospital’s marketing brochure and yet see no word mentioning robotic surgery inside.
As far as ideas and science, surgical robotics is a deep, fertile soil. It may come to pass that robotic systems are used very little but the technology they are generating and the advances in ancillary products will continue. Already, the development of robotics is spurring interest in new tissue anastomosis techniques, improving laparoscopic instruments, and digital integration of already existing technologies.
As mentioned previously, applications of robotic surgery are expanding rapidly into many different surgical disciplines. The cost of procuring one of these systems remains high, however, making it unlikely that an institution will acquire more than one or two. This low number of machines and the low number of surgeons trained to use them makes incorporation of robotics in routine surgeries rare. Whether this changes with the passing of time remains to be seen.
THE FUTURE OF ROBOTIC SURGERY
Robotic surgery is in its infancy. Many obstacles and disadvantages will be resolved in time and no doubt many other questions will arise. Many question have yet to be asked; questions such as malpractice liability, credentialing, training requirements, and interstate licensing for tele-surgeons, to name just a few.
Many of current advantages in robotic assisted surgery ensure its continued development and expansion. For example, the sophistication of the controls and the multiple degrees of freedom afforded by the Zeus and da Vinci systems allow increased mobility and no tremor without comprising the visual field to make micro anastomosis possible. Many have made the observation that robotic systems are information systems and as such they have the ability to interface and integrate many of the technologies being developed for and currently used in the operating room. 9 One exciting possibility is expanding the use of preoperative (computed tomography or magnetic resonance) and intraoperative video image fusion to better guide the surgeon in dissection and identifying pathology. These data may also be used to rehearse complex procedures before they are undertaken. The nature of robotic systems also makes the possibility of long-distance intraoperative consultation or guidance possible and it may provide new opportunities for teaching and assessment of new surgeons through mentoring and simulation. Computer Motion, the makers of the Zeus robotic surgical system, is already marketing a device called SOCRATES that allows surgeons at remote sites to connect to an operating room and share video and audio, to use a “telestrator” to highlight anatomy, and to control the AESOP endoscopic camera.
Technically, much remains to be done before robotic surgery’s full potential can be realized. Although these systems have greatly improved dexterity, they have yet to develop the full potential in instrumentation or to incorporate the full range of sensory input. More standard mechanical tools and more energy directed tools need to be developed. Some authors also believe that robotic surgery can be extended into the realm of advanced diagnostic testing with the development and use of ultrasonography, near infrared, and confocal microscopy equipment. 10
Much like the robots in popular culture, the future of robotics in surgery is limited only by imagination. Many future “advancements” are already being researched. Some laboratories, including the authors’ laboratory, are currently working on systems to relay touch sensation from robotic instruments back to the surgeon. 15–19,32 Other laboratories are working on improving current methods and developing new devices for suture-less anastomoses. 33–35 When most people think about robotics, they think about automation. The possibility of automating some tasks is both exciting and controversial. Future systems might include the ability for a surgeon to program the surgery and merely supervise as the robot performs most of the tasks. The possibilities for improvement and advancement are only limited by imagination and cost.
Although still in its infancy, robotic surgery has already proven itself to be of great value, particularly in areas inaccessible to conventional laparoscopic procedures. It remains to be seen, however, if robotic systems will replace conventional laparoscopic instruments in less technically demanding procedures. In any case, robotic technology is set to revolutionize surgery by improving and expanding laparoscopic procedures, advancing surgical technology, and bringing surgery into the digital age. Furthermore, it has the potential to expand surgical treatment modalities beyond the limits of human ability. Whether or not the benefit of its usage overcomes the cost to implement it remains to be seen and much remains to be worked out. Although feasibility has largely been shown, more prospective randomized trials evaluating efficacy and safety must be undertaken. Further research must evaluate cost effectiveness or a true benefit over conventional therapy for robotic surgery to take full root. Table 3 .
TABLE 3. Current Applications of Robotic Surgery

This material is based upon work supported by the National Science †Foundation under Grant No. 0079830 and Grant No. 0133471.
Reprints: Andres E Castellanos, MD, Assistant Professor, Department of Surgery, Drexel University College of Medicine. Mail Stop 413, 245 N. 15th Street, Philadelphia PA 19102. E-mail: [email protected] .

IMAGES
VIDEO
COMMENTS
Review. Fundamentals of robotic surgery. Robotic Surgical Systems. Da Vinci surgical system: The Da Vinci system features multiple robotic arms with surgical instruments.These arms precisely mimic the surgeon's hand movements and provide a more excellent range of motion than traditional laparoscopic instruments [].The surgeons control the robotic arms from a surgical console in the operating room.
Early research on robotic surgery showed the future function of this technology to be uncertain. A study of 372 residents and 56 program directors conducted in 2006 found a little experience and a great deal of ambiguity regarding the current use of robotics. A little over 15% thought robotics was a "trend that would fail," 35% said it was ...
Robotic surgery (RS) is an evolution of minimally invasive surgery that combines medical science, robotics, and engineering. ... The concept of RS originated from research in robotics funded by NASA and the United States Defense Research Advanced Projects Agency during the ... 2021 WSES position paper. de'Angelis N, Khan J, Marchegiani F, et al ...
Journal of Robotic Surgery (JORS) is a peer-reviewed publication dedicated to advancing the field of robotic surgery through the dissemination of cutting-edge research, technological developments, and clinical innovations.. The journal serves as the leading platform for the exchange of knowledge and ideas among researchers, surgeons, engineers, and healthcare professionals in the field of ...
Robotic surgery offers advantages over conventional endoscopic surgery in visualization, dexterity and ergonomics, while maintaining the peri-operative benefits of minimally invasive surgery . The dual-camera system offers 3D views with depth perception, unlike conventional endoscopic views [ 1 ].
Robotic surgery has been widely incorporated into practise despite limited supporting evidence. More rigorous research focused on patient-important benefits is needed before further expansion of robotic surgery. Clinical Outcomes of Robotic Surgery Compared to Conventional Surgical Approaches (Laparoscopic or Open): A Systematic Overview of ...
Since the first surgical robot was introduced in 1985 (the PUMA560, tasked with performing a computed tomography-guided brain biopsy 2), the field of robotic surgery has expanded in size and scope ...
1. Introduction1.1. Robot-Assisted surgery. As the term implies, 'robot-assisted surgery' can be defined as a surgical procedure where a robotic system assists a surgeon in executing the complex, invasive surgical steps on a human body with increased control and flexibility enabling high accuracy and precision when compared to the traditional techniques [1].
Since 2008, more than 14,000 robotic surgeries have been performed at Einstein, with a 72% increase between 2021 and 2022. "Robotic surgery is good for the patient — smaller incisions, reduced ...
Background: From traditional open surgery to laparoscopic surgery and robot-assisted surgery, advances in robotics, machine learning, and imaging are pushing the surgical approach to-wards better clinical outcomes. Pre-clinical and clinical evidence suggests that automation may standardise techniques, increase efficiency, and reduce clinical complications.
Purpose of Review Robotic assistance systems for diagnosis and therapy have become technically mature and widely available. Thus, they play an increasingly important role in patient care. This paper provides an overview of the general concepts of robotically assisted surgical systems, briefly revisiting historical and current developments in the surgical robotics market and discussing current ...
The robot-assistance dental implant robotic systems provide haptic, 75 visual or combined visual and tactile guidance during dental implant surgery. 46,76,77 Throughout the procedure, surgeons ...
minimally invasive robotic surgery (Alexandr a Beier/DLR CC-BY 3.0) 328 Curr Robot Rep (2021) ... Springer Nature supports a reasonable amount of sharing of research papers by authors, subscribers ...
View a PDF of the paper titled Accelerating Surgical Robotics Research: A Review of 10 Years With the da Vinci Research Kit, by Claudia D'Ettorre and 10 other authors View PDF Abstract: Robotic-assisted surgery is now well-established in clinical practice and has become the gold standard clinical treatment option for several clinical indications.
Otolaryngology. The da Vinci surgical system also offers utility in the treatment of tumors of the ears, nose, and throat. A recent case-study reports that the da Vinci robot assisted in the first case of robotic-assisted excision of a cystic hygroma in a patient younger than 2 years old ().The robot provided greater range of motion and reduced tremor in a small area as well as an improved ...
Robotic surgery continues to diffuse across an increasingly broad range of surgical procedures. ... : This study was supported grants 2T32HS000053-27 (Dr Sheetz) and R01HS023597 (Dr Dimick) from the Agency for Healthcare Research and Quality and grant R01AG039434 from the National Institute on Aging, National Institutes of Health. Role of the ...
In urological oncology, where robotic procedures have rapidly become the standard of care, surgery has been revolutionised. In 2019, for example, 92% of radical prostatectomies in the UK were performed with robotic assistance. 1 The landscape has been dominated by the da Vinci surgical system (Intuitive Surgical), but with patents coming to an end a flurry of novel robotic systems are entering ...
Background Robotics represents the most technologically advanced approach in minimally invasive surgery (MIS). Its application in general surgery has increased progressively, with some early experience reported in emergency settings. The present position paper, supported by the World Society of Emergency Surgery (WSES), aims to provide a systematic review of the literature to develop consensus ...
Robotic surgery has been successfully implemented in several hospitals around the globe and has received world wide acceptance. The focus of this paper is to provide a review of the latest robotic ...
The role of robotics has grown exponentially. There is an active interest amongst practitioners in the transferability of the potential benefits into plastic and reconstructive surgery; however, many plastic surgeons report lack of widespread implementation, training, or clinical exposure. We report the current evidence base, and surgical opportunities, alongside key barriers, and limitations ...
system. 137 Analyses of costs for different procedures are made: for robot-assisted laparoscopic rectopexy, there was an. increase in operative costs of e557 or $745 (including material. and time ...
None of the patients suffered POPF Grade C according to the international study group of pancreatic surgery definition. The anastomotic time for mBA was 80 min (54-125 min). Conclusion. Robotic mBA resulted in reasonable outcomes. We propose that mBA could be used as one of the standard methods for robotic pancreatojejunosotomy.
2.3. M7 surgical robot system. SRI's (Stanford Research Institute's) in 1995 formed Intuitive Surgical, Inc. SRI's in 1998 developed the M7 remote surgery robot. M7 has two anthropomorphic robotic arms with seven force‐reflective degrees of freedom. Surgeons can teleoperate using stereo vision. Each arm weights 4.5 kg.
This paper discusses an information fusion sensing technique that can be used in seed implantation surgery, which senses the real-time state of the puncture needle by fusing force and image modes and dramatically improves puncture accuracy and surgical tolerance. ... The International Agency for Research on Cancer (IARC) of the World Health ...
The management of urothelial carcinoma has evolved with the introduction of minimally invasive techniques such as laparoscopic or robotic procedures, challenging the traditional approach of open surgery, and giving rise to atypical recurrences (ARs). ARs include port-site metastasis and peritoneal carcinomatosis, yet discrepancies persist among authors regarding their precise classification ...
DOI: 10.1007/s11701-024-02003-8 Corpus ID: 270198812; Comment on: "A meta‑analysis between robotic hepatectomy and conventional open hepatectomy". @article{Chen2024CommentO, title={Comment on: "A meta‑analysis between robotic hepatectomy and conventional open hepatectomy".}, author={I.-W. Chen and Li-Chen Chang and K-C Hung}, journal={Journal of robotic surgery}, year={2024}, volume={18 1 ...
Higher cost and higher operative time were two of the most common aspects in which robotic surgery compared inferiorly to laparoscopic surgery. Robotic surgery has been previously shown to cost on average between $1000 and $4000 more per case than its laparoscopic or endoscopic counterparts . These analyses are largely based on the DaVinci ...
Dr. Menon could not have pioneered the robotic prostatectomy without someone to finance his research: another Indian immigrant, Raj Vattikuti. A decade after the 1965 act, Mr. Vattikuti went to ...
Robotic surgery is a proliferating new technology despite a paucity of information comparing it with conventional laparoscopy. This article reviews the early data and attempts to give the reader a broad perspective on robotic surgery. Robotic surgery is a new and exciting emerging technology that is taking the surgical profession by storm.