
An official website of the United States government
The .gov means it’s official. Federal government websites often end in .gov or .mil. Before sharing sensitive information, make sure you’re on a federal government site.
The site is secure. The https:// ensures that you are connecting to the official website and that any information you provide is encrypted and transmitted securely.
- Publications
- Account settings
Preview improvements coming to the PMC website in October 2024. Learn More or Try it out now .
- Advanced Search
- Journal List
- GM Crops Food
- v.8(4); 2017

The impact of Genetically Modified (GM) crops in modern agriculture: A review
Ruchir raman.
Faculty of Science (School of Biosciences), The University of Melbourne, Parkville, VIC 3010, Australia
Genetic modification in plants was first recorded 10,000 years ago in Southwest Asia where humans first bred plants through artificial selection and selective breeding. Since then, advancements in agriculture science and technology have brought about the current GM crop revolution. GM crops are promising to mitigate current and future problems in commercial agriculture, with proven case studies in Indian cotton and Australian canola. However, controversial studies such as the Monarch Butterfly study (1999) and the Séralini affair (2012) along with current problems linked to insect resistance and potential health risks have jeopardised its standing with the public and policymakers, even leading to full and partial bans in certain countries. Nevertheless, the current growth rate of the GM seed market at 9.83–10% CAGR along with promising research avenues in biofortification, precise DNA integration and stress tolerance have forecast it to bring productivity and prosperity to commercial agriculture.
INTRODUCTION
Genetic modification (GM) is the area of biotechnology which concerns itself with the manipulation of the genetic material in living organisms, enabling them to perform specific functions. 1 , 2 The earliest concept of modification for domestication and consumption of plants dates back ∼10,000 years where human ancestors practiced “selective breeding” and “artificial selection” – the Darwinian-coined terms broadly referring to selection of parent organisms having desirable traits (eg: hardier stems) and breeding them for propagating their traits. The most dramatic alteration of plant genetics using these methods occurred through artificial selection of corn – from a weedy grass possessing tiny ears and few kernels (teosinte; earliest recorded growth: central Balsas river valley, southern Mexico 6300 years ago) to the current cultivars of edible corn and maize plants (Doebley et al., 2016, Fig 1 ). The use of similar techniques has also been reported to derive current variants of apples, broccoli and bananas different from their ancestral plant forms which are vastly desirable for human consumption. 3

The evolution of modern corn/maize (top) from teosinte plants (bottom) by repetitive selective breeding over several generations. [Sources: 50 (top figure), 51 (bottom figure)].
The developments leading to modern genetic modification took place in 1946 where scientists first discovered that genetic material was transferable between different species. This was followed by DNA double helical structure discovery and conception of the central dogma – the transcription of DNA to RNA and subsequent translation into proteins – by Watson and Crick in 1954. Consequently, a series of breakthrough experiments by Boyer and Cohen in 1973, which involved “cutting and pasting” DNA between different species using restriction endonucleases and DNA ligase – “molecular scissors and glue” (Rangel, 2016) successfully engineered the world's first GM organism. In agriculture, the first GM plants – antibiotic resistant tobacco and petunia – were successfully created in 1983 by three independent research groups. In 1990, China became the first country to commercialise GM tobacco for virus resistance. In 1994, the Flavr Savr tomato (Calgene, USA) became the first ever Food and Drug Administration (FDA) approved GM plant for human consumption. This tomato was genetically modified by antisense technology to interfere with polygalacturonase enzyme production, consequently causing delayed ripening and resistance to rot. 4 Since then, several transgenic crops received approvals for large scale human production in 1995 and 1996. Initial FDA-approved plants included corn/maize, cotton and potatoes ( Bacillus thuringiensis (Bt) gene modification, Ciba-Geigy and Monsanto) canola (Calgene: increased oil production), cotton (Calgene: bromoxynil resistance) and Roundup Ready soybeans (Monsanto: glyphosate resistance), 4 Fig 2 ). Currently, the GM crop pipeline has expanded to cover other fruits, vegetables and cereals such as lettuce, strawberries, eggplant, sugarcane, rice, wheat, carrots etc. with planned uses to increase vaccine bioproduction, nutrients in animal feed as well as confer salinity and drought resistant traits for plant growth in unfavourable climates and environment. 4 , 2

A timeline of events leading to the current GM crop era.
Since their commercialisation, GM crops have been beneficial to both economy and the environment. The global food crop yield (1996–2013) has increased by > 370 million tonnes over a relatively small acreage area. 2 Furthermore, GM crops have been recorded to reduce environmental and ecological impacts, leading to increases in species diversity. It is therefore unsurprising that GM crops have been commended by agricultural scientists, growers and most environmentalists worldwide.
Nevertheless, advancements in GM crops have raised significant questions of their safety and efficacy. The GM seed industry has been plagued with problems related to human health and insect resistance which have seriously undermined their beneficial effects. Moreover, poor science communication by seed companies, a significant lack of safety studies and current mistrust regarding GMOs have only compounded problems. These have led many countries, particularly the European Union and Middle East to implement partial or full restrictions on GM crops. GM agriculture is now widely discussed in both positive and negative frames, and currently serves as a hotbed of debate in public and policymaking levels.
CHALLENGES IN COMMERCIAL AGRICULTURE
The agriculture industry has been valued at an estimated US$ 3.2 trillion worldwide and accounts for a large share of the GDP and employment in developing and underdeveloped nations. 5 For instance: Agriculture contributes only 1.4% towards the GDP and 1.62% of the workforce in US in comparison with South Asian regions, where it contributes 18.6% towards the GDP and 50% of the workforce. 6 However, despite employing nearly 1 in 5 people worldwide (19% of the world's population), 7 the agriculture industry is projected to suffer significant global setbacks (population growth, pest resistance and burden on natural resources) by 2050, which has been elaborated further in this section.
Explosive Population Growth
The Food and Agricultural Organisation projects the global population to grow to approximately 9.7 billion by 2050 – a near 50% increase from 2013 – and further to an estimated 11bn by 2100. Current agricultural practices alone cannot sustain the world population and eradicate malnutrition and hunger on a global scale in the future. Indeed, the FAO also estimates that despite a significant reduction in global hunger, 653 mn people will still be undernourished in 2030. 8 Additionally, Ray et al. and other studies depict the top four global crops (soybean, maize, wheat and rice) are increasing at 1.0%, 0.9%, 1.6% and 1.3% per annum respectively– approximately 42%, 38%, 67% and 55% lower than the required growth rate (2.4%/annum) to sustain the global population in 2050. 9 Compounded with other problems such as improved nutritional standards in the burgeoning lower-middle class and projected loss in arable land (from 0.242 ha/person in 2016 to 0.18 ha/person in 2050) 2 due to degradation and accelerated urbanization, rapid world population expansion will increase demand for food resources.
Pests and Crop Diseases
Annual crop loss to pests alone account for 20–40% of the global crop losses. In terms of economic value, tackling crop diseases and epidemics and invasive insect problem costs the agriculture industry approximately $290 mn annually. 8 Currently, major epidemics continue to plague commercial agriculture. It has been projected that crop disease and pest incidences are expanding in a poleward direction (2.7 km annually), 10 indicated by coffee leaf rust and wheat rust outbreaks in Central America. These incidences have largely been attributed to an amalgamation of globalisation leading to increased plant, pest and disease movement, increase in disease vectors, climate change and global warming. 8
While integrated pest management and prevention techniques somewhat mitigate the pest problem, they are insufficient to tackle the transboundary crop-demics. The epidemiology of the Panama disease (or Panama wilt), caused by the soil fungus Fusarium oxysporum f.sp. cubense (Foc) 11 provides solid evidence in this regard. Since the early-mid 1990s the Tropical Race-4 (TR4) strain, a single pathogen Foc fungus clone, has significantly crippled the global banana industry. In 2013, the Mindanao Banana Farmers and Exporters association (in Philippines) reported infection in 5900 hectares of bananas, including 3000 hectares that were abandoned. In Mozambique, symptomatic plants currently account for >20% of total banana plantations (570,000 out 2.5m) since the reporting of TR4 in 2015. Additionally, TR4 losses have cost Taiwanese, Malaysian, and Indonesian economies a combined estimate of US$ 388.4 mn. 12 Therefore, an alarming increase in transboundary crop and pest diseases have broad environmental, social and economic impacts on farmers and threaten food security.
Burden on Natural Resources
The FAO's 2050 projections suggest projected natural resource scarcities for crop care. 8 Despite overall agricultural efficiency, unsustainable competition has intensified due to urbanisation, population growth, industrialisation and climate change. Deforestation for agricultural purposes has driven 80% of the deforestation worldwide. In tropical and subtropical areas where deforestation is still widespread, agricultural expansion accounted for loss of 7 million hectares per annum of natural forests between 2000–2010. 8 Additionally, water withdrawals for agriculture accounted for 70% of all withdrawals, seriously depleting natural water resources in many countries. This has particularly been observed in low rainfall regions, such as Middle East, North Africa and Central Asia where water for agriculture accounts for 80–90% 8 of the total water withdrawal. These trends are predicted to continue well into the 21st century and therefore increase the burden of natural resource consumption globally.
SOLUTIONS PROVIDED BY GM CROPS
GM crops have been largely successful in mitigating the above major agriculture challenges while providing numerous benefits to growers worldwide. From 1996–2013, they generated $117.6 bn over 17 years in global farm income benefit alone. The global yearly net income increased by 34.3% in 2010–2012. 13 , 14 Furthermore, while increasing global yield by 22%, GM crops reduced pesticide (active ingredient) usage by 37% and environmental impact (insecticide and herbicide use) by 18%. 15 To achieve the same yield standards more than 300 million acres of conventional crops would have been needed, which would have further compounded current environmental and socioeconomic problems in agriculture. 2
To further emphasise the impact of GM crops on economies: two case studies – GM Canola (Australia) and GM cotton (India) – have been highlighted in this review.
GM Cotton (India)
In India, cotton has served as an important fibre and textile raw material and plays a vital role in its industrial and agricultural economy. Nearly 8 million farmers, most of them small and medium (having less than 15 acres of farm size and an average of 3–4 acres of cotton holdings) depend on this crop for their livelihood. In 2002, Monsanto-Mahyco introduced Bollgard-I, India's first GM cotton hybrid containing Cry1Ac -producing Bacillus thuringiensis ( Bt ) genes for controlling the pink bollworm ( P. gossypiella ) pest. 16 Initially, only 36% of the farmers adopted the new crop however this statistic soon grew to 46% in 2004 17 after Bt- cotton was approved nationwide. This was followed by approval and launch of Bollgard-II (a two-toxin Cry1Ac and Cry2Ab -producing Bt- pyramid conferring resistance to bollworm) by Monsanto-Mahyco, which subsequently enhanced Bt- cotton adoption among Indian cotton growers ( Fig 3 ).

Adoption of GM canola (top) and GM cotton (bottom) in Australia and India respectively. The primary vertical axis shows the total acreage of cotton and canola along with the proportion of GM and non-GM crops grown per year, while the secondary horizontal axis depicts the percentage of GM crop adoption among farmers and growers per year. (Sources: 22 , 18 ).
Despite controversies, Bt -cotton's implementation has largely benefited Indian farmers and agricultural economy. Bt -cotton has increased profits and yield by Rs. 1877 per acre (US$38) and 126 kg/acre of farmland respectively, 50% and 24% more than profit and yield by conventional cotton. This translates to a net increase of Bt -cotton growers' annual consumption expenditures by 18% (Rs. 15,841/US$321) compared to non-adapters, highlighting improved living standards. 17 Bt -cotton adoption has also resulted in a 22-fold increase in India's agri-biotech industry due to an unprecedented 212-fold rise in plantings from 2002–2011 (accounting for ∼30% of global cotton farmland), surpassing China and making it a world leading grower and exporter. 7 million out of the 8 million farmers (88%) are growing Bt-cotton annually. Cotton crop yields have also increased 31% while conversely insecticide usage has more than halved (46% to 21%) enhancing India's cotton income by US$11.9 bn. 18 Therefore, Bt- cotton has resulted in economic prosperity among Bt -cotton growers, with 2002–11 often being called a white gold period for India's GM cotton industry.
GM Canola (Australia)
Canola in Australia is grown as a break crop, providing farmers a profitable alternative along with rotational benefits from continuous cereal crop phases and their related weed/pest mechanisms. Other benefits include broadleaf weed and cereal root disease control and better successive cereal crop growth. It is most prominently grown in Western Australia (WA), where it accounts for 400–800,000 ha of farmland and is the most successful of four break crops (oat, lupin, canola and field pea). From 2002–2007, Canola production in WA alone accounted for a yield of 440 mn tonnes valued at A$200mn. 19 Nevertheless Canola has been a high risk crop and particularly susceptible to blackleg disease (caused by fungus Leptosphaeria maculans ), and weeds such as charlock ( Sinapis arvensis ), wild radish ( Raphanus raphanistrum L) and Buchan ( Hirschfeldia incana (L.) Lagr.-Foss) which increase anti-nutritional compound content and composition in canola oil, degrading quality. 20
In 2008–09, two herbicide resistant GM canola varieties: Roundup Ready® (Monsanto) and InVigor® (Bayer Cropsciences) were introduced in Australia. Roundup Ready® contained gene variants with altered EPSP synthase (5-enolpyruvylshikimate-3-phosphate) alterations along with a glyphosate oxidoreductase gene making it glyphosate resistant. It gained OGTR approval after trials showed its environmental impact was less than half (43%) of triazine tolerant canola varieties 21 , 19 and remains the only OGTR-approved GM canola till date. The introduction of Roundup Ready® canola has had a positive impact on farmers by controlling weeds that were erstwhile difficult to mitigate. In 2014, GM canola planting area (hectares) was up to 14% in 2014 from just 4% in 2009 ( Fig 3 ), representing a near three-fold increase and contributing to Australia's growing biotech crop hectarage. This increase was more notable in WA, where GM canola was planted from 21% canola farmers in 2014, up from 0% in 2009. 22 This has led to more research and development of different canola varieties to improve oil content and quality, yield and maturity. 20
PROBLEMS AND CONTROVERSIES
Although a successful technology, GM crop use has been controversial and a hotbed for opposition. Their public image has been severely impacted leading to full or partial bans in 38 countries including the European Union ( Fig 4 ). This section highlights major controversies and reflects on some real problems faced by commercialised GM crops.

The figure depicts the current acceptance of GM crops in different countries. Green: National bans. Yellow: Restrictive laws, Red: No formal laws (Source: 52 ).
Monarch Butterfly Controversy (1999)
The Monarch butterfly controversy relates Losey et al.’s publication in Nature wherein they compared Monarch butterfly ( Danaus plexippus ) larval feeding cycle of milkweed ( Asclepias curassavica) dusted with N4640- Bt maize pollen to a control (milkweed dusted with untransformed corn pollen). They observed the N4640- Bt reared larvae to eat lesser, grow slower and have higher mortality and predicted N4640- Bt maize to have significant off target effects and significantly impact Monarch populations due to the following reasons:
- • Monarch larvae's main nutrition is derived from milkweed, which commonly occurs in and around the corn field edges.
- • Maize pollen shedding coincides with monarch larval feeding cycles during seasonal summer.
- • ∼50% of the Monarch population is concentrated within the US maize belt during summer, a region known for intense maize production. 23
Losey et al. ’s conclusions were challenged by academics for improper experimental design and validity and soundness of extrapolating laboratory assays to field testing. There were many subsequent studies performed, depicting Bt- maize to be highly unlikely to affect Monarch population. For instance: Pleasants et al., 24 reasoned that several factors, most notably rainfall (reducing pollen by 54–86%) and leaf pollen distribution (30–50% on upper plant portions/preferred larval feeding sites) reduced larval exposure to Bt- maize pollen 24 and Sears et al., 25 argued that Bt- maize production, should it rise to ∼80% would only affect 0.05%-6% monarch population. 25
Nevertheless, Losey et al. ’s results garnered acclaim in the press for raising both the public's and biotech companies' consciousness about possible off-target Bt- maize on monarch butterflies. However further attempts to extrapolate their results to other Bt and GM crops have been unsuccessful, with current evidence suggesting effectiveness in insect control without off-target effects. 25
The Séralini Affair (2012)
The Séralini affair concerns itself with a controversial GM crop study by Gilles-Éric Séralini in Springer during 2012–14. The original paper published in 2012 studied the effect of NK-603 Roundup Ready® Maize (NK-603 RR Maize) on rats. It used the same experimental setup as an earlier Monsanto safety study to gain maize approval 26 and reached the following observations:
- • Significant chronic kidney deficiencies representing 76% of altered parameters.
- • 3–5x higher incidence of necrosis and liver congestions in treated males.
- • 2–3-fold increase in female treatment group mortality.
- • High tumour incidences in both treated sexes, starting 600 days earlier than control (only one tumour noted in control).
The 2012 study attributed observations to EPSPS overexpression in NK-603 RR Maize, found the Monsanto study conclusions “unjustifiable” and recommended thorough long-term toxicity feeding studies on edible GM crops. 27 The paper divided opinion, with Séralini being framed as both as a hero of the anti-GM movement and as an unethical researcher. His paper drew heavy criticism for its flawed experimental design, animal type used for study, statistical analysis and data presentation deficiencies and overall misrepresentations of science and was retracted (Arjó et al., 2012,. 28 In 2014, Séralini republished his nearly-identical study in expanded form which since continues to fuel the GM crop debate.
GM Crops: An Imperfect Technology
Despite the above controversies being proven unfounded, GM crops are an “imperfect technology” with potential major health risks of toxicity, allergenicity and genetic hazards associated to them. These could be caused by inserted gene products and their potential pleiotropic effects, the GMO's natural gene disruption or a combination of both factors. 4 , 2 The most notable example of this is Starlink maize, a Cry9c- expressing cultivar conferring gluphosinate resistance. In the mid-1990s, the USDA's Scientific Advisory Panel (SAP) classified Cry9c Starlink as “potentially allergenic” due to its potential to interact with the human immune system. In 1998, the US Environment Protection Agency (EPA) granted approval for Starlink's use in commercial animal feed and industry (eg: biofuels) but banned it for human consumption. Following this, relatively small Starlink quantities (∼0.5% of the US corn acreage) were planted between 1998–2000. 29 , 30 In 2000, Starlink residues were detected in food supplies not only in USA but also EU, Japan and South Korea where it completely banned. Furthermore, the EPA received several adverse allergic event reports related to corn, prompting a worldwide Starlink recall. About 300 different maize products were recalled in US alone by Kellogg's and Mission Foods. Starlink inadvertently affected ∼50% of US maize supply and depressed US corn prices by 8% for CY2001. 31
Another problem faced by GM crops currently is pest resistance due to gene overexpression leading to pest evolution via natural selection. Indeed, an analysis of 77 studies' results by Tabashnik et al. depicted reduced Bt- crop efficacy caused by field evolved pest resistance for 5 out of 13 (38.4%) major pest species examined in 2013, compared to just one in 2005, 32 Table 1 ). Furthermore, such resistance can be evolved over several generations in a relatively short time as most insects have shorter life spans. In maize, S.frugiperda and B.fusca resistance was reported after just 3 and 8 years respectively, consistent with the worst case scenarios. In the former, it led to crop withdrawal in Puerto Rico and was reported to still affect maize growers in 2011, 4 years after crop withdrawal. In India, P. gossypiella resistance currently affects ∼90% Bollgard-II Bt- hybrid cotton growers and ∼35% (4 million ha) of cultivable cotton area in key regions. 32 , 33
Crops reported with >50% pest resistance and reduced efficacy.
1- Time to first reported resistance of pest to GM plant. 2-Toxin secreted by affected GM plant.
To mitigate the problems regarding GM technologies, a series of strict regulatory measures have been proposed to prevent cross-contamination of split-approved GM crops banned for human consumption. These include implementation and enforcement buffer zones to prevent cross contamination of crops, better laboratory testing to confirm adverse allergic event cases and an overall inclusion of stakeholders and representatives in policymaking and communication. 30 Additionally, Bt pest resistance could be controlled by implementation of high-dose Bt toxin standards in transgenic crops and evaluation of insect responses, integration of Host plant resistance (HPR) traits in cultivars to control secondary pests, 34 preparation of abundant non- Bt plants refuges near Bt crops and proactive implementation of two-toxin Bt- pyramids producing ≥ 2 distinct toxins against as single pest species. 32 These suggested measures in pest management and regulation if implemented could help the agriculture industry overcome the imperfect problems of GM crops while significantly regaining public trust of this technology.
GM AGRICULTURE: TRENDS AND FUTURE AVENUES
The GM seed market has changed drastically since 1996 from a competitive sector owned by family owners to one of the fastest growing global industries dominated by a small number of corporations. Analysts predict a Compounded Annual Growth Rate (CAGR) between 9.83–10% between 2017–2022 for this industry where it is projected to reach US$113.28 bn, an approximately four-fold increase from US$26.7 bn in 2007, 35 , 36 MarketWatch, 2016). This has been attributed to a rising biofuel adoption in lieu of conventional fuels in Asia-Pacific (APAC) and Africa, leading to increase plantings of energy crops (wheat, sugarcane, corn and soybean) for production. Nevertheless, despite growth spikes in APAC and Africa, North America currently dominates the GM seed industry with a market share of ∼30%, and is forecast to do so in 2020 (MarketWatch, 2017).
The GM seed market has currently been consolidated by the “big five” companies: Monsanto, Bayer CropScience, Dupont, Syngenta and Groupe Limagrain ( Table 2 ). As of 2016, they account for 70% of the market (up from ∼60% in 2009). 37 , 38 The “big five” players are currently acquiring and forming joint ventures with smaller firms and competitors on a transnational scale, serving as strong entry barriers in this industry. 36 Since 2016, major ongoing Mergers and Acquisitions (M&As): Syngenta's takeover by ChemChina (completed June 2017- US$43 bn), 39 Bayer-Monsanto merger (ongoing- $66bn) 40 and Dow-Dupont merger (∼US$140 bn- antitrust approval) 41 have been happening in the industry. Only time will determine how these M&As impact the industry, growers and consumers.
A snapshot of the “big five” GM seed companies.
1 – Converted from EUR at current NASDAQ rates (July 2017), 2 – Ongoing Merger/Acquisition, 3- Completed Merger/Acquisition, 4- Public non-quoted company, 5- Sourced from Hoovers D&B, 2017, 6 – In this case, market share represents global market share and market capitalisation is local.
The latest reports indicate that the agriculture industry invests around $69 billion globally on its Research and Development (R&D). 42 This investment has fuelled research many emerging avenues for GM crop technology. However, innovation has strictly been influenced by the “big five” due to broad patent claims, and high research, legal and development costs for patent eligible products. For instance, the top 3 seed companies controlled 85% transgenic and 70% non-transgenic corn patents in USA in 2009. 36
In the GM seed market, R&D is currently occurring in the conventional areas of insect resistance, increased crop yield and herbicide tolerance. Increasing R&D has also been invested on precision site-directed nuclease techniques (CRISPR, ZFNs and TALENs) for desired gene integration in host plants. 14 , 43 Current studies show negligible/zero off target mutations (Schnell et al., 2015,. 44 This is starkly contrasting to conventional breeding techniques which are often associated with undesired alteration risks via linkage drag and random, unspecified mutations. 45 Additionally, biofortification and stress tolerance have been identified as areas for future GM seed research. Both fields are currently of major interest with a growing body of scientific studies. They tackle key problems: while biofortification addresses malnutrition and micronutrient deficiency; stress tolerance addresses biodegradation, climate change and shrinking cultivable area. Since the development of Vitamin-A biofortified rice in 2000, 46 studies highlight further extrapolation in enhancing human diet using biofortifications, with recorded success in iron and zinc. 47 Moreover, recent genetic modification studies in Arabidopsis and Barley have depicted adaptation to stress tolerance and biomass growth in adverse conditions (Mendiondo et al., 2016,. 48 Three stress-tolerant corn hybrids [Pioneer Optimum AQUAmax™ (Dupont Pioneer), Syngenta Artesian™ (Syngenta) and Genuity™ DroughtGard™ (Monsanto)] are currently being marketed for drought resistance, 49 showcasing enormous potential for economic profitability in the above areas.
GM crops can mitigate several current challenges in commercial agriculture. Current market trends project them as one of the fastest growing and innovative global industries, which not only benefit growers but also consumers and major country economies. However, it is imperative that the agricultural industry and science community invest in better science communication and regulation to tackle unethical research and misinformation. Imperfections and major GM technology can also be combated by stricter regulation, monitoring and implementation by government agriculture bodies, a globally improved risk mitigation strategy and communication with growers, therefore ensuring greater acceptance. With key innovation in precision gene-integration technologies and emerging research in biofortification and stress tolerance, GM crops are forecast to bring productivity and profitability in commercial agriculture for smoother progress in the future.
ACKNOWLEDGMENTS
Although this review article is my own work, it would not have been possible without certain people. I would like to thank the editor and the reviewers for their helpful comments and remarks. I would also like to extend my gratitude towards the University of Melbourne staff, especially Dr. Matthew Digby and Mrs. Fiona Simpson for their encouragement in this venture. I would further extend my thanks to my peers, teachers and other people I met during my academic journey. Lastly, I would like to extend my deepest appreciation towards my family, who encouraged me to pursue a scientific career in Biotechnology and have been wonderfully supportive of my career goals. This review article is my maiden article in an academic journal, and I would like to thank all the readers for being a part of it.
Click through the PLOS taxonomy to find articles in your field.
For more information about PLOS Subject Areas, click here .
Loading metrics
Open Access
Peer-reviewed
Research Article
A Meta-Analysis of the Impacts of Genetically Modified Crops
Affiliation Department of Agricultural Economics and Rural Development, Georg-August-University of Goettingen, Goettingen, Germany
* E-mail: [email protected]
- Wilhelm Klümper,
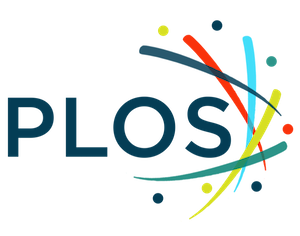
- Published: November 3, 2014
- https://doi.org/10.1371/journal.pone.0111629
- Reader Comments
Despite the rapid adoption of genetically modified (GM) crops by farmers in many countries, controversies about this technology continue. Uncertainty about GM crop impacts is one reason for widespread public suspicion.
We carry out a meta-analysis of the agronomic and economic impacts of GM crops to consolidate the evidence.
Data Sources
Original studies for inclusion were identified through keyword searches in ISI Web of Knowledge, Google Scholar, EconLit, and AgEcon Search.
Study Eligibility Criteria
Studies were included when they build on primary data from farm surveys or field trials anywhere in the world, and when they report impacts of GM soybean, maize, or cotton on crop yields, pesticide use, and/or farmer profits. In total, 147 original studies were included.
Synthesis Methods
Analysis of mean impacts and meta-regressions to examine factors that influence outcomes.
On average, GM technology adoption has reduced chemical pesticide use by 37%, increased crop yields by 22%, and increased farmer profits by 68%. Yield gains and pesticide reductions are larger for insect-resistant crops than for herbicide-tolerant crops. Yield and profit gains are higher in developing countries than in developed countries.
Limitations
Several of the original studies did not report sample sizes and measures of variance.
The meta-analysis reveals robust evidence of GM crop benefits for farmers in developed and developing countries. Such evidence may help to gradually increase public trust in this technology.
Citation: Klümper W, Qaim M (2014) A Meta-Analysis of the Impacts of Genetically Modified Crops. PLoS ONE 9(11): e111629. https://doi.org/10.1371/journal.pone.0111629
Editor: emidio albertini, University of Perugia, Italy
Received: June 23, 2014; Accepted: October 3, 2014; Published: November 3, 2014
Copyright: © 2014 Klümper, Qaim. This is an open-access article distributed under the terms of the Creative Commons Attribution License , which permits unrestricted use, distribution, and reproduction in any medium, provided the original author and source are credited.
Data Availability: The authors confirm that all data underlying the findings are fully available without restriction. All relevant data are within the paper and its Supporting Information files.
Funding: This research was financially supported by the German Federal Ministry of Economic Cooperation and Development (BMZ) and the European Union’s Seventh Framework Programme (FP7/2007-2011) under Grant Agreement 290693 FOODSECURE. The funders had no role in study design, data collection and analysis, decision to publish, or preparation of the manuscript. Neither BMZ nor FOODSECURE and any of its partner organizations, any organization of the European Union or the European Commission are accountable for the content of this article.
Competing interests: The authors have declared that no competing interests exist.
Introduction
Despite the rapid adoption of genetically modified (GM) crops by farmers in many countries, public controversies about the risks and benefits continue [1] – [4] . Numerous independent science academies and regulatory bodies have reviewed the evidence about risks, concluding that commercialized GM crops are safe for human consumption and the environment [5] – [7] . There are also plenty of studies showing that GM crops cause benefits in terms of higher yields and cost savings in agricultural production [8] – [12] , and welfare gains among adopting farm households [13] – [15] . However, some argue that the evidence about impacts is mixed and that studies showing large benefits may have problems with the data and methods used [16] – [18] . Uncertainty about GM crop impacts is one reason for the widespread public suspicion towards this technology. We have carried out a meta-analysis that may help to consolidate the evidence.
While earlier reviews of GM crop impacts exist [19] – [22] , our approach adds to the knowledge in two important ways. First, we include more recent studies into the meta-analysis. In the emerging literature on GM crop impacts, new studies are published continuously, broadening the geographical area covered, the methods used, and the type of outcome variables considered. For instance, in addition to other impacts we analyze effects of GM crop adoption on pesticide quantity, which previous meta-analyses could not because of the limited number of observations for this particular outcome variable. Second, we go beyond average impacts and use meta-regressions to explain impact heterogeneity and test for possible biases.
Our meta-analysis concentrates on the most important GM crops, including herbicide-tolerant (HT) soybean, maize, and cotton, as well as insect-resistant (IR) maize and cotton. For these crops, a sufficiently large number of original impact studies have been published to estimate meaningful average effect sizes. We estimate mean impacts of GM crop adoption on crop yield, pesticide quantity, pesticide cost, total production cost, and farmer profit. Furthermore, we analyze several factors that may influence outcomes, such as geographic location, modified crop trait, and type of data and methods used in the original studies.
Materials and Methods
Literature search.
Original studies for inclusion in this meta-analysis were identified through keyword searches in relevant literature databanks. Studies were searched in the ISI Web of Knowledge, Google Scholar, EconLit, and AgEcon Search. We searched for studies in the English language that were published after 1995. We did not extend the review to earlier years, because the commercial adoption of GM crops started only in the mid-1990s [23] . The search was performed for combinations of keywords related to GM technology and related to the outcome of interest. Concrete keywords used related to GM technology were (an asterisk is a replacement for any ending of the respective term; quotation marks indicate that the term was used as a whole, not each word alone): GM*, “genetically engineered”, “genetically modified”, transgenic, “agricultural biotechnology”, HT, “herbicide tolerant”, Roundup, Bt, “insect resistant”. Concrete keywords used related to outcome variables were: impact*, effect*, benefit*, yield*, economic*, income*, cost*, soci*, pesticide*, herbicide*, insecticide*, productivity*, margin*, profit*. The search was completed in March 2014.
Most of the publications in the ISI Web of Knowledge are articles in academic journals, while Google Scholar, EconLit, and AgEcon Search also comprise book chapters and grey literature such as conference papers, working papers, and reports in institutional series. Articles published in academic journals have usually passed a rigorous peer-review process. Most papers presented at academic conferences have also passed a peer-review process, which is often less strict than that of good journals though. Some of the other publications are peer reviewed, while many are not. Some of the working papers and reports are published by research institutes or government organizations, while others are NGO publications. Unlike previous reviews of GM crop impacts, we did not limit the sample to peer-reviewed studies but included all publications for two reasons. First, a clear-cut distinction between studies with and without peer review is not always possible, especially when dealing with papers that were not published in a journal or presented at an academic conference [24] . Second, studies without peer review also influence the public and policy debate on GM crops; ignoring them completely would be short-sighted.
Of the studies identified through the keyword searches, not all reported original impact results. We classified studies by screening titles, abstracts, and full texts. Studies had to fulfill the following criteria to be included:
- The study is an empirical investigation of the agronomic and/or economic impacts of GM soybean, GM maize, or GM cotton using micro-level data from individual plots and/or farms. Other GM crops such as GM rapeseed, GM sugarbeet, and GM papaya were commercialized in selected countries [23] , but the number of impact studies available for these other crops is very small.
- The study reports GM crop impacts in terms of one or more of the following outcome variables: yield, pesticide quantity (especially insecticides and herbicides), pesticide costs, total variable costs, gross margins, farmer profits. If only the number of pesticide sprays was reported, this was used as a proxy for pesticide quantity.
- The study analyzes the performance of GM crops by either reporting mean outcomes for GM and non-GM, absolute or percentage differences, or estimated coefficients of regression models that can be used to calculate percentage differences between GM and non-GM crops.
- The study contains original results and is not only a review of previous studies.
In some cases, the same results were reported in different publications; in these cases, only one of the publications was included to avoid double counting. On the other hand, several publications involve more than one impact observation, even for a single outcome variable, for instance when reporting results for different geographical regions or derived with different methods (e.g., comparison of mean outcomes of GM and non-GM crops plus regression model estimates). In those cases, all observations were included. Moreover, the same primary dataset was sometimes used for different publications without reporting identical results (e.g., analysis of different outcome variables, different waves of panel data, use of different methods). Hence, the number of impact observations in our sample is larger than the number of publications and primary datasets ( Data S1 ). The number of studies selected at various stages is shown in the flow diagram in Figure 1 . The number of publications finally included in the meta-analysis is 147 ( Table S1 ).
- PPT PowerPoint slide
- PNG larger image
- TIFF original image
https://doi.org/10.1371/journal.pone.0111629.g001
Effect sizes and influencing factors
Effect sizes are measures of outcome variables. We chose the percentage difference between GM and non-GM crops for five different outcome variables, namely yield, pesticide quantity, pesticide cost, total production cost, and farmer profits per unit area. Most studies that analyze production costs focus on variable costs, which are the costs primarily affected through GM technology adoption. Accordingly, profits are calculated as revenues minus variable production costs (profits calculated in this way are also referred to as gross margins). These production costs also take into account the higher prices charged by private companies for GM seeds. Hence, the percentage differences in profits considered here are net economic benefits for farmers using GM technology. Percentage differences, when not reported in the original studies, were calculated from mean value comparisons between GM and non-GM or from estimated regression coefficients.
Since we look at different types of GM technologies (different modified traits) that are used in different countries and regions, we do not expect that effect sizes are homogenous across studies. Hence, our approach of combining effect sizes corresponds to a random-effects model in meta-analysis [25] . To explain impact heterogeneity and test for possible biases, we also compiled data on a number of study descriptors that may influence the reported effect sizes. These influencing factors include information on the type of GM technology (modified trait), the region studied, the type of data and method used, the source of funding, and the type of publication. All influencing factors are defined as dummy variables. The exact definition of these dummy variables is given in Table 1 . Variable distributions of the study descriptors are shown in Table S2 .
https://doi.org/10.1371/journal.pone.0111629.t001
Statistical analysis
In a first step, we estimate average effect sizes for each outcome variable. To test whether these mean impacts are significantly different from zero, we regress each outcome variable on a constant with cluster correction of standard errors by primary dataset. Thus, the test for significance is valid also when observations from the same dataset are correlated. We estimate average effect sizes for all GM crops combined. However, we expect that the results may differ by modified trait, so that we also analyze mean effects for HT crops and IR crops separately.
Meta-analyses often weight impact estimates by their variances; estimates with low variance are considered more reliable and receive a higher weight [26] . In our case, several of the original studies do not report measures of variance, so that weighting by variance is not possible. Alternatively, weighting by sample size is common, but sample sizes are also not reported in all studies considered, especially not in some of the grey literature publications. To test the robustness of the results, we employ a different weighting procedure, using the inverse of the number of impact observations per dataset as weights. This procedure avoids that individual datasets that were used in several publications dominate the calculation of average effect sizes.
Results and Discussion
Average effect sizes.
Distributions of all five outcome variables are shown in Figure S1 . Table 2 presents unweighted mean impacts. As a robustness check, we weighted by the inverse of the number of impact observations per dataset. Comparing unweighted results ( Table 2 ) with weighted results ( Table S3 ) we find only very small differences. This comparison suggests that the unweighted results are robust.
https://doi.org/10.1371/journal.pone.0111629.t002
On average, GM technology has increased crop yields by 21% ( Figure 2 ). These yield increases are not due to higher genetic yield potential, but to more effective pest control and thus lower crop damage [27] . At the same time, GM crops have reduced pesticide quantity by 37% and pesticide cost by 39%. The effect on the cost of production is not significant. GM seeds are more expensive than non-GM seeds, but the additional seed costs are compensated through savings in chemical and mechanical pest control. Average profit gains for GM-adopting farmers are 69%.
Average percentage differences between GM and non-GM crops are shown. Results refer to all GM crops, including herbicide-tolerant and insect-resistant traits. The number of observations varies by outcome variable; yield: 451; pesticide quantity: 121; pesticide cost: 193; total production cost: 115; farmer profit: 136. *** indicates statistical significance at the 1% level.
https://doi.org/10.1371/journal.pone.0111629.g002
Results of Cochran’s test [25] , which are reported in Figure S1 , confirm that there is significant heterogeneity across study observations for all five outcome variables. Hence it is useful to further disaggregate the results. Table 2 shows a breakdown by modified crop trait. While significant reductions in pesticide costs are observed for both HT and IR crops, only IR crops cause a consistent reduction in pesticide quantity. Such disparities are expected, because the two technologies are quite different. IR crops protect themselves against certain insect pests, so that spraying can be reduced. HT crops, on the other hand, are not protected against pests but against a broad-spectrum chemical herbicide (mostly glyphosate), use of which facilitates weed control. While HT crops have reduced herbicide quantity in some situations, they have contributed to increases in the use of broad-spectrum herbicides elsewhere [2] , [11] , [19] . The savings in pesticide costs for HT crops in spite of higher quantities can be explained by the fact that broad-spectrum herbicides are often much cheaper than the selective herbicides that were used before. The average farmer profit effect for HT crops is large and positive, but not statistically significant because of considerable variation and a relatively small number of observations for this outcome variable.
Impact heterogeneity and possible biases
Table 3 shows the estimation results from the meta-regressions that explain how different factors influence impact heterogeneity. Controlling for other factors, yield gains of IR crops are almost 7 percentage points higher than those of HT crops (column 1). Furthermore, yield gains of GM crops are 14 percentage points higher in developing countries than in developed countries. Especially smallholder farmers in the tropics and subtropics suffer from considerable pest damage that can be reduced through GM crop adoption [27] .
https://doi.org/10.1371/journal.pone.0111629.t003
Most original studies in this meta-analysis build on farm surveys, although some are based on field-trial data. Field-trial results are often criticized to overestimate impacts, because farmers may not be able to replicate experimental conditions. However, results in Table 3 (column 1) show that field-trial data do not overestimate the yield effects of GM crops. Reported yield gains from field trials are even lower than those from farm surveys. This is plausible, because pest damage in non-GM crops is often more severe in farmers’ fields than on well-managed experimental plots.
Another concern often voiced in the public debate is that studies funded by industry money might report inflated benefits. Our results show that the source of funding does not significantly influence the impact estimates. We also analyzed whether the statistical method plays a role. Many of the earlier studies just compared yields of GM and non-GM crops without considering possible differences in other inputs and conditions that may also affect the outcome. Net impacts of GM technology can be estimated with regression-based production function models that control for other factors. Interestingly, results derived from regression analysis report higher average yield effects.
Finally, we examined whether the type of publication matters. Controlling for other factors, the regression coefficient for journal publications in column (1) of Table 3 implies that studies published in peer-reviewed journals show 12 percentage points higher yield gains than studies published elsewhere. Indeed, when only including observations from studies that were published in journals, the mean effect size is larger than if all observations are included ( Figure S2 ). On first sight, one might suspect publication bias, meaning that only studies that report substantial effects are accepted for publication in a journal. A common way to assess possible publication bias in meta-analysis is through funnel plots [25] , which we show in Figure S3 . However, in our case these funnel plots should not be over-interpreted. First, only studies that report variance measures can be included in the funnel plots, which holds true only for a subset of the original studies used here. Second, even if there were publication bias, our mean results would be estimated correctly, because we do include studies that were not published in peer-reviewed journals.
Further analysis suggests that the journal review process does not systematically filter out studies with small effect sizes. The journal articles in the sample report a wide range of yield effects, even including negative estimates in some cases. Moreover, when combining journal articles with papers presented at academic conferences, average yield gains are even higher ( Table 3 , column 2). Studies that were neither published in a journal nor presented at an academic conference encompass a diverse set of papers, including reports by NGOs and outspoken biotechnology critics. These reports show lower GM yield effects on average, but not all meet common scientific standards. Hence, rather than indicating publication bias, the positive and significant journal coefficient may be the result of a negative NGO bias in some of the grey literature.
Concerning other outcome variables, IR crops have much stronger reducing effects on pesticide quantity than HT crops ( Table 3 , column 3), as already discussed above. In terms of pesticide costs, the difference between IR and HT is less pronounced and not statistically significant (column 4). The profit gains of GM crops are 60 percentage points higher in developing countries than in developed countries (column 6). This large difference is due to higher GM yield gains and stronger pesticide cost savings in developing countries. Moreover, most GM crops are not patented in developing countries, so that GM seed prices are lower [19] . Like for yields, studies published in peer-reviewed journals report higher profit gains than studies published elsewhere, but again we do not find evidence of publication bias (column 7).
This meta-analysis confirms that – in spite of impact heterogeneity – the average agronomic and economic benefits of GM crops are large and significant. Impacts vary especially by modified crop trait and geographic region. Yield gains and pesticide reductions are larger for IR crops than for HT crops. Yield and farmer profit gains are higher in developing countries than in developed countries. Recent impact studies used better data and methods than earlier studies, but these improvements in study design did not reduce the estimates of GM crop advantages. Rather, NGO reports and other publications without scientific peer review seem to bias the impact estimates downward. But even with such biased estimates included, mean effects remain sizeable.
One limitation is that not all of the original studies included in this meta-analysis reported sample sizes and measures of variance. This is not untypical for analyses in the social sciences, especially when studies from the grey literature are also included. Future impact studies with primary data should follow more standardized reporting procedures. Nevertheless, our findings reveal that there is robust evidence of GM crop benefits. Such evidence may help to gradually increase public trust in this promising technology.
Supporting Information
Histograms of effect sizes for the five outcome variables.
https://doi.org/10.1371/journal.pone.0111629.s001
Impacts of GM crop adoption including only studies published in journals.
https://doi.org/10.1371/journal.pone.0111629.s002
Funnel plots for the five outcome variables.
https://doi.org/10.1371/journal.pone.0111629.s003
List of publications included in the meta-analysis.
https://doi.org/10.1371/journal.pone.0111629.s004
Distribution of study descriptor dummy variables for different outcomes.
https://doi.org/10.1371/journal.pone.0111629.s005
Weighted mean impacts of GM crop adoption.
https://doi.org/10.1371/journal.pone.0111629.s006
Data used for the meta-analysis.
https://doi.org/10.1371/journal.pone.0111629.s007
Acknowledgments
We thank Sinja Buri and Tingting Xu for assistance in compiling the dataset. We also thank Joachim von Braun and three reviewers of this journal for useful comments.
Author Contributions
Conceived and designed the research: WK MQ. Analyzed the data: WK MQ. Contributed to the writing of the manuscript: WK MQ. Compiled the data: WK.
- View Article
- Google Scholar
- 2. Fernandez-Cornejo J, Wechsler JJ, Livingston M, Mitchell L (2014) Genetically Engineered Crops in the United States. Economic Research Report ERR-162 (United Sates Department of Agriculture, Washington, DC).
- 6. European Academies Science Advisory Council (2013) Planting the Future: Opportunities and Challenges for Using Crop Genetic Improvement Technologies for Sustainable Agriculture (EASAC, Halle, Germany).
- 7. European Commission (2010) A Decade of EU-Funded GMO Research 2001–2010 (European Commission, Brussels).
- 12. Sexton S, Zilberman D (2012) Land for food and fuel production: the role of agricultural biotechnology. In: The Intended and Unintended Effects of US Agricultural and Biotechnology Policies (eds. Zivin, G. & Perloff, J.M.), 269–288 (University of Chicago Press, Chicago).
- 13. Ali A, Abdulai A (2010) The adoption of genetically modified cotton and poverty reduction in Pakistan. Journal of Agricultural Economics 61, 175–192.
- 17. Smale M, Zambrano P, Gruere G, Falck-Zepeda J, Matuschke I, et al.. (2009) Measuring the Economic Impacts of Transgenic Crops in Developing Agriculture During the First Decade: Approaches, Findings, and Future Directions (International Food Policy Research Institute, Washington, DC).
- 23. James C (2013) Global Status of Commercialized Biotech/GM Crops: 2013. ISAAA Briefs No.46 (International Service for the Acquisition of Agri-biotech Applications, Ithaca, NY).
- 24. Rothstein HR, Hopewell S (2009) Grey literature. In: Handbook of Research Synthesis and Meta-Analysis, Second Edition (eds. Cooper, H., Hegdes, L.V. & Valentine, J.C.), 103–125 (Russell Sage Foundation, New York).
- 25. Borenstein M, Hedges LV, Higgins JPT, Rothstein HR (2009) Introduction to Meta-Analysis (John Wiley and Sons, Chichester, UK).
- 26. Shadish WR, Haddock CK (2009) Combining estimates of effect size. In: Handbook of Research Synthesis and Meta-Analysis, Second Edition (eds. Cooper, H., Hegdes, L.V. & Valentine, J.C.), 257–277 (Russell Sage Foundation, New York).
- Open access
- Published: 13 January 2022
Evaluation of adverse effects/events of genetically modified food consumption: a systematic review of animal and human studies
- Chen Shen 1 ,
- Xiang-Chang Yin 2 ,
- Bo-Yang Jiao 3 ,
- Jing Li 4 ,
- Peng Jia 5 ,
- Xiao-Wen Zhang 1 ,
- Xue-Hao Cheng 6 ,
- Jian-Xin Ren 6 ,
- Hui-Di Lan 7 ,
- Wen-Bin Hou 1 ,
- Min Fang 1 ,
- Yu-Tong Fei 1 ,
- Nicola Robinson 1 , 8 &
- Jian-Ping Liu ORCID: orcid.org/0000-0002-0320-061X 1 , 9
Environmental Sciences Europe volume 34 , Article number: 8 ( 2022 ) Cite this article
45k Accesses
15 Citations
67 Altmetric
Metrics details
A systematic review of animal and human studies was conducted on genetically modified (GM) food consumption to assess its safety in terms of adverse effects/events to inform public concerns and future research.
Seven electronic databases were searched from January 1st 1983 till July 11th 2020 for in vivo, animal and human studies on the incidence of adverse effects/events of GM products consumption. Two authors independently identified eligible studies, assessed the study quality, and extracted data on the name of the periodical, author and affiliation, literature type, the theme of the study, publication year, funding, sample size, target population characteristics, type of the intervention/exposure, outcomes and outcome measures, and details of adverse effects/events. We used the Chi-square test to compare the adverse event reporting rates in articles funded by industry funding, government funding or unfunded articles.
One crossover trial in humans and 203 animal studies from 179 articles met the inclusion criteria. The study quality was all assessed as being unclear or having a high risk of bias. Minor illnesses were reported in the human trial. Among the 204 studies, 59.46% of adverse events (22 of 37) were serious adverse events from 16 animal studies (7.84%). No significant differences were found in the adverse event reporting rates either between industry and government funding ( χ 2 = 2.286, P = 0.131), industry and non-industry funding ( χ 2 = 1.761, P = 0.185) or funded and non-funded articles ( χ 2 = 0.491, P = 0.483). We finally identified 21 GM food-related adverse events involving 7 GM events (NK603 × MON810 maize, GTS 40-3-2 soybean, NK603 maize, MON863 maize, MON810 maize, MON863 × MON810 × NK603 maize and GM Shanyou 63 rice), which had all been on regulatory approval in some countries/regions.
Serious adverse events of GM consumption include mortality, tumour or cancer, significant low fertility, decreased learning and reaction abilities, and some organ abnormalities. Further clinical trials and long-term cohort studies in human populations, especially on GM food-related adverse events and the corresponding GM events, are still warranted. It suggests the necessity of labelling GM food so that consumers can make their own choice.
Introduction
Genetic modification is defined as introducing transgene(s) with desired traits into the recipient organism’s genome by recombinant deoxyribonucleic acid (DNA) technology, and therefore it does not occur naturally [ 1 , 2 , 3 ]. Genetically modified (GM) crops are thought to address food security, sustainability and climate change solutions by improving crop yields, conserving biodiversity, providing a better environment in terms of the insect-resistant and herbicide-tolerant traits, reducing CO 2 emissions and helping alleviate poverty through uplifting the economic situation [ 4 ]. Insect-resistant and herbicide-tolerant traits were first introduced into four types of crop, canola, cotton, maize and soybeans, at the beginning of GM production [ 5 ]. At present, the mainstream characteristics of new crops still pursue higher-yielding, more nutritious, pest- and disease-resistant and climate-smart to meet future demand for a yield increase of major crops such as wheat, rice and corn, due to the growing population [ 6 ].
Since 1996, the first year of commercialization of GM crops, 70 countries had adopted GM crops until 2018, including 26 countries that cumulatively planted 2.5 billion hectares of GM crops and an additional 44 countries that imported GM crops. During the 27 years (1992 to 2018), 4349 approvals for 387 GM events from 27 GM crops were granted by 70 countries involving 2063 for food (when the direct consumers are mainly humans), 1461 for feed (the products only intended for animal consumption) use and 825 for environmental release or cultivation [ 4 , 7 ]. The major agricultural product exporting countries like the U.S.A., Brazil and Argentina show over 90% adoption of biotech crops [ 4 ]. For GM animal products, biotech salmon, considered to be the first genetically engineered animal for human consumption, was approved by the United States Department of Agriculture and Food & Drug Administration in 2015 [ 8 ]. In addition, it is illegal to grow major GM food crops in China while there are substantial investments in biotechnology research and GM maize, soybeans, and canola are allowed to import and eat [ 9 ].
Genetically modified food, however, is an example of the controversial relation between the inherent uncertainty of the scientific approach and the need of consumers to use products resulting from scientific developments thought to be safe [ 10 ]. Significant health risks have not been reported in peer-reviewed studies on GM food safety/security, which may cause some publication bias [ 11 ] but with a few exceptions, like the most famous “Monarch Butterfly controversy” [ 12 ], "Pusztai case" [ 13 ] and the "Séralini case" [ 14 ]. Unexpected effects of GM crops were reported in these studies, occupying an important place in the pages of scientific journals. Nevertheless, the above controversies severely impacted the public image, leading to full or partial bans in 38 countries including the European Union [ 15 ].
The complexity of risk evaluation is shown in these conflicting results, and concerns about the citizen-consumers have been raised against GM food [ 10 ]. Of most concern, aroused from the controversial events and some research results, is the potential of carcinogenesis, teratogenesis [ 16 ], lethal effects and adverse influences on fertility. GM agriculture is now widely discussed in both positive and negative frames and currently serves as a hotbed of debate in the public and policymakers. Although there are some reports and evidence from human and animal studies on the potential health effects of GM food/feed, the evidence is not conclusive and public concerns have not been resolved.
We aimed to conduct a systematic review of animal and human studies on GM food consumption to assess its safety in terms of adverse effects/events to inform public concerns and future research.
This study was a systematic review of previously published studies, conducted and reported in adherence with the Preferred Reporting Items for Systematic Reviews and Meta-Analyses (PRISMA) [ 17 ] guideline.
Search strategy
China National Knowledge Infrastructure (CNKI), Wanfang, VIP Database, Chinese Biomedical Database (SinoMed), PubMed, the Cochrane Library and Embase databases were searched from January, 1st, 1983 till July, 11th, 2020, using a predefined search strategy (Additional file 1 : Appendix S1). Reference lists of retrieved articles were also searched.
Eligibility criteria
Based on the evidence pyramid proposed by the Medical Center of State University of New York in 2001, we determined the type of research we included in the study. For a comprehensive evaluation of the literature, all in vivo animal studies and human studies (cross-sectional studies, case reports, case series, case–control studies, case–crossover studies, cohort studies, controlled clinical trials, including randomized trials, quasi-randomized trials and non-randomized trials) in multiple languages were included. Animal studies in all fields were included, that is, they could be clinical, agricultural and animal husbandry, veterinary medicine, life sciences, etc. Field studies were excluded.
The study population in animal studies was applied with inclusion criteria based on the categorization approach that highlights the actual use of them: laboratory animals and economical animals (livestock and aquatilia) were included, with no prespecified limitations on age, population, species/races, health status or others. Interventions/exposures of the genetically modified animal/plant/microorganism products included for animal/human ingestion referred to GM food, GM food ingredients and GM feed, regardless of their dosage or duration. The GM strain (line) and GM event were not limited. There was no restriction on whether controls were or were not included. The studies were excluded if they focused on the effects of GM food/feed on secondary or multilevel consumers in the food chain where GM food/feed was only consumed by primary consumers in the predator relationships. For instance, if non-GM fishes were fed with diet containing GM ingredients and then the fish was fed to the experimental cats, the study was excluded.
Outcomes focused on the incidence of adverse effects or adverse events in GM food/feed consumption, including primary outcomes on carcinogenesis, teratogenesis, lethal effect (all-cause mortality) and reproduction and secondary outcomes on other biomarkers were included. Toxicity studies of general toxicity studies (acute, sub-acute, sub-chronic, chronic and carcinogenicity toxicity studies) and specific toxicity studies (genotoxicity, reproductive and developmental toxicity, immunotoxicity and other toxicology studies) were included. Mortality in pups before weaning was considered as an outcome of reproductive toxicity but not as a lethal effect. Outcomes of adverse events in laboratory testing would not be included only when they could indicate tissue or organ toxicity. Outcomes of adverse events in breeding performance in animal husbandry studies, which focused on the economic benefits of the animal products, were included and these indicators were regarded as reproduction biomarkers in this research.
Outcomes of adverse events on growth performance, carcass traits, meat and fur production performance and meat quality for economic benefit evaluation of live stocks were excluded, of which the indicators included final body weight, weight gain, feed to gain ratio, half-eviscerated weight, eviscerated weight, percentage of eviscerated yield and muscle lean meat, sebum rate in some parts of the body, etc. Studies on the insecticidal effect of insect-resistant GM feed and outcomes of adverse events in gene fragments residual in the digestive tract were excluded. Besides, duplicate publications, studies with duplicate statistics, or references devoid of necessary information of participants, sample size, interventions/exposures or results were excluded.
Study selection and data extraction
Titles and abstracts of the retrieved articles were reviewed by 6 researchers in pair (C Shen, XC Yin, BY Jiao, J Peng, YZ Li, XH Cheng). 6 authors (C Shen, XC Yin, BY Jiao, JX Ren, J Li and XW Zhang) independently reviewed the full texts to identify the studies meeting eligibility criteria and then 8 researchers in pair (C Shen, XC Yin, BY Jiao, J Li, P Jia, XW Zhang, XH Cheng and JX Ren) independently extracted data from the included studies according to a predesignated extraction table. The discrepancies were resolved through consensus and if necessary, arbitrated by another author (JP Liu).
We extracted the name of the periodical, author and affiliation, literature type, the theme of the study, publication year, funding, sample size, target population characteristics, type of the intervention/exposure, outcomes and outcome measures. For those studies in which adverse effects/events occurred, details of interventions/exposures and control conditions (if any), dosage, duration, number of the generation, and the results were extracted.
Quality assessment
The methodological quality for animal studies was assessed, using criteria from the SYRCLE’s risk of bias tool for animal studies. The quality of animal studies was categorized into low risk of bias, unclear risk of bias, or high risk of bias according to the risk for each important outcome within included studies, including the adequacy of generation of the sequence generation, baseline characteristics, allocation concealment, random housing, blinding (performance bias), random outcome assessment, blinding (detection bias), incomplete outcome data, selective outcome reporting, or other sources of bias. The judgment of other risk of bias was based on whether there were contamination (pooling drugs), inappropriate influence of funders, unit of analysis errors, design-specific risks of bias or new animals added to the control and experimental groups to replace drop-outs from the original population.
Statistical synthesis and analyses
Statistical analyses were carried out using Microsoft Excel 2016 and SPSS 20.0. The findings were reported mainly in two parts, characteristics of the included studies and detailed information on the studies in which adverse effects/events occurred. Initially, descriptive statistics, frequencies, and percentages were calculated to summarize the data. Subsequently, studies that evaluated similar populations, interventions, controls (if any) and outcomes were pooled using a random-effects meta-analysis, and data from other studies were presented in tables and described in a narrative summary. The incidence of adverse events reported in articles funded by industry funding, government funding or unfunded articles were, respectively, counted and the Chi-square test was used for the comparisons.
Besides, we figured the incidence of serious adverse events (SAEs) by percentage. With reference to the Food and Drug Administration’s definition [ 18 ], our study defined SAEs as death, life-threatening, hospitalization (initial or prolonged), disability or permanent change, disruption, impairment or damage in a body function or structure (including cancer or tumour), in physical activities or quality of life, congenital anomaly or birth defect in the newborn child or pups, infertility or significant low in the number of deliveries or live birth rate than the non-GM commercial, conventional or blank controls, and an event resulting in intervention/treatment to prevent permanent impairment, damage or to prevent one of the other outcomes.
Meanwhile, the adverse events which cannot be ruled out that it has nothing to do with GM food (hereinafter abbreviated as GM food-related adverse events) were identified and the percentages under each outcome were calculated.
Description of studies
The flow diagram of the literature selection is shown in Fig. 1 . A total of 9668 records were identified, including 9584 from the initial search through seven databases and 84 from other sources. After removal of duplicates and exclusion of references by reading titles and abstracts, 455 full-text articles were screened and 276 references were excluded with reasons (seen in the flow chart). Finally, 204 studies from 179 articles [ 19 , 20 , 21 , 22 , 23 , 24 , 25 , 26 , 27 , 28 , 29 , 30 , 31 , 32 , 33 , 34 , 35 , 36 , 37 , 38 , 39 , 40 , 41 , 42 , 43 , 44 , 45 , 46 , 47 , 48 , 49 , 50 , 51 , 52 , 53 , 54 , 55 , 56 , 57 , 58 , 59 , 60 , 61 , 62 , 63 , 64 , 65 , 66 , 67 , 68 , 69 , 70 , 71 , 72 , 73 , 74 , 75 , 76 , 77 , 78 , 79 , 80 , 81 , 82 , 83 , 84 , 85 , 86 , 87 , 88 , 89 , 90 , 91 , 92 , 93 , 94 , 95 , 96 , 97 , 98 , 99 , 100 , 101 , 102 , 103 , 104 , 105 , 106 , 107 , 108 , 109 , 110 , 111 , 112 , 113 , 114 , 115 , 116 , 117 , 118 , 119 , 120 , 121 , 122 , 123 , 124 , 125 , 126 , 127 , 128 , 129 , 130 , 131 , 132 , 133 , 134 , 135 , 136 , 137 , 138 , 139 , 140 , 141 , 142 , 143 , 144 , 145 , 146 , 147 , 148 , 149 , 150 , 151 , 152 , 153 , 154 , 155 , 156 , 157 , 158 , 159 , 160 , 161 , 162 , 163 , 164 , 165 , 166 , 167 , 168 , 169 , 170 , 171 , 172 , 173 , 174 , 175 , 176 , 177 , 178 , 179 , 180 , 181 , 182 , 183 , 184 , 185 , 186 , 187 , 188 , 189 , 190 , 191 , 192 , 193 , 194 , 195 , 196 , 197 ] (153 journal articles, 22 dissertations, 3 conference proceedings and 1 unpublished report) were included in data synthesis, since there were more than one study conducted in each of the 2 included dissertations [ 107 , 127 ], 11 journal articles [ 19 , 33 , 35 , 63 , 67 , 88 , 102 , 118 , 132 , 172 , 184 ] and 1 unpublished report [ 32 ]. The included studies were of 203 in vivo animal studies and 1 crossover trial [ 97 ] in humans.

The flow of literature search and selection of studies on the safety of GM food
Study characteristics
Of the 179 included articles, 94 were in English [ 19 , 20 , 21 , 22 , 23 , 24 , 25 , 26 , 27 , 28 , 29 , 30 , 31 , 32 , 33 , 34 , 35 , 36 , 37 , 38 , 39 , 40 , 41 , 42 , 43 , 44 , 45 , 46 , 47 , 48 , 49 , 50 , 51 , 52 , 53 , 54 , 55 , 56 , 57 , 58 , 59 , 60 , 61 , 62 , 63 , 64 , 65 , 66 , 67 , 68 , 69 , 70 , 71 , 72 , 73 , 74 , 75 , 76 , 77 , 78 , 79 , 80 , 81 , 82 , 83 , 84 , 85 , 86 , 87 , 88 , 89 , 90 , 91 , 92 , 93 , 94 , 95 , 96 , 97 , 98 , 99 , 100 , 101 , 102 , 103 , 104 , 105 , 106 , 107 , 108 , 109 , 110 , 111 , 112 ], 83 were published in Chinese [ 113 , 114 , 115 , 116 , 117 , 118 , 119 , 120 , 121 , 122 , 123 , 124 , 125 , 126 , 127 , 128 , 129 , 130 , 131 , 132 , 133 , 134 , 135 , 136 , 137 , 138 , 139 , 140 , 141 , 142 , 143 , 144 , 145 , 146 , 147 , 148 , 149 , 150 , 151 , 152 , 153 , 154 , 155 , 156 , 157 , 158 , 159 , 160 , 161 , 162 , 163 , 164 , 165 , 166 , 167 , 168 , 169 , 170 , 171 , 172 , 173 , 174 , 175 , 176 , 177 , 178 , 179 , 180 , 181 , 182 , 183 , 184 , 185 , 186 , 187 , 188 , 189 , 190 , 191 , 192 , 193 , 194 , 195 ], and 2 in Japanese [ 196 , 197 ]. The earliest included reference dated back to 1998 [ 153 ] (shown in Fig. 2 ), after which the remaining articles were distributed from 2000 to 2020 (45 articles in the 2000s, while 131 in the 2010s and 2 in the 2020s). The year 2012 witnessed the largest volume of publication (n = 26 articles, 14.53%). For funding sources or sponsors (Additional file 1 : Appendix S2), in addition to 57 articles not mentioning the funding/sponsor (hereinafter as non-funded articles), there were 116 articles (64.8% of the 179 articles) supported by 56 kinds of government funding from 12 countries/government organizations and, still, 9 articles (5.03%) by 10 kinds of industry/institute funding sources/sponsors from 4 countries (America, Australia, French and German). Among them, 3 articles [ 29 , 62 , 74 ] claimed to have been funded or sponsored by both government and industry. China had undertaken the most government/school-level funding projects (39 of 56 projects, 69.64%).
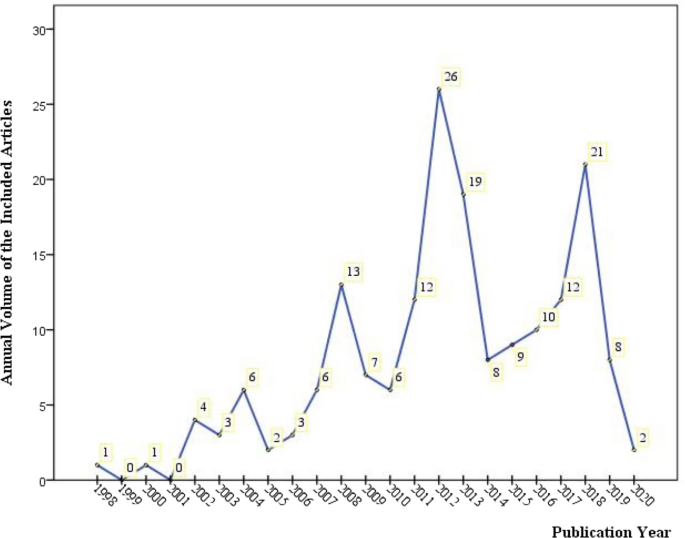
The publications (number of articles) on the safety of GM food by year
The periodicals that have published more than 5 included articles were Food and Chemical Toxicology (published 25 included articles), EFSA Journal (13), Regulatory Toxicology and Pharmacology (9), Journal of Hygiene Research (9) and Chinese Journal of Food Hygiene (8). 11 of 13 authors, who have published ten or more included studies, were from European Food Safety Authority and published 12 included articles as co-authors. They were Christina Tlustos (published 12 included articles), Claudia Bolognesi (12), Konrad Grob (12), Vittorio Silano (12), Andre Penninks (11), Gilles Riviere (11), Holger Zorn (11), Karl-Heinz Engel (11), Yi Liu (11), Natalia Kovalkovicova (10), Sirpa Karenlampi (10). In addition to the above 12 articles, the top 3 of the 11 authors who published five or more included studies was Yang Xiao-Guang (from Chinese Center for Disease Control and Prevention, published 11 included articles), Wang Jing (from Tianjin Centre for Disease Control and Prevention, published 10 included articles) and Zhuo Qin (from Chinese Center for Disease Control and Prevention, published 7 included articles). The top 5 affiliations which published included articles were Chinese Center for Disease Control and Prevention (published 16 included articles), Tianjin Centre for Disease Control and Prevention (12), European Food Safety Authority (12), National Chung Hsing University (10), International Rice Research Institute (9).
Of the 204 included studies, one was a double-blind crossover trial ( n = 36) in humans and the others were all animal studies. Individual sample sizes of the total 54,392 study population ranged from 4 (cats) [ 153 ] to 21,000 (Atlantic salmon) [ 23 ]. The studies involved 14 different kinds of animals (see Table 1 ). Apart from the most commonly used rats/mice (in 160 studies, 78.82%), pigs and chicks were two of the most extensively studied animals (in 23 studies, 11.33%). For themes of the 178 included animal studies, 158 were on clinical and 20 were on agricultural and animal husbandry. For the ones on clinical, 117 were on general toxicity (8 on acute, 6 sub-acute, 84 sub-chronic, 16 chronic toxicity, and still 3 on both acute, sub-acute and sub-chronic toxicity), 35 on specific toxicity (15 on reproductive and developmental toxicity, 16 on immunotoxicity, 3 on teratogenic effect and 1 on mutagenicity), 3 on allergenicity, 1 on learning and memory ability, 1 on athletic ability and 1 on both sub-chronic toxicity and allergenicity.
For interventions/exposures, 31 kinds of GM food were identified, including 18 kinds of GM plant food, 7 kinds of GM animal food and 6 kinds of GM microorganism food. Each included study covered one intervention/exposure, except for one study, Chen [ 29 ], that involved two kinds of GM products (sweet pepper and tomato) modified with the same gene (coat protein gene of cucumber mosaic virus), respectively, in two experimental groups. Maize, rice and soybean were the three most popular kinds of GM plant food (taken 79.38%) in research while milk/milk powder and animal-derived protein occupied the top two in GM animal food (56.25%). As for GM microorganism products, 5 kinds of food/feed enzyme derived from 5 different kinds of GM fungi or bacteria as well as 1 kind of microorganism-derived protein were among included studies.
Methodological quality of the animal studies
According to our predefined quality assessment criteria, all of the studies were identified as being unclear or having a high risk of bias (Fig. 3 ). None of the studies were reported to blind researchers from knowing which intervention each animal received. None of the studies reported prior sample-size calculation, 31 studies (15.27%) described wrong randomization procedures or did not mention the method of “randomization”, and 12 studies (5.91%) did not report adequate allocation concealment. 28 studies (13.79%) described that the groups were similar at baseline and 76 studies (37.44%) claimed that the housing conditions of animals from the various experimental groups were identical. 10 studies (4.93%) described randomly pick an animal during outcome assessment while 7 studies (3.45%) failed to select animals at random for outcome assessment. 88 studies (43.35%) completely used objective outcome indicators for outcome measurement. 185 studies (91.13%) reported consistent outcomes in the method and result sections while 5 studies did not, but none of the study protocols were available.
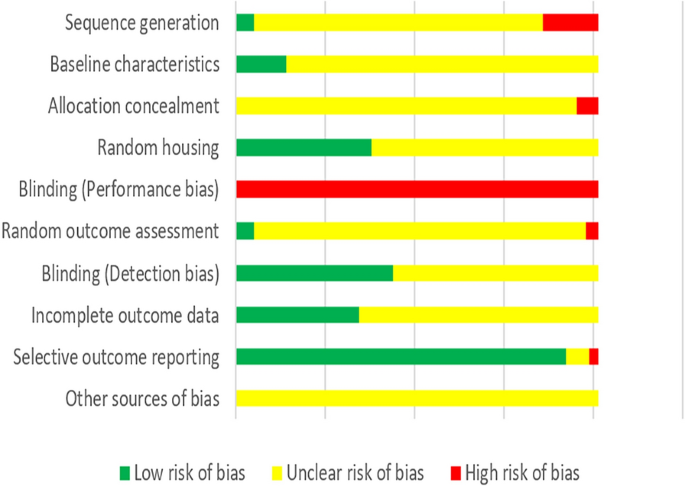
Risk of bias of the included animal studies
Incidence of adverse events/effects
No meta-analysis was conducted due to the significant heterogeneity of the primary studies. Among the 204 studies, a total of 29 studies (14.22%) from 23 articles reported 37 adverse events, involving 13 on mortality, 6 on reproductive toxicity, 3 on carcinogenesis and 15 on other biomarkers (including one human trial). It is worth noting that when, in one study, there were multiple aspects of adverse events on “other biomarkers”, we recorded it as 1 adverse event. Then, 22 serious adverse events (59.46% of adverse events) were identified in 16 studies (7.84% of the included studies and 55.17% of the studies reporting adverse events, marked in the tables with double asterisks). The SAEs mainly rested on mortality (13 studies), tumour or cancer (3), significant low in the number of pup deliveries (2), decreased learning and reaction abilities (1), severe stomach inflammation (1), intestinal adenoma lesions (1), and other pathology abnormalities (1) as hypertrophies and hyperplasia in mammary glands and pituitary, liver congestions and necrosis as well as severe chronic progressive nephropathies.
The incidence of adverse events reporting in government funding, industry funding and non-funded articles were 10.34% (12 of 116), 33.33% (3 of 9) and 15.79% (9 of 57), respectively. When comparing the adverse event reporting rates using the Chi-square test, we found that there were no significant differences either between industry funding and government funding ( χ 2 = 2.286, P = 0.131), industry funding and non-industry funding ( χ 2 = 1.761, P = 0.185) or funded and non-funded articles ( χ 2 = 0.491, P = 0.483).
Incidence of adverse events/effects in human trial
As for the human trial [ 97 ], shown in Table 2 , a randomized double-blind crossover design was conducted for acute consumption of two single breakfasts, with a 14-day washout period, containing either seed oil generated from transgenic Camelina sativa plants or commercially blended fish oil. 36 healthy people were randomly allocated into two groups and venous blood samples were collected after the postprandial session, 8 h after each meal. No follow-up was reported. No major adverse symptoms or health effects were reported but some unrelated minor illnesses for the 72 postprandial sessions from 36 participants, such as minor upper respiratory tract infections (2.78%), minor nose bleed (1.39%), pyelonephritis (1.39%) and headaches (8.33%).
Incidence of adverse events/effects in animal studies
For the 203 animal studies, 28 studies (13.79%) from 22 articles reported 36 adverse events, including 13 on mortality (Table 3 , 36.11%), 6 on reproductive toxicity ( Table 4 , 16.67%), 3 on carcinogenesis (Table 5 , 8.33%) and 14 on other biomarkers (Additional file 1 : Appendix S3, 38.89%).
All causes of death were included in this analysis and 11 of the 13 studies claimed that the mortality was not significantly different between the groups or had nothing to do with GM food. One study (Ermakova [ 37 ]) reported higher pup mortality in the Roundup-Ready soya (40.3.2 line) group compared with the controls. In Séralini [ 74 ] , the general cause of death was large mammary tumours in females and other organ problems in males. Besides, rats in the Roundup-tolerant GM NK603 maize groups were 2–3 times more likely to die than controls, and more rapidly.
With respect to effects on reproduction, 5 animal feeding studies were reported to trigger reproductive toxicity but one study (Cisterna [ 31 ]) claimed to have no substantial impact on fertility. The reproductive toxicity manifested in the significant low in the number of deliveries, survival rate (from birth to weaning), litter weight, litter size and weight of some organs in the pups. For example, in Ermakova I 2005, the rats fed with Roundup-Ready soya had a 55.6% pup mortality rate during lactation periods compared to 9% in the control of traditional soya and 6.8% in the reference group. The pups kept dying during the lactation period while pups from the control group only died during the first week. Cyran N 2008 a and Cyran N 2008 c [ 32 ] were two rat feeding studies reported in one article, both given NK603 × MON810 maize. A multi-generation study was conducted as Cyran N 2008 a while Cyran N 2008 c did a continuous breeding study. Both of them indicated that fewer sum of pups was born and weaned in the GM groups. Pup losses, in Cyran N 2008 a, overall generations were about twice as many pups lost as compared to the control group (14.59% vs 7.4%) but was not significantly different and significantly lower litter weight was also reported in Cyran N 2008 c.
Three mouse/rat feeding studies reported triggering cancers/tumours when Tang [ 156 ] attributed the incidence of the tumour to the elder age of rats. Séralini 2014 (on Roundup-tolerant GM maize) found that females in the treatment groups almost always developed large mammary tumours more often than and controls. As for males, 4 times larger palpable tumours than controls were presented which emerged up to 600 days earlier. Cyran 2008 b [ 32 ] revealed a life term study where mice in the three groups were fed with transgenic maize NK603xMON810 (from 33.0% in the diet), control isoline diet and GM-free Austrian corn reference diet, respectively. The survival rate was not significantly different while cancer (leucosis) was the common cause of death.
GM food-related adverse events
Among the 37 adverse events reported, 16 of them claimed to have nothing to do with GM food, while the rest 21 (from 17 studies) did not, still leaving the question open. The GM food-related adverse events existed in mortality (2 studies), reproductive toxicity (5), carcinogenesis (2), and other biomarkers (12).
By gathering evidence, we identified 3 kinds of GM food associated with adverse events, GM soybean, GM maize as well as GM rice. For the 17 studies involved in the GM food-related adverse events, 4 studies were absent of information on the GM event of their test substance and the remainder concentrated on 7 GM events (3 studies on NK603 × MON810 maize, 2 on GTS 40-3-2 soybean, 2 on NK603 maize, 2 on MON863 maize, 2 on MON810 maize, 1 on maize mixed with MON863 × MON810 × NK603, NK603 × MON810 and NK603 and 1 on GM Shanyou 63 rice). When searching in the GM Approval Database on the ISAAA website, we found that all of the first 6 GM events listed, all developed by Monsanto Company, had been on regulatory approval for food, feed and cultivation in multiple countries/regions, including the European Union. GM -39 Shanyou 63 was developed in China and given approval for food, feed, and cultivation only by China in 2009.
Summary of findings
We included 203 in vivo animal studies and 1 human trial, and all of the studies were identified as being unclear or having a high risk of bias. Overall, we reported two main findings. First, we identified 37 adverse events for GM food consumption while 22 of them (59.46%) were serious adverse events extracted from 16 animal studies (7.84%). SAEs were mortality, tumour or cancer, significantly low in the number of pup deliveries, decreased learning and reaction abilities, severe stomach inflammation, intestinal adenoma lesions, and other pathological abnormalities in the mammary glands, pituitary, liver and kidney.
Second, there were 21 GM food-related adverse events indicating that GM food may have effects on increased mortality (2 studies), reproductive toxicity (5 studies), which referred to significantly low fertility in parental generation and low survival rate, litter weight, litter size and weight of some organs in the pups, carcinogenesis (2 studies) and other biomarkers (12 studies). The effect-related GM food included 7 GM events (NK603 × MON810 maize, GTS 40-3-2 soybean, NK603 maize, MON863 maize, MON810 maize, MON863 × MON810 × NK603 maize and GM Shanyou 63 rice), which had all been on regulatory approval for food, feed and cultivation in some countries/regions.
Agreements and disagreements with other reviews
To our knowledge, there have been 3 previous systematic reviews (SRs) [ 198 , 199 , 200 ] and 6 conventional reviews [ 16 , 201 , 202 , 203 , 204 , 205 ] addressing similar research questions on the unexpected effects of GM food consumption. Keshani et al. [ 198 ], searching in 4 English databases, included experimental studies on GM crops’ potential effects on sperm parameters. The study finally included 7 rat feeding studies, which were all identified in our study, and indicated no harm to GM plants consumers. Edge et al. [ 199 ] addressed 30 review questions for including human studies, published in recent 20 years (1994–2014), on health effects of genetically engineered (GE) food crops, but found no human study on 25 questions. The remaining 5 questions, related to allergenicity and nutrient adequacy, were answered based on 21 human studies. The human studies were all excluded in our research because of no direct ingestion of GE food in the allergenicity assessment studies or no targeted outcomes in the nutrient assessment trial. To illustrate, the above-mentioned nutrient assessment clinical trial evaluated the effect of carrots containing twofold higher calcium content on calcium absorption and we thought it was not on outcome related to adverse events/effects. The conclusion of the research also supported that there were no clear adverse health effects associated with the consumption of GE food. Moreover, Dunn et al. [ 200 ] included both human and animal studies for examining the allergenicity of GM organisms and finally found 34 human studies and 49 animal studies eligible. In addition to 32 human studies which involved human serum for IgE binding or inhibition studies and not direct consumption of GM product, the rest 2 [ 206 , 207 ]studies were on actual ingestion of a GM food. However, they were not included in our research because of not targeted study type and unrelated outcomes. The conclusion agreed with the first two SRs that GM foods did not appear to be more allergenic than their conventional counterparts.
As for conventional reviews, Domingo showed special attention to the safety of GM food and published four literature reviews in 2000 [ 203 ], 2007 [ 204 ], 2011 [ 205 ] and 2016 [ 16 ]. Domingo searched two databases, PubMed and Scopus, to assess adverse/toxic effects of GM plants. In the latest updated review, he addressed the conclusion that GM soybeans, rice, corn/maize and wheat would be as safe as the parental species of these plants. However, our results may not be consistent with Domingo’s conclusion: we focus on a summarization of adverse events for GM food consumption through a systematic search in 7 databases; we identified 37 adverse events, 22 serious adverse events and 21 GM food-related adverse events; GM maize, soybean and rice with some specific GM events were all related to GM food-related adverse events. In addition, Domingo found a notable advance of studies published in scientific journals by biotechnology companies. Coincidentally, we did a Chi-square test to compare the adverse event reporting rates and found no significant differences between industry funding, government funding and non-funded articles. Besides, our systematic review validated Domingo’s findings that some GM plants were studied scarcely in recent years including GM potatoes discussed in the controversy of Pusztai case.
Strengths and limitations
In this review, a systematic search of major databases was conducted to identify all available studies in all languages on the adverse effects/events of GM food consumption. To make the inclusion and data synthesis comprehensive, both in vivo human and animal studies in all fields were included, with no limitations on the type of participant, type of intervention/exposure or whether control was included. The terms used for searching, containing all kinds of names of GM food, were based on a basic search on the internet by the researchers and the list was perfected as much as possible. With respect to additional searching, we went through multifarious news which reported controversy of GM food and thus we identified several hot studies by following the clue. In order to trace the potential conflicts of interest, we performed a Chi-square test for comparing adverse events report rates in articles funded by industry funding, government funding or unfunded articles, but found no statistical significance. Nevertheless, it was hard to conduct a quantitative data synthesis for the effects of GM food consumption on the adverse events because of the significant heterogeneity of the primary studies.
There are several limitations in this review. The methodological quality of the included studies is generally poor, which indicates a high or unclear risk of bias resulting from insufficient reporting of methodological components in the studies. Methodological quality may not be fully reflected based solely on the reporting of the manuscript. There were unclear descriptions of randomization procedures and a lack of blinding in all of the studies, which may have created potential performance biases and detection biases, as researchers might have been aware of the effects of interventions. The ability to perform meta-analysis was limited because of the heterogeneity of the participants, interventions (GM food in various GM events), comparisons, feeding doses, administration time, other exposure factors, and the variance of composite outcome measures used in the 204 included studies. When we did the manual search, we found that related publications were retracted sometimes, under the name of inadequate experimental designs or statistical analysis. For example, Séralini 2012 was retracted by Food and Chemical Toxicology , but subsequently republished in another journal [ 14 , 74 ]. This indicates that it was hard for us to find the original full-text papers of the retracted publications and articles provided by databases still have some unavoidable publication bias. The retraction on controversial researches may also cause the controversy for the public to doubt the reality of the studies published and to concern the safety of GM food. In addition, the lack of human studies is another key limitation of this research. As for the searching strategy, we did not include publication types as newspaper articles and comments. This was thought to be a limitation of this research because these sources may give us clues of related researches and can help us to do a manual search comprehensively. It is also an implication for future systematic reviews.
Implications for research
Future research should be conducted in humans, especially observational cohort studies. High-quality animal studies according to the ARRIVE reporting standard focusing on reproductive toxicity and carcinogenesis are still needed. Trials or studies should be registered prospectively, and be accessible. Furthermore, to address public concerns, future studies should focus on SAEs and GM food-related adverse events reported in this research such as NK603 maize, MON863 maize and MON810 maize. Meanwhile, some implications of findings still could be explored such as how GM food affects people’s eating habits, labelling of GM food and public choice. Some of the included studies conducted an intergenerational or multigenerational evaluation of the safety of GM food, but only two studies (Cyran N 2008 a and Cyran N 2008 c) in one article reported adverse events related to fertility. The differences in the results may be due to different interventions/exposures (GM food in certain GM events), laboratory animals, intervention/exposure time, experiment environment, etc. Therefore, it is necessary for subsequent studies to start with intergenerational or multigenerational research to verify the safety of GM food in terms of study design.
Serious adverse events accounted for 59.46% of the total 37 identified adverse events of GM consumption, which include: mortality, tumour or cancer, significantly lower number of pup deliveries, decreased learning and reaction abilities, and organ abnormalities in the stomach, intestinal adenoma, mammary glands, pituitary, liver and kidney. The interventions/exposures in the adverse event related studies emphasized on GM soybean, maize and rice in specific GM events. Animal studies occupy the lowest hierarchy of evidence, and there are flaws in study design and is not convincing enough. The evidence on the effect of GM consumption on humans is still insufficient. Further clinical trials and long-term cohort studies in human populations, especially on GM food-related adverse events and the corresponding GM events, are still warranted. It is better to prove the safety before they are approved for food consumption and it also suggests the necessity of labelling on GM food so that consumers can make their own choice.
Availability of data and materials
All data generated or analysed during this study are included in this published article.
Abbreviations
Genetically modified
Deoxyribonucleic acid
China National Knowledge Infrastructure
Preferred Reporting Items for Systematic Reviews and Meta-Analyses
Serious adverse event
Camelina sativa Seed oil
Blended fish oil
Body weight
Systematic reviews
Genetically engineered
Hundleby PA, Harwood WA (2019) Impacts of the EU GMO regulatory framework for plant genome editing. Food Energy Secur. https://doi.org/10.1002/fes3.161
Article Google Scholar
Sprink T, Eriksson D, Schiemann J et al (2016) Regulatory hurdles for genome editing: process- vs. product-based approaches in different regulatory contexts. Plant Cell Rep 35:1493–1506. https://doi.org/10.1007/s00299-016-1990-2
Article CAS Google Scholar
Georges F, Ray H (2017) Genome editing of crops: a renewed opportunity for food security. GM Crops Food 8:1–12. https://doi.org/10.1080/21645698.2016.1270489
ISAAA (2018) Global status of commercialized biotech/GM Crops in 2018: biotech crops continue to help meet the challenges of increased population and climate change. ISAAA Brief No. 54 [Online]. http://www.isaaa.org/resources/publications/briefs/54/default.asp . Accessed 17 July 2020
Andrew J, Ismail NW, Djama M (2018) An overview of genetically modified crop governance, issues and challenges in Malaysia. J Sci Food Agric 98:12–17. https://doi.org/10.1002/jsfa.8666
Hickey LT, Hafeez AN, Robinson H et al (2019) Breeding crops to feed 10 billion. Nat Biotechnol 37(7):744–754. https://doi.org/10.1038/s41587-019-0152-9
Giraldo PA, Shinozuka H, Spangenberg GC et al (2019) Safety assessment of genetically modified feed: is there any difference from food? Front Plant Sci 10:1592. https://doi.org/10.3389/fpls.2019.01592
ISAAA (2016) Global status of commercialized biotech/GM crops: 2016. ISAAA Brief No. 52 [Online]. http://www.isaaa.org/resources/publications/briefs/52/default.asp . Accessed 17 July 2020
Pray C, Huang J, Hu R, Deng H et al (2018) Prospects for cultivation of genetically engineered food crops in China. Global Food Secur Agric Policy Econ Environ 16:133–137. https://doi.org/10.1016/j.gfs.2018.01.003
Martinelli L, Karbarz M, Siipi H (2013) Science, safety, and trust: the case of transgenic food. Croat Med J 54:91–96. https://doi.org/10.3325/cmj.2013.54.91
Klümper W, Qaim M (2014) A meta-analysis of the impacts of genetically modified crops. PLoS ONE. https://doi.org/10.1371/journal.pone.0111629
Losey JE, Rayor LS, Carter ME (1999) Transgenic pollen harms monarch larvae. Nature 399:214. https://doi.org/10.1038/20338
Ewen SW, Pusztai A (1999) Effect of diets containing genetically modified potatoes expressing Galanthus nivalis lectin on rat small intestine. Lancet 354:1353–1354. https://doi.org/10.1016/S0140-6736(98)05860-7
Séralini GE, Clair E, Mesnage R et al (2012) Long term toxicity of a Roundup herbicide and a Roundup-tolerant genetically modified maize [retracted in: Food Chem Toxicol. 2014 Jan;63:244]. Food Chem Toxicol 50(11):4221–4231. https://doi.org/10.1016/j.fct.2012.08.005
Genetic Literacy Project (2017) Where are GMOs grown and banned? [Online]. https://gmo.geneticliteracyproject.org/FAQ/where-are-gmos-grownand-banned/ . Accessed 5 Sept 2020
Domingo JL (2016) Safety assessment of GM plants: an updated review of the scientific literature. Food Chem Toxicol 95:12–18. https://doi.org/10.1016/j.fct.2016.06.013 ( Epub 2016 Jun 16 )
Moher D, Liberati A, Tetzlaff J et al (2009) Preferred reporting items for systematic reviews and meta analyses: the PRISMA statement. BMJ. https://doi.org/10.1136/bmj.b2535
FDA (2016) What is a serious adverse event? [Online]. https://www.fda.gov/safety/reporting-serious-problems-fda/what-serious-adverse-event . Accessed 28 Sept 2020
Al-Harbi A, Lary S, Edwards MG et al (2019) A proteomic-based approach to study underlying molecular responses of the small intestine of Wistar rats to genetically modified corn (MON810). Transgenic Res 28(5–6):479–498. https://doi.org/10.1007/s11248-019-00157-y
Appenzeller LM, Munley SM, Hoban D et al (2009) Subchronic feeding study of grain from herbicide-tolerant maize DP-Ø9814Ø-6 in Sprague-Dawley rats. Food Chem Toxicol 47:2269–2280. https://doi.org/10.1016/j.fct.2009.06.014
Bai H, Wang Z, Hu R et al (2015) A 90-day toxicology study of meat from genetically modified sheep overexpressing TLR4 in Sprague-Dawley rats. PLoS ONE. https://doi.org/10.1371/journal.pone.0121636
Bakke-Mckellep AM, Koppang EO, Gunnes G et al (2007) Histological, digestive, metabolic, hormonal and some immune factor responses in Atlantic salmon, Salmo salar L, fed genetically modified soybeans. J Fish Dis 30:65–79. https://doi.org/10.1111/j.1365-2761.2007.00782.x
Bakke-McKellep AM, Sanden M, Danieli A et al (2008) Atlantic salmon ( Salmo salar L.) parr fed genetically modified soybeans and maize: histological, digestive, metabolic, and immunological investigations. Res Vet Sci 84:395–408. https://doi.org/10.1016/j.rvsc.2007.06.008
Buzoianu SG, Walsh MC, Rea MC et al (2012) Effect of feeding genetically modified Bt MON810 maize to ∼ 40-day-old pigs for 110 days on growth and health indicators. Animal 6:1609–1619. https://doi.org/10.1017/S1751731112000249
Buzoianu SG, Walsh MC, Rea MC et al (2012) Effects of feeding Bt maize to sows during gestation and lactation on maternal and offspring immunity and fate of transgenic material. PLoS ONE. https://doi.org/10.1371/journal.pone.0047851
Carman JA, Vlieger HR, Ver Steeg LJ et al (2013) A long-term toxicology study on pigs fed a combined genetically modified (GM) soy and GM maize diet. J Organic Systems 1:1–12
Google Scholar
Chen X, Gao MQ, Liang D et al (2017) Safety assessment of genetically modified milk containing human beta-defensin-3 on rats by a 90-day feeding study. Food Chem Toxicol 100:34–41. https://doi.org/10.1016/j.fct.2016.12.012
Chen YN, Hwang WZ, Fang TJ et al (2011) The impact of transgenic papaya (TPY10-4) fruit supplementation on immune responses in ovalbumin-sensitised mice. J Sci Food Agric 91:539–546. https://doi.org/10.1002/jsfa.4218
Chen ZL, Gu H, Li Y et al (2003) Safety assessment for genetically modified sweet pepper and tomato. Toxicology 188:297–307. https://doi.org/10.1016/s0300-483x(03)00111-2
Chukwudebe A, Privalle L, Reed A et al (2012) Health and nutritional status of Wistar rats following subchronic exposure to CV127 soybeans. Food Chem Toxicol 50:956–971. https://doi.org/10.1016/j.fct.2011.11.034
Cisterna B, Flach F, Vecchio L et al (2008) Can a genetically-modified organism-containing diet influence embryo development? A preliminary study on pre-implantation mouse embryos. Eur J Histochem 52:263–267. https://doi.org/10.4081/1226
Cyran N, Gülly C, Handl S et al (2008) Biological effects of transgenic maize NK603xMON810 fed in long term reproduction studies in mice. FiBL, Wien
de Vendômois JS, Roullier F, Cellier D et al (2009) A comparison of the effects of three GM corn varieties on mammalian health. Int J Biol Sci 5:706–726. https://doi.org/10.7150/ijbs.5.706
Delaney B, Appenzeller LM, Munley SM et al (2008) Subchronic feeding study of high oleic acid soybeans (Event DP-3Ø5423-1) in Sprague-Dawley rats. Food Chem Toxicol 46:3808–3817. https://doi.org/10.1016/j.fct.2008.10.003
EFSA Panel on Genetically Modified Organisms (GMO), Naegeli H, Birch AN et al (2018) Assessment of genetically modified soybean MON 87751 for food and feed uses under Regulation (EC) No 1829/2003 (application EFSA-GMO-NL-2014-121). EFSA J 16(8):e05346. https://doi.org/10.2903/j.efsa.2018.5346
El-Shamei ZS, Gab-Alla AA, Shatta AA (2012) Histopathological changes in some organs of male rats fed on genetically modified corn (Ajeeb YG). Am J Sci 10(8):684–696
Ermakova I (2005) Influence of genetically modified soya on the birth-weight and survival of rat pups. In: Epigenetics, transgenic plants& risk assessment.
Gao MQ, Zhang R, Yang Y et al (2018) A subchronic feeding safety evaluation of transgenic milk containing human β-defensin 3 on reproductive system of C57BL/6J mouse. Food Chem Toxicol 115:198–204. https://doi.org/10.1016/j.fct.2018.03.007
Gu J, Krogdahl Å, Sissener NH et al (2013) Effects of oral Bt-maize (MON810) exposure on growth and health parameters in normal and sensitised Atlantic salmon, Salmo salar L. Br J Nutr 109:1408–1423. https://doi.org/10.1017/S000711451200325X
Guo QY, He LX, Zhu H et al (2015) Effects of 90-day feeding of transgenic maize BT799 on the reproductive system in male Wistar rats. Int J Environ Res Public Health 12:15309–15320. https://doi.org/10.3390/ijerph121214986
Hammond B, Dudek R, Lemen J et al (2004) Results of a 13 week safety assurance study with rats fed grain from glyphosate tolerant corn. Food Chem Toxicol 42:1003–1014. https://doi.org/10.1016/j.fct.2004.02.013
Hammond B, Lemen J, Dudek R et al (2006) Results of a 90-day safety assurance study with rats fed grain from corn rootworm-protected corn. Food Chem Toxicol 44:147–160. https://doi.org/10.1016/j.fct.2005.06.008
He XY, Tang MZ, Luo YB et al (2009) A 90-day toxicology study of transgenic lysine-rich maize grain (Y642) in Sprague-Dawley rats. Food Chem Toxicol 47:425–432. https://doi.org/10.1016/j.fct.2008.11.032
Healy C, Hammond B, Kirkpatrick J (2008) Results of a 13-week safety assurance study with rats fed grain from corn rootworm-protected, glyphosate-tolerant MON 88017 corn. Food Chem Toxicol 46:2517–2524. https://doi.org/10.1016/j.fct.2008.04.005
Ibrahim MA, Okasha EF (2016) Effect of genetically modified corn on the jejunal mucosa of adult male albino rat. Exp Toxicol Pathol 68:579–588. https://doi.org/10.1016/j.etp.2016.10.001
Kiliç A, Akay MT (2008) A three generation study with genetically modified Bt corn in rats: Biochemical and histopathological investigation. Food Chem Toxicol 46:1164–1170. https://doi.org/10.1016/j.fct.2007.11.016
Kiliçgün H, Gürsul C, Sunar M et al (2013) The comparative effects of genetically modified maize and conventional maize on rats. J Clin Anal Med 4:136–139
Lee NJ, Yang BC, Hwang JS et al (2010) Effects of cloned-cattle meat diet on reproductive parameters in pregnant rabbits. Food Chem Toxicol 48:871–876. https://doi.org/10.1016/j.fct.2009.12.025
Lee NJ, Yang BC, Im GS et al (2013) No long-term feeding toxicities on the health status in rats fed with cloned Korean native beef cattle (Hanwoo) meat. Toxicol Pathol 41:872–879. https://doi.org/10.1177/0192623312470762
Lin HT, Lee WC, Tsai YT et al (2016) Subchronic immunotoxicity assessment of genetically modified virus-resistant papaya in rats. J Agric Food Chem 64:5935–5940. https://doi.org/10.1021/acs.jafc.6b02242
Liu P, He X, Chen D et al (2012) A 90-day subchronic feeding study of genetically modified maize expressing Cry1Ac-M protein in Sprague-Dawley rats. Food Chem Toxicol 50:3215–3221. https://doi.org/10.1016/j.fct.2012.06.009
Liu Q, Yang W, Li M et al (2017) Effects of 60-week feeding diet containing Bt rice expressing the Cry1Ab protein on the offspring of inbred Wuzhishan Pigs fed the same diet. J Agric Food Chem 65:10300–10309. https://doi.org/10.1021/acs.jafc.7b04067
Liu S, Li CX, Feng XL et al (2013) Safety assessment of meat from transgenic cattle by 90-day feeding study in rats. Food Chem Toxicol 57:314–321. https://doi.org/10.1016/j.fct.2013.04.00
Liu S, Liu HB, Wang HL et al (2019) Evaluation of behavioral profiles in mice fed with milk supplemented diets derived from human lactoferrin gene-modified cows. Regul Toxicol Pharmacol 104:133–140. https://doi.org/10.1016/j.yrtph.2019.03.008
Liu Y, Zhang S, Zhou Q et al (2020) Subchronic feeding toxicity studies of drought-tolerant transgenic wheat MGX11-10 in Wistar Han RCC rats. Food Chem Toxicol 137:111129. https://doi.org/10.1016/j.fct.2020.111129
MacKenzie SA, Lamb I, Schmidt J et al (2007) Thirteen week feeding study with transgenic maize grain containing event DAS-Ø15Ø7-1 in Sprague-Dawley rats. Food Chem Toxicol 45:551–562. https://doi.org/10.1016/j.fct.2006.09.016
Malatesta M, Boraldi F, Annovi G et al (2008) A long-term study on female mice fed on a genetically modified soybean: effects on liver ageing. Histochem Cell Biol 130(5):967–977. https://doi.org/10.1007/s00418-008-0476-x
Malatesta M, Caporaloni C, Gavaudan S et al (2002) Ultrastructural morphometrical and immunocytochemical analyses of hepatocyte nuclei from mice fed on genetically modified soybean [published correction appears in Cell Struct Funct. 2002 Oct;27(5):399]. Cell Struct Funct 27(4):173–180. https://doi.org/10.1247/csf.27.173
Malatesta M, Caporaloni C, Rossi L et al (2002) Ultrastructural analysis of pancreatic acinar cells from mice fed on genetically modified soybean. J Anat 201(5):409–415. https://doi.org/10.1046/j.0021-8782.2002.00103.x
Mao J, Sun X, Cheng JH et al (2016) A 52-week safety study in cynomolgus macaques for genetically modified rice expressing Cry1Ab/1Ac protein. Food Chem Toxicol 95:1–11. https://doi.org/10.1016/j.fct.2016.06.015
Nouri-Ellouz O, Zeghal N, Makni S et al (2015) New food from a potato somatic hybrid: nutritional equivalence and safety assessment by a feeding study on rats. J Sci Food Agric 95(9):1911–1917. https://doi.org/10.1002/jsfa.6898
Oliva N, Florida Cueto-Reaño M, Trijatmiko KR et al (2020) Molecular characterization and safety assessment of biofortified provitamin A rice. Sci Rep 10(1):1376. https://doi.org/10.1038/s41598-020-57669-5
Papineni S, Golden RM, Thomas J (2017) The aryloxyalkanoate dioxygenase-12 (AAD-12) protein is not acutely toxic in mice[J]. Food Chem Toxicol 110:200–203. https://doi.org/10.1016/j.fct.2017.10.036
Papineni S, Murray JA, Ricardo E et al (2017) Evaluation of the safety of a genetically modified DAS-444Ø6-6 soybean meal and hulls in a 90-day dietary toxicity study in rats. Food Chem Toxicol 109(Pt 1):245–252. https://doi.org/10.1016/j.fct.2017.08.048
Papineni S, Passage JK, Ekmay RD et al (2018) Evaluation of 30% DAS-444Ø6-6 soybean meal in a subchronic rat toxicity study. Regul Toxicol Pharmacol 94:57–69. https://doi.org/10.1016/j.yrtph.2018.01.005
Poulsen M, Kroghsbo S, Schrøder M et al (2007) A 90-day safety study in Wistar rats fed genetically modified rice expressing snowdrop lectin Galanthus nivalis (GNA). Food Chem Toxicol 45(3):350–363. https://doi.org/10.1016/j.fct.2006.09.002
Qian ZY, Zhang SJ, Zhang L et al (2018) Subchronic toxicity study in rats evaluating genetically modified DAS-81419-2 soybean. Regul Toxicol Pharmacol 96:48–56. https://doi.org/10.1016/j.yrtph.2018.04.019
Qian ZY, Bultman J, Papineni S et al (2018) Safety evaluation of DAS-44406-6 soybeans in Wistar rats. Regul Toxicol Pharmacol 92:152–164. https://doi.org/10.1016/j.yrtph.2017.11.016
Tudisco R, Calabrò S, Cutrignelli MI et al (2015) Genetically modified soybean in a goat diet: Influence on kid performance. Small Rumin Res 126:67–74. https://doi.org/10.1016/j.smallrumres.2015.01.023
Richards HA, Han CT, Hopkins RG et al (2003) Safety assessment of recombinant green fluorescent protein orally administered to weaned rats. J Nutr 133(6):1909–1912. https://doi.org/10.1093/jn/133.6.1909
Sanden M, Ornsrud R, Sissener NH et al (2013) Cross-generational feeding of Bt ( Bacillus thuringiensis )-maize to zebrafish ( Danio rerio ) showed no adverse effects on the parental or offspring generations. Br J Nutr 110(12):2222–2233. https://doi.org/10.1017/S0007114513001748
Schrøder M, Poulsen M, Wilcks A et al (2007) A 90-day safety study of genetically modified rice expressing Cry1Ab protein ( Bacillus thuringiensis toxin) in Wistar rats. Food Chem Toxicol 45(3):339–349. https://doi.org/10.1016/j.fct.2006.09.001
Séralini GE, Cellier D, de Vendomois JS (2007) New analysis of a rat feeding study with a genetically modified maize reveals signs of hepatorenal toxicity. Arch Environ Contam Toxicol 52(4):596–602. https://doi.org/10.1007/s00244-006-0149-5
Séralini GE, Clair E, Mesnage R et al (2014) Republished study: long-term toxicity of a roundup herbicide and a roundup-tolerant genetically modified maize. Environ Sci Eur 26(1):14. https://doi.org/10.1186/s12302-014-0014-5
Sheng Y, Qi X, Liu Y et al (2014) Subchronic toxicity study in vivo and allergenicity study in vitro for genetically modified rice that expresses pharmaceutical protein (human serum albumin). Food Chem Toxicol 72:242–246. https://doi.org/10.1016/j.fct.2014.07.030
EFSA Panel on Food Contact Materials, Enzymes, Processing Aids (EFSA CEP Panel) et al (2019) Safety evaluation of the food enzyme endo-1,4-β-xylanase from a genetically modified Bacillus licheniformis (strain NZYM-CE). EFSA J 17(4):e05685. https://doi.org/10.2903/j.efsa.2019.5685 ( Published 2019 Apr 30 )
EFSA Panel on Food Contact Materials, Enzymes, Flavourings and Processing Aids (CEF) et al (2018) Safety evaluation of the food enzyme endo-1,4-β-xylanase from a genetically modified Bacillus subtilis (strain LMG S-24584). EFSA J 16(10):e05447. https://doi.org/10.2903/j.efsa.2018.5447 ( Published 2018 September 27 )
EFSA Panel on Food Contact Materials, Enzymes and Processing Aids (CEP), Silano V et al (2018) Safety evaluation of the food enzyme endo-1,4-β-xylanase from a genetically modified Aspergillus oryzae (strain NZYM-FA). EFSA J 16(11):e05480. https://doi.org/10.2903/j.efsa.2018.5480 ( Published 2018 Nov 16 )
EFSA Panel on Food Contact Materials, Enzymes and Processing Aids (CEP), Silano V et al (2018) Safety evaluation of the food enzyme endo-1,4-β-xylanase from a genetically modified Trichoderma reesei (strain DP-Nzd22). EFSA J 16(11):e05479. https://doi.org/10.2903/j.efsa.2018.5479 ( Published 2018 Nov 30 )
EFSA Panel on Food Contact Materials, Enzymes, Processing Aids (CEP) et al (2019) Safety evaluation of the food enzyme pullulanase from a genetically modified Bacillus licheniformis (strain DP-Dzp39). EFSA J 17(1):e05554. https://doi.org/10.2903/j.efsa.2019.5554 ( Published 2019 Jan 10 )
EFSA Panel on Food Contact Materials, Enzymes, Processing Aids (CEP) et al (2018) Safety evaluation of the food enzyme α-amylase from a genetically modified Aspergillus niger (strain NZYM-MC). EFSA J 16(10):e05451. https://doi.org/10.2903/j.efsa.2018.5451 ( Published 2018 Oct 31 )
EFSA Panel on Food Contact Materials, Enzymes, Flavourings and Processing Aids (CEF) et al (2018) Safety evaluation of the food enzyme alpha-amylase from a genetically modified Bacillus licheniformis (strain NZYM-AN). EFSA J 16(7):e05317. https://doi.org/10.2903/j.efsa.2018.5317 ( Published 2018 Jul 6 )
EFSA Panel on Food Contact Materials, Enzymes, Flavourings and Processing Aids (CEF) et al (2018) Safety evaluation of the food enzyme glucose oxidase from a genetically modified Aspergillus oryzae (strain NZYM-KP). EFSA J 16(7):e05319. https://doi.org/10.2903/j.efsa.2018.5319 ( Published 2018 Jul 6 )
EFSA Panel on Food Contact Materials, Enzymes, Flavourings and Processing Aids (CEF) et al (2018) Safety evaluation of the food enzyme endo-1,4-β-xylanase from a genetically modified Aspergillus niger (strain XEA). EFSA J 16(4):e05228. https://doi.org/10.2903/j.efsa.2018.5228 ( Published 2018 Apr 27 )
EFSA Panel on Food Contact Materials, Enzymes, Flavourings and Processing Aids (CEF) et al (2018) Safety evaluation of the food enzyme aqualysin 1 from a genetically modified Bacillus subtilis (strain LMGS 25520). EFSA J 16(5):e05170. https://doi.org/10.2903/j.efsa.2018.5170 ( Published 2018 May 2 )
EFSA Panel on Food Contact Materials, Enzymes, Flavourings and Processing Aids (CEF) et al (2018) Safety evaluation of the food enzyme xylanase from a genetically modified Bacillus subtilis strain TD160(229). EFSA J 16(1):e05008. https://doi.org/10.2903/j.efsa.2018.5008 ( Published 2018 Jan 22 )
EFSA Panel on Food Contact Materials, Enzymes, Flavourings and Processing Aids (CEF) et al (2018) Safety evaluation of food enzyme xylanase from a genetically modified Bacillus subtilis (strain LMG S-27588). EFSA J 16(5):e05169. https://doi.org/10.2903/j.efsa.2018.5169 ( Published 2018 May 2 )
Talyn B, Lemon R, Badoella M et al (2019) Roundup ®, but not roundup-ready ® corn, increases mortality of drosophila melanogaster. Toxics. https://doi.org/10.3390/toxics7030038
Tang M, Xie T, Cheng W et al (2012) A 90-day safety study of genetically modified rice expressing rhIGF-1 protein in C57BL/6J rats [published correction appears in Transgenic Res. 2012 Aug;21(4):927]. Transgenic Res 21(3):499–510. https://doi.org/10.1007/s11248-011-9550-6
Tang X, Han F, Zhao K et al (2012) A 90-day dietary toxicity study of genetically modified rice T1C–1 expressing Cry1C protein in Sprague Dawley rats. PLoS ONE 7(12):e52507. https://doi.org/10.1371/journal.pone.0052507
Teshima R, Watanabe T, Okunuki H et al (2002) Effect of subchronic feeding of genetically modified corn (CBH351) on immune system in BN rats and B10A mice. Shokuhin Eiseigaku Zasshi 43(5):273–279. https://doi.org/10.3358/shokueishi.43.273
Trabalza Marinucci M, Brandi G, Rondini C (2008) A three-year longitudinal study on the effects of a diet containing genetically modified Bt176 maize on the health status and performance of sheep. Livest Sci 2008(113):178–190
Walsh MC, Buzoianu SG, Gardiner GE et al (2011) Fate of transgenic DNA from orally administered Bt MON810 maize and effects on immune response and growth in pigs. PLoS ONE 6(11):e27177. https://doi.org/10.1371/journal.pone.0027177
Walsh MC, Buzoianu SG, Gardiner GE et al (2012) Effects of short-term feeding of Bt MON810 maize on growth performance, organ morphology and function in pigs. Br J Nutr 107(3):364–371. https://doi.org/10.1017/S0007114511003011
Walsh MC, Buzoianu SG, Rea MC et al (2012) Effects of feeding Bt MON810 maize to pigs for 110 days on peripheral immune response and digestive fate of the cry1Ab gene and truncated Bt toxin. PLoS ONE 7(5):e36141. https://doi.org/10.1371/journal.pone.0036141
Wang X, He X, Zou S et al (2016) A subchronic feeding study of dicamba-tolerant soybean with the dmo gene in Sprague-Dawley rats. Regul Toxicol Pharmacol 77:134–142. https://doi.org/10.1016/j.yrtph.2016.02.001
West AL, Miles EA, Lillycrop KA et al (2019) Postprandial incorporation of EPA and DHA from transgenic Camelina sativa oil into blood lipids is equivalent to that from fish oil in healthy humans. Br J Nutr 121(11):1235–1246. https://doi.org/10.1017/S0007114519000825
Wu Y, Xu Y, Du Y et al (2017) Dietary safety assessment of genetically modified rice EH rich in β-carotene. Regul Toxicol Pharmacol 88:66–71. https://doi.org/10.1016/j.yrtph.2017.05.019
Xiao GJ, Jiang SW, Qian LL et al (2016) A 90-day feeding study in rats to assess the safety of genetically engineered pork. PLoS ONE 11(11):e0165843. https://doi.org/10.1371/journal.pone.0165843
Xie Z, Zou S, Xu W et al (2018) No subchronic toxicity of multiple herbicide-resistant soybean FG72 in Sprague-Dawley rats by 90-days feeding study. Regul Toxicol Pharmacol 94:299–305. https://doi.org/10.1016/j.yrtph.2018.02.004
Yang L, Sun Y, Wang Y et al (2014) Effects of dietary transgenic poplar leaf pellets on performance and tissues in rabbits. J Sci Food Agric 94(6):1163–1167. https://doi.org/10.1002/jsfa.6388
Yen GC, Lin HT, Cheng YH et al (2011) Food safety evaluation of papaya fruits resistant to papaya ring spot virus. J Food Drug Anal 19(2):269–377
CAS Google Scholar
Yong L, Liu YM, Jia XD et al (2012) Subchronic toxicity study of GH transgenic carp. Food Chem Toxicol 50(11):3920–3926. https://doi.org/10.1016/j.fct.2012.07.064
Zeljenková D, Aláčová R, Ondrejková J et al (2016) One-year oral toxicity study on a genetically modified maize MON810 variety in Wistar Han RCC rats (EU 7th Framework Programme project GRACE). Arch Toxicol 90(10):2531–2562. https://doi.org/10.1007/s00204-016-1798-4
Zeljenková D, Ambrušová K, Bartušová M et al (2014) Ninety-day oral toxicity studies on two genetically modified maize MON810 varieties in Wistar Han RCC rats (EU 7th Framework Programme project GRACE). Arch Toxicol 88(12):2289–2314. https://doi.org/10.1007/s00204-014-1374-8
Zhou C, Wang JW, Huang KL et al (2011) A 90-day safety study in Sprague-Dawley rats fed milk powder containing recombinant human lactoferrin (rhLF) derived from transgenic cloned cattle. Drug Chem Toxicol 34(4):359–368. https://doi.org/10.3109/01480545.2010.542465
Zhou XH, Dong Y, Xiao X et al (2011) A 90-day toxicology study of high-amylose transgenic rice grain in Sprague-Dawley rats. Food Chem Toxicol 49:3112–3118. https://doi.org/10.1016/j.fct.2011.09.024
Zhu HJ, Chen Y, Li YH et al (2015) A 90 day safety assessment of genetically modified rice expressing Cry1Ab/1Ac protein using an aquatic animal model [published correction appears in J Agric Food Chem. 2015 Aug 26;63(33):7462]. J Agric Food Chem 63(14):3627–3633. https://doi.org/10.1021/jf5055547
Zhu Y, He X, Luo Y et al (2013) A 90-day feeding study of glyphosate-tolerant maize with the G2-aroA gene in Sprague-Dawley rats. Food Chem Toxicol 51:280–287. https://doi.org/10.1016/j.fct.2012.09.008
Zou S, Tang M, He X et al (2015) A 90-day subchronic study of rats fed lean pork from genetically modified pigs with muscle-specific expression of recombinant follistatin. Regul Toxicol Pharmacol 73(2):620–628. https://doi.org/10.1016/j.yrtph.2015.09.009
Dong SS, Zhang DN, Zhang ZH et al (2019) Ecotoxicological effects of transgenic mCry1Ac maize (BT799) on zebrafish. Ying Yong Sheng Tai Xue Bao 30(8):2845–2853. https://doi.org/10.13287/j.1001-9332.201908.031
Hu Y, Piao J, Yang X et al (2012) Nutritional components and sub-chronic toxicity of genetically modified rice expressing human lactoferrin. Wei Sheng Yan Jiu 41(1):6–12. https://doi.org/10.2166/wst.2012.090
Bai H (2015) Bio-Safety assessment of transgenic sheep overexpressing oTLR4. PhD Thesis, China Agricultural University, Beijing, China
Bao ZK (2016) Safety assessment of GH-Transgenic dairy goats. MS Thesis, Nanjing Agricultural University, Nanjing, China
Cao ZH (2014) Safety assessment of transgenic Bt rice in growing pig diet. MS Thesis, Henan University of Science and Technology, Henan, China
Chen XP, Zhuo Q, Piao JH et al (2004) Immunotoxicologic assessment of transgenetic rice. J Hyg Res 33(1):77–80
Chu HH, Si QQ, Xu Y et al (2016) Effect of genetically modified soybean meal on the immune function, intestinal digestive enzyme activities and serum biochemical indexes of growing pigs. J Qingdao Agric Univ Nat Sci 33(02):119–123. https://doi.org/10.3969/J.ISSN.1674-148X.2016.02.008
Dai YN, Yang XZ, Liu Y et al (2018) Acute oral toxicity and 90 days feeding test of recombinant human lactoferrin. J Hyg Res 47(02):286–311
Du HF (2006) Safety assessment of transgenic rice used in broiler diet. PhD Thesis, Chinese Academy of Agricultural Sciences, Beijing, China
Feng XL, Wang HL, Li CX et al (2017) Safety assessment of meat from transgenic cattle by 90-day feeding study in rats. J Hyg Res 29(1):19–25. https://doi.org/10.13590/j.cjfh.2017.01.005
Feng YQ, Hu J, Zhi Y et al (2013) The effect of exposure to transgenic Bt rice on the immune system of parental female rats. Chin J Food Hyg 25(4):298–302
Feng YQ, Wang EH, Zhi Y et al (2013) The effect of exposure to transgenic Bt rice on reproductive system of male offspring rats. Chin J Food Hyg 25(02):113–117
Guo MF (2018) A three generation study to evaluate reproductive and neurodevelopmental toxicity of genetically modified maize with Cry1Ab and epsps genes in SD rats. MS Thesis, Chinese Center for Disease Control and Prevention, Beijing, China
Hu J (2013) Establish of a rat model for development immunotoxicity and its application in safety evaluation of transgenic CryAb/Ac Rice. MS Thesis, Chinese Center for Disease Control and Prevention, Beijing, China
Huang Q, Xu HB, Gao F et al (2009) Anaphylactic reactions in WZS minipig orally induced by glycinin. J Hyg Res 38(05):531–534
Jia XD, Li N, Wang W et al (2005) Assessment of allergenicity of genetically modified rice S86 by BN rat model. Chin J Food Hyg 01:7–9. https://doi.org/10.13590/j.cjfh.2005.01.003
Li M (2012) Safety evaluation of recombinant herbicide-resistant protein AROa-CC-M and transgenic insect-resistant maize BT-799. MS Thesis, Chinese Academy of Agricultural Sciences, Beijing, China
Li M, Piao JH, Yang XG (2010) Subchronic toxicity test of genetically modified rice with double antisense starch-branching enzyme gene. J Hyg Res 39(04):436–443
Li R, Wang J, Jiang WL et al (2012) Effects of rice genetically modified with HJC-1 and G6-EPSPS genes on immunological parameters in Wuzhishan minipigs. J Environ Health 29(08):689–692. https://doi.org/10.16241/j.cnki.1001-5914.2012.08.017
Li YH, Piao JH, Chen XP et al (2004) Immunotoxicologic assessment on transgenic rice. China Public Health 20(04):20–22
Li YH, Piao JH, Zhuo Q et al (2004) Study on the teratogenicity effects of genetically modified rice with Xa21 on rats. J Hyg Res 33(06):710–712
Liang LQ, Wang J, Cao XC et al (2010) Toxicity analysis of common carp transferred salmon growth hormone gene. Food Sci 31(05):261–265
Liu HT, Wang CR, Liu L et al (2018) Acute toxicity of fresh leaves of insect resistant transgenic populus nigra to mice. J Anhui Agric Sci 46(01):94–136. https://doi.org/10.13989/j.cnki.0517-6611.2018.01.028
Liu HL, Wang J, Zeng Q et al (2012) Effects of rapeseed genetically modified with bar gene on immunological indicators in WZS minipigs. J Environ Health 29(11):977–980. https://doi.org/10.16241/j.cnki.1001-5914.2012.11.020
Liu SS, Tan JZ, Sun Z et al (2011) Effects of glyphosate—resistant soybean meal on immune function of AA broilers. Chin J Anim Sci 47(13):41–46
Liu S, Wang XD, Feng XL et al (2013) Twenty-eight days feeding study on human lactoferrin expressed by cattle mammary bioreactor in mice. Chin J Public Health 29(02):230–232
Liu YH, Jiang SQ, Zhang J et al (2018) Subchronic toxicity of genetically modified Herbicide-resistant maize MON87427 with Cp4epsps gene in Wistar rats. J Public Health Prevent Med 29(06):17–20. https://doi.org/10.3969/j.issn.1006-2483.2018.06.004
Liu YF, Liu WH, He L et al (2008) Acute toxicity and mutagenic effect of transgenic rice on mice. J Hunan Univ Sci Technol Nat Sci Ed 23(04):111–117
Liu YF, Liu WH, He L et al (2008) Effects of resistant insects transgenic hybrid rice 21S/MSB on behavior and physiology of SD rats. Life Sci Res 12(03):257–261. https://doi.org/10.16605/j.cnki.1007-7847.2008.03.013
Lu MJ, Li F, Zhou GL et al (2008) Assessment of an anti-LeETR1 genetically modified tomato on reproductive-development toxicity and transferability of the transgene. J Toxicol 22(04):272–274. https://doi.org/10.16421/j.cnki.1002-3127.2008.04.006
Lu CB, Lin ZB, Zhang Y et al (2016) Effects of glyphosate-resistant transgenic soybean on physical enginery of male mice. Acta Agriculturae Zhejiangensis 28(07):1115–1120. https://doi.org/10.3969/j.issn.1004-1524.2016.07.04
Lu CB, Yang DY, Gao Z et al (2012) Safety assessment of reproductive system in male mice fed with genetically modified soybeans. J Yangzhou Univ Agric Life Sci Ed 33(01):23–27. https://doi.org/10.16872/j.cnki.1671-4652.2012.01.006
Lu CB, Zhang Y, Chen BH et al (2017) Effects of glyphosate-resistant transgenic soybean on in vitro fertilization of male mice with reproductive damage. Acta Agriculturae Zhejiangensis 29(06):910–916. https://doi.org/10.3969/j.issn.1004-1524.2017.06.08
Lu CB, Zhou W, Liu B et al (2013) Effects of transgenic soybean on reproductive system in male mice. Soybean Sci 32(01):119–123
Lv L, Guo J, Li SF et al (2013) Effects of transphytase gene maize on organ development and pathological changes of broilers. Chin J Anim Sci 49(05):31–34
Ma BT, Yuan XY, Wang XD et al (2017) Animal experiment of recombinant human lactoferrin based on the 28 days repeated oral toxicity. J Hyg Res 46(03):443–454
Ma YM, Wang J, Jiang WL et al (2012) Subchronic oral toxicity of genetically modified cottonseed with FBP7-iaaM gene in rats. J Environ Health 29(11):1001–1007. https://doi.org/10.16241/j.cnki.1001-5914.2012.11.024
Qi XZ, Wang J, Zhou C et al (2010) Effect of transferred human lactoferrin milk powder on serum iron and ferritin in rats. Food Sci 31(23):340–343
Qin HF (2012) Safety assessment of rice genetically modified with Cry1Ac and sck feeding studies on broilers. PhD Thesis, Chinese Academy of Agricultural Sciences, Beijing, China.
Qiu ZL, Sun N, Wang J et al (2011) Sub-Chronic toxicity study of transgenic cottonseed in SD rats. Progr Mod Biomed 11(12):2215–2220. https://doi.org/10.13241/j.cnki.pmb.2011.12.010
Song LS, Gao GQ, Wei ZY et al (2017) Effects of Fat1 transgenic milk on the health and reproductive ability of mice. Lab Anim Sci 34(03):28–37
Song Y (2013) Establishment of immunotoxicity screening system in rodents and its application in immunotoxicity evaluation of pesticides and genetically modified foods. PhD Thesis, Chinese Center for Disease Control and Prevention, Beijing, China
Sun XW, Liang LQ, Yan XC et al (1998) Research on transgenic carp as food. High Technol Lett 03:3–5
Sun Z, Liu SS, Tan JZ et al (2011) Effects of glyphosate—resistant soybean meal on immune function of AA broilers. Chin J Anim Sci 23:836–841
Tan JZ (2011) The feed safety assessment of Glyphosate-Tolerant soybean meal in broilers. MS Thesis, Chinese Academy of Agricultural Sciences, Beijing, China
Tang XQ, Wang YF, Pei LJ et al (2019) Long-term toxicity study on transgenic rice T2A–1 with cry2A* gene. Chin J Food Hyg 31(6):510–516. https://doi.org/10.13590/j.jfh.2019.06.002
Tao R (2008) Detection of transgenic ingredients in feed and primary assessment on the safety of aquatic livestock fed transgenic soybean. MS Thesis, Chinese Academy of Sciences, Shandong, China
Wang EH (2014) A study to access effects of transgenic Cry1Ab/Ac Rice TT51 on reproductive and neural development in rats. PhD Thesis, Chinese Center for Disease Control and Prevention, Beijing, China
Wang EH, Yu Z, Fang HQ et al (2013) Effect of transgenic Bt rice TT51 on early physiological and neurological development of rats offspring. Chin J Food Hyg 25(06):485–488. https://doi.org/10.13590/j.cjfh.2013.06.008
Wang HM, Yin JY, Zhai WS, et al (2015) Toxic pathology of cynomolgus monkeys fed transgenic rice for 52 weeks. In: The 7th National Toxicology Conference of China Toxicology Society and the 8th Hubei Science and Technology Forum, Wuhan, China, pp 442–443
Wang J, Jiang WL, Wang XJ et al (2002) Toxicological safety evaluation of transgenic T5 line pepper. Chin J Urban Rural Ind Hyg 01:46
Wang J, Jiang WL, Wang Y et al (2012) Subacute oral toxicity of recombinant human lactoferrin from transgenic cows in rats on 28d. J Toxicol 26(05):393–397
Wang J, Li R, Liu HL (2012) Assessment of allergenicity of rice genetically modified with HJC-1 and G6-EPSPS genes by BN rats model. J Environ Health 29(11):967–970. https://doi.org/10.16241/j.cnki.1001-5914.2012.11.014
Wang J, Liu HL, Zeng Q et al (2012) Subacute toxicity of rapeseed genetically modified with bar gene in WZS minipigs. J Environ Health 29(11):980–984. https://doi.org/10.16241/j.cnki.1001-5914.2012.11.021
Wang J, Zhou C, Che HL et al (2010) Study on sub-chronic toxicity of powered milk containing transgenic lactoferrin on SD rats. Progr Mod Biomed 10(15):2809–2813. https://doi.org/10.13241/j.cnki.pmb.2010.15.002
Wang RY (2017) The effect of genetically modified feed on structure of mice testes. Livestock Poult Ind 28(06):6–7. https://doi.org/10.19567/j.cnki.1008-0414.2017.06.004
Wang R, Hu YC, Li M et al (2017) Study on the subchronic toxicity of transgenic DBN9978 herbicide resistant maize to rats. Food Nutr China 23(06):12–17
Wang XJ, Wang J, Liu HL et al (2012) Subchronic toxicity test of rice containing transgenic HJC-1 and G6-EPSPS in rats. J Environ Health 29(11):970–976. https://doi.org/10.16241/j.cnki.1001-5914.2012.11.019
Wang Y (2011) Food safety assessment of genetically modified milk with human lactoferrin gene. MS Thesis, Tianjin Medical University, Tianjin, China
Wang Y, Lai WQ, Chen JG et al (2000) Toxicity of anti-herbicide gene (BAR) transgenic rice. J Hyg Res 29(03):141–142
Wu JH (2013) The nutritional, edible safety and efficacy assessment of genetically modified rice with human lactoferrin gene and its purified protein and the nutritional assessment of genetically modified wheat expressing GmDREB/TaDREB4 genes with drought-resistance. Ph.D. Thesis, Chinese Center for Disease Control and Prevention, Beijing, China
Wu P, Su YL, Zhang J et al (2003) Safety evaluation of transgenic tomato against Cucumber Mosaic Virus. J Capital Univ Med Sci 24(03):254–258
Xu YJ (2012) The forage safety assessment of genetically modified organism corn to weaning piglets. M.S. Thesis, Fujian Agriculture and Forestry University, Fujian, China
Yu T, Liu Y, Wang JW et al (2017) Teratogenic test of recombinant human lactoferrin in rats. J Toxicol 31(03):247–250
Yuan JQ (2015) The detection of the transgenic GTS40-3-2 related genes and their products of livestock products sold and toxicology study of Sprague-Dawley rats ( Rattus norvegicus ). Ph.D. Thesis, Shanxi Agricultural University, Shanxi, China
Zhang L, Cheng C, He N et al (2011) Study on subchronic toxicity of transgenic soybean with high oleic acid on rats. J Toxicol 25(05):391–394. https://doi.org/10.16421/j.cnki.1002-3127.2011.05.012
Zhang L, Wang J, Jiang SQ et al (2016) Subchronic toxicity of genetically modified corn with Cry1Ab/Cry2Aj and G10evo (EPSPS) genes in rats. J Environ Health 33(07):585–589
Zhang LL (2018) The Unintended Effects of Long-Term Intergenerational Feeding Transgenic Maize Diets to Pure Line White Leghorn Chickens on the Intestinal Health. M.S. Thesis, Chinese Academy of Agricultural Sciences, Beijing, China
Zhang M, Zhuo Q, Tian Y et al (2012) Study on chronic toxicity of genetically modified rice expressing human lactoferrin. Chin J Food Hyg 24(06):391–394. https://doi.org/10.13590/j.cjfh.2012.06.008
Zhang Q (2014) Production of GH transgenic goat by somatic cell nuclear transfer. Ph.D. Thesis, Nanjing Agricultural University, Nanjing, China
Zhang WP, Li YY, Wang WG et al (2009) Detection of mutagenicity of chitinase and -1, 3 dextran gene in maize. Chin Remed Clin 9(05):405–407
Zhang ZY, Liu LJ, Zhang L et al (2010) Subchronic toxicity of Bt transgenic rice to mice. J Toxicol 24(02):126–129. https://doi.org/10.16421/j.cnki.1002-3127.2010.02.016
Zhao L, Zhang L, Zhang YY et al (2009) Immunotoxicological evaluation of transgenic soybean oil. China Health Care Nutr 11:5
Zhi Y, Liu HB, Di GY et al (2013) Genetic toxicity of transgenic human α-lactalbumin powdered milk. Carcinogen Teratogen Mutagen 25(02):124–133. https://doi.org/10.3969/j.issn.1004-616x.2013.02.010
Zhi Y, Liu HB, Di GY et al (2011) Study on sub-chronic toxicity of powered milk containing transgenic human α-lactalbumin. J Hyg Res 40(04):426–430. https://doi.org/10.19813/j.cnki.weishengyanjiu.2011.04.004
Zhong F (2013) Subchronic feeding study of transgenic BADH alfalfa on rabbits. M.S. Thesis, Shandong Agricultural University, Shandong, China
Zhou GL, Lu MJ, Chen YX et al (2007) Antisense LeETR1 transgenic tomato rats were fed for 4 weeks. J Toxicol 21(02):160–161
Zhou H (2012) Safety aeeseement of the phytase transgenic corn in the broiler diet. M.S. Thesis, Fujian Agriculture and Forestry University, Fujian, China
Zhou LG (2009) Research of high lysine transgenic paddy on broiler feed security. M.S. Thesis, Yangzhou University, Jiangsu, China
Zhou WL, Yang XF, Ping A et al (2014) Effects of transgenic Dwarf-mosaic-resistant maize on learning and memory abilities of rats. J Shanxi Agric Univ Nat Sci Ed 34(02):129–131. https://doi.org/10.13842/j.cnki.issn1671-8151.2014.02.006
Zhou XH (2012) Toxicological studies on the food safety of two transgenic rice. Ph.D. Thesis, Jiangsu University, Jiangsu, China
Zhou ZW, Wang DZ, Shen H et al (2012) Comprehensive evaluation on functions & safety of imported GM soybean using BDI-GS system. Soybean Sci 31(05):822–826
Zhu H, Zhu LY, Guo JY et al (2014) Safety evaluation of BT-799 corn on Wistar rats. Food Nutr China 20(09):63–67
Zhuo Q, Chen XP, Piao JH et al (2004) Experimental study on converting cowpea trypsin inhibitor rice for 90 days. J Hyg Res 33(02):176–179
Zhuo Q, Chen XP, Piao JH et al (2004) Study on teratogenic effect of cowpea trypsin inhibitor rice. J Hyg Res 33(01):74–77
Sakamoto Y, Tada Y, Fukumori N et al (2008) A 104-week feeding study of genetically modified soybeans in F344 rats. Shokuhin Eiseigaku Zasshi 49(4):272–282. https://doi.org/10.3358/shokueishi.49.272
Sakamoto Y, Tada Y, Fukumori N et al (2007) A 52-week feeding study of genetically modified soybeans in F344 rats. Shokuhin Eiseigaku Zasshi 48(3):41–50. https://doi.org/10.3358/shokueishi.48.41
Keshani P, Sharifi MH, Heydari MR et al (2020) The effect of genetically modified food on infertility indices: a systematic review study. Sci World J. https://doi.org/10.1155/2020/1424789
Edge MS, Kunkel ME, Schmidt J et al (2018) Evidence analysis library systematic review on advanced technology in food production. J Acad Nutr Diet 118(6):1106–1127. https://doi.org/10.1016/j.jand.2017.08.005
Dunn SE, Vicini JL, Glenn KC et al (2017) The allergenicity of genetically modified foods from genetically engineered crops: a narrative and systematic review. Ann Allergy Asthma Immunol 119(3):214–222. https://doi.org/10.1016/j.anai.2017.07.010
de Vos CJ, Swanenburg M (2018) Health effects of feeding genetically modified (GM) crops to livestock animals: a review. Food Chem Toxicol 117:3–12. https://doi.org/10.1016/j.fct.2017.08.031
Ricroch AE, Boisron A, Kuntz M (2014) Looking back at safety assessment of GM food/feed: an exhaustive review of 90-day animal feeding studies. Int J Biotechnology 13(4):230–256
Domingo Roig JL, Gómez Arnáiz M (2000) Riesgos sobre la salud de los alimentos modificados genéticamente: una revisión bibliográfica [Health risks of genetically modified foods: a literature review]. Revista espanola de salud publica 74(3):255–261
Domingo JL (2007) Toxicity studies of genetically modified plants: a review of the published literature. Crit Rev Food Sci Nutr 47(8):721–733. https://doi.org/10.1080/10408390601177670
Domingo JL, Giné Bordonaba J (2011) A literature review on the safety assessment of genetically modified plants. Environ Int 37(4):734–742. https://doi.org/10.1016/j.envint.2011.01.003
Teshima R, Watanabe T, Okunuki H et al (2002) Effect of subchronic feeding of genetically modified corn (CBH351) on immune system in BN rats and B10A mice. J Food Hyg Soc Jpn 43:273–279
Dubois AEJ, Pagliarani G, Brouwer RM et al (2015) First successful reduction of clinical allergenicity of food by genetic modification: Mal d 1-silenced apples cause fewer allergy symptoms than the wild-type cultivar. Allergy 70:1406–1412
Download references
Acknowledgements
We appreciate Yi-Zhen Li for participating in screening the titles and abstracts .
This work was supported by Innovation Team and Talents Cultivation Program of National Administration of Traditional Chinese Medicine (ZYYCXTD-C-202006). Prof. Nicola Robinson (visiting professor of Beijing University of Chinese Medicine) was funded by the International development and capacity enhancement of evidence-based Chinese medicine Project, Ministry of Science and Technology of the People's Republic of China, G20200001187.
Author information
Authors and affiliations.
Centre for Evidence-Based Chinese Medicine, Beijing University of Chinese Medicine, 11 Bei San Huan Dong Lu, Chaoyang District, Beijing, 100029, China
Chen Shen, Xiao-Wen Zhang, Wen-Bin Hou, Min Fang, Xun Li, Yu-Tong Fei, Nicola Robinson & Jian-Ping Liu
Beijing Institute of Radiation Medicine, Beijing, China
Xiang-Chang Yin
School of Chinese Medicine, Beijing University of Chinese Medicine, Beijing, China
Bo-Yang Jiao
Beijing Key Laboratory of the Innovative Development of Functional Staple and the Nutritional Intervention for Chronic Disease, China National Research Institute of Food & Fermentation Industries Co,. Ltd, Beijing, China
School of Life Sciences, Beijing University of Chinese Medicine, Beijing, China
School of Chinese Materia Medica, Beijing University of Chinese Medicine, Beijing, China
Xue-Hao Cheng & Jian-Xin Ren
Ruikang Hospital, Guangxi University of Chinese Medicine, Guangxi, China
School of Health and Social Care, London South Bank University, London, SE1 OAA, UK
Nicola Robinson
Institute for Excellence in Evidence-Based Chinese Medicine, Beijing University of Chinese Medicine, Beijing, China
Jian-Ping Liu
You can also search for this author in PubMed Google Scholar
Contributions
All work was done by the authors. JPL and YTF conceived the study and revised the manuscript. CS contributed to data searching, screening and extraction, analysis of the data, drafted and revised the paper and approved the final version to be submitted. XCY, BYJ, JP, XHC, JXR, JL, XWZ, HDL, WBH and MF participated in identifying or screening the titles, abstracts and full-text screening and data extraction. XL, NR and JPL advised on the analysis of the data and revised the manuscript. All authors read and approved the final manuscript.
Corresponding author
Correspondence to Jian-Ping Liu .
Ethics declarations
Ethics approval and consent to participate.
Not applicable.
Consent for publication
Competing interests.
The authors declared that the research was conducted in the absence of any commercial or financial relationships that could be constructed as a potential conflict of interest.
Additional information
Publisher's note.
Springer Nature remains neutral with regard to jurisdictional claims in published maps and institutional affiliations.
Supplementary Information
Additional file 1: appendix s1..
Search strategy applied in English language databases. Appendix S2. Funding sources or sponsors. Appendix S3. Adverse events/effects—other biomarkers.
Rights and permissions
Open Access This article is licensed under a Creative Commons Attribution 4.0 International License, which permits use, sharing, adaptation, distribution and reproduction in any medium or format, as long as you give appropriate credit to the original author(s) and the source, provide a link to the Creative Commons licence, and indicate if changes were made. The images or other third party material in this article are included in the article's Creative Commons licence, unless indicated otherwise in a credit line to the material. If material is not included in the article's Creative Commons licence and your intended use is not permitted by statutory regulation or exceeds the permitted use, you will need to obtain permission directly from the copyright holder. To view a copy of this licence, visit http://creativecommons.org/licenses/by/4.0/ .
Reprints and permissions
About this article
Cite this article.
Shen, C., Yin, XC., Jiao, BY. et al. Evaluation of adverse effects/events of genetically modified food consumption: a systematic review of animal and human studies. Environ Sci Eur 34 , 8 (2022). https://doi.org/10.1186/s12302-021-00578-9
Download citation
Received : 07 July 2021
Accepted : 11 December 2021
Published : 13 January 2022
DOI : https://doi.org/10.1186/s12302-021-00578-9
Share this article
Anyone you share the following link with will be able to read this content:
Sorry, a shareable link is not currently available for this article.
Provided by the Springer Nature SharedIt content-sharing initiative
- Adverse event
- Genetically modified food
- Evidence-based medicine
- Systematic review
Global Status of Genetically Modified Crops
- First Online: 08 January 2022
Cite this chapter
- Vipasha Verma 4 ,
- Shivanti Negi 4 ,
- Pankaj Kumar 4 &
- Dinesh Kumar Srivastava 4
2128 Accesses
4 Citations
1 Altmetric
The biggest problem the world is facing today is hunger, malnutrition, and the rising population. Adopting advanced breeding innovation technologies for enhanced agricultural production is urgently needed to meet the rising global demand for food and nutritional security. Recent advancements in GM technology, genome editing seem promising to accelerate crop improvement by enabling effective targeted modification in most crops/plants. Biosafety regulations for genome-modified plants are essential and often variable in various countries. In India, the GM Crops Release Regulation is one of the most regulated GM technologies in the world. The USA, Brazil, Argentina, Canada, and India planted about 91% of the global GM crop area of 190.4 million hectares. Soybean, maize, cotton, and canola are extensively grown and are of the utmost importance among all GM food crops. On six continents, approximately 32 plants were released for commercial cultivation. In five countries all over the world, 1.95 billion people have benefited from GM food biotechnology. This book chapter highlighted the global and Indian status of research and commercialization of GM crops, regulation of GM, and genome-edited technologies in India.
This is a preview of subscription content, log in via an institution to check access.
Access this chapter
- Available as PDF
- Read on any device
- Instant download
- Own it forever
- Available as EPUB and PDF
- Compact, lightweight edition
- Dispatched in 3 to 5 business days
- Free shipping worldwide - see info
- Durable hardcover edition
Tax calculation will be finalised at checkout
Purchases are for personal use only
Institutional subscriptions

Similar content being viewed by others
Genetically Modified Crops and Their Applications
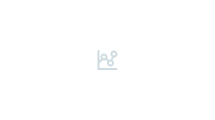
Benefits of genome-edited crops: expert opinion
Genetic Manipulation of Crop for Enhanced Food Quality and Nutrition Toward Sustainable Production
Abdallah N, Prakash C, McHughen A (2015) Genome editing for crop improvement: challenges and opportunities. GM Crops Food 6:183–205
Article PubMed Google Scholar
Altpeter F, Varshney A, Abderhalden O, Douchkov D, Sautter C, Kumlehn J, Dudler R, Schweizer P (2005) Stable expression of a defense-related gene in wheat epidermis under transcriptional control of a novel promoter confers pathogen resistance. Plant Mol Biol 57(2):271–283
Article CAS PubMed Google Scholar
Barrows G, Sexton S, Zilberman D (2014) The impact of agricultural biotechnology on supply and land-use. Environ Dev Econ 19(6):676–703
Article Google Scholar
Bhattacharya S, Sengupta S, Karmakar A, Sarkar SN, Gangopadhyay G, Datta K, Datta SK (2019) Genetically engineered rice with appA gene enhanced phosphorus and minerals. J Plant Biochem Biotechnol 28(4):470–482
Article CAS Google Scholar
Bortesi L, Fischer R (2015) The CRISPR/Cas9 system for plant genome editing and beyond. Biotechnol Adv 33(1):41–52
Bruening G, Lyons J (2000) The case of the FLAVR SAVR tomato. California Agric 54(4):6–7
Čermák T, Baltes NJ, Čegan R, Zhang Y, Voytas DF (2015) High-frequency, precise modification of the tomato genome. Genome Biol 16(1):1–15
Chandrasekaran J, Brumin M, Wolf D, Leibman D, Klap C, Pearlsman M, Sherman A, Arazi T, Gal-On A (2016) Development of broad virus resistance in non-transgenic cucumber using CRISPR/Cas9 technology. Mol Plant Pathol 17(7):1140–1153
Article CAS PubMed PubMed Central Google Scholar
Choudhary B, Gaur K (2015) Biotech cotton in India, 2002 to 2014. In: ISAAA series of biotech crop profiles. ISAAA, Ithaca, pp 1–34
Google Scholar
Datta K, Baisakh N, Oliva N, Torrizo L, Abrigo E, Tan J, Rai M, Rehana S, Al-Babili S, Beyer P (2003) Bioengineered ‘golden’ indica rice cultivars with β-carotene metabolism in the endosperm with hygromycin and mannose selection systems. Plant Biotechnol J 1(2):81–90
Datta K, Koukolikova-Nicola Z, Baisakh N, Oliva N, Datta S (2000) Agrobacterium -mediated engineering for sheath blight resistance of India rice cultivars from different ecosystems. Theor Appl Genet 100(6):832–839
FAO (2012) Genetically modified crops, part 4. http:// www.fao.org/docrep/015/i2490e/i2490e04d.pdf
FAO (2014) Report of the regional workshop on strengthening regional cooperation and national capacity building on biosafety in Asia. http://www.fao.org/3/a-i3902e.pdf
Frewer LJ, van der Lans IA, Fischer AR, Reinders MJ, Menozzi D, Zhang X, van den Berg I, Zimmermann KL (2013) Public perceptions of agri-food applications of genetic modification–a systematic review and meta-analysis. Trends Food Sci Technol 30(2):142–152
Friedrichs S, Takasu Y, Kearns P, Dagallier B, Oshima R, Schofield J, Moreddu C (2019) An overview of regulatory approaches to genome editing in agriculture. Biotechnol Res Innov 3(2):208–220
Gaj T, Sirk SJ, S-l S, Liu J (2016) Genome-editing technologies: principles and applications. Cold Spring Harb Perspect Biol 8(12):a023754
Article PubMed PubMed Central Google Scholar
George S, Venkataraman G, Parida A (2007) Identification of stress-induced genes from the drought-tolerant plant Prosopis juliflora (Swartz) DC. through analysis of expressed sequence tags. Genome 50(5):470–478
Gupta V, Ahuja V (2016) Challenges for developing a regulatory dossier by public sector developers in developing countries. http://ilsirf.org/wp-content/uploads/sites/5/2016/06/gupta_ahuja.pdf
Herring RJ (2014) On risk and regulation: Bt crops in India. GM Crops Food 5(3):204–209
Indian Ministry of Science and Technology (2020) Draft document on genome edited organisms: regulatory framework and guidelines for risk assessment. Indian Ministry of Science and Technology, New Delhi
ISAAA (2019) Global Status of Commercialized Biotech/GM Crops in 2019: biotech crops drive socio-economic development and sustainable environment in the new frontier. Executive Summary ISAAA Brief 55. http://www.isaaa.org/
James C (2011) Brief 42 global status of commercialized biotech/GM crops: 2010. ISAAA Brief 44
James C (2014) ISAAA briefs brief 49 global status of commercialized biotech/GM crops: 2014
Jyothi-Prakash PA, Mohanty B, Wijaya E, Lim T-M, Lin Q, Loh C-S, Kumar PP (2014) Identification of salt gland-associated genes and characterization of a dehydrin from the salt secretor mangrove Avicennia officinalis . BMC Plant Biol 14(1):1–16
Kandasamy M, Padmavati M (2014) Transgenic crop research and regulation in India: whether legislation rightly drives the motion? J Commer Biotechnol 20:4
Karthik K, Nandiganti M, Thangaraj A, Singh S, Mishra P, Rathinam M, Sharma M, Singh NK, Dash PK, Sreevathsa R (2020) Transgenic cotton ( Gossypium hirsutum L.) to combat weed vagaries: utility of an apical meristem-targeted in planta transformation strategy to introgress a modified CP4-EPSPS gene for glyphosate tolerance. Front Plant Sci 11
Kumar K, Gambhir G, Dass A, Tripathi AK, Singh A, Jha AK, Yadava P, Choudhary M, Rakshit S (2020) Genetically modified crops: current status and future prospects. Planta 251(4):1–27
Langner T, Kamoun S, Belhaj K (2018) CRISPR crops: plant genome editing toward disease resistance. Annu Rev Phytopathol 56:479–512
Li J-F, Norville JE, Aach J, McCormack M, Zhang D, Bush J, Church GM, Sheen J (2013) Multiplex and homologous recombination–mediated genome editing in Arabidopsis and Nicotiana benthamiana using guide RNA and Cas9. Nat Biotechnol 31(8):688–691
Liang Z, Zhang K, Chen K, Gao C (2014) Targeted mutagenesis in Zea mays using TALENs and the CRISPR/Cas system. J Genet Genomics 41(2):63–68
McHughen A, Smyth S (2008) US regulatory system for genetically modified [genetically modified organism (GMO), rDNA or transgenic] crop cultivars. Plant Biotechnol J 6(1):2–12
PubMed Google Scholar
Menz J, Modrzejewski D, Hartung F, Wilhelm R, Sprink T (2020) Genome edited crops touch the market: a view on the global development and regulatory environment. Frontiers Pant Sci:11
Miao J, Guo D, Zhang J, Huang Q, Qin G, Zhang X, Wan J, Gu H, Qu L-J (2013) Targeted mutagenesis in rice using CRISPR-Cas system. Cell Res 23(10):1233–1236
MoEF Notification (1989) Rules for the manufacture, use, import, export and storage of hazardous microorganisms, genetically engineered organisms or cells. http://envfor.nic.in/legis/hsm/hsm3.html . Accessed 5 Dec 1989
Molla KA, Yang Y (2019) CRISPR/Cas-mediated base editing: technical considerations and practical applications. Trends Biotechnol 37(10):1121–1142
NAAS (2020) Genome edited plants: accelerating the pace and precision of plant breeding. Policy brief no. 7. National Academy of Agricultural Sciences, New Delhi, pp 1–16
Nalluri N, Karri VR (2020) Recent advances in genetic manipulation of crops: a promising approach to address the global food and industrial applications. Plant Sci Today 7(1):70–92
Nekrasov V, Staskawicz B, Weigel D, Jones JD, Kamoun S (2013) Targeted mutagenesis in the model plant Nicotiana benthamiana using Cas9 RNA-guided endonuclease. Nat Biotechnol 31(8):691–693
Oliva R, Ji C, Atienza-Grande G, Huguet-Tapia JC, Perez-Quintero A, Li T, Eom J-S, Li C, Nguyen H, Liu B (2019) Broad-spectrum resistance to bacterial blight in rice using genome editing. Nat Biotechnol 37(11):1344–1350
Raman R (2017) The impact of genetically modified (GM) crops in modern agriculture: a review. GM Crops Food 8(4):195–208
Ramkumar N, Rathinam M, Singh S, Kesiraju K, Muniyandi V, Singh NK, Dash PK, Sreevathsa R (2020) Assessment of Pigeonpea ( Cajanus cajan L.) transgenics expressing Bt ICPs, Cry2Aa and Cry1AcF under nethouse containment implicated an effective control against herbivory by Helicoverpa armigera (Hübner). Pest Manag Sci 76(5):1902–1911
Ricroch A (2019) Global developments of genome editing in agriculture. In: Transgenic research, vol 2. Springer, Berlin, pp 45–52
Roberts RJ (2018) The Nobel laureates’ campaign supporting GMOs. J Innov Knowl 3(2):61–65
Sahrawat AK, Becker D, Lütticke S, Lörz H (2003) Genetic improvement of wheat via alien gene transfer, an assessment. Plant Sci 165(5):1147–1168
San-Epifanio LE (2017) Towards a new regulatory framework for GM crops in the European Union: scientific, ethical, social and legal issues and the challenges ahead. Academic Publishers, Wageningen
Book Google Scholar
Shi J, Gao H, Wang H, Lafitte HR, Archibald RL, Yang M, Hakimi SM, Mo H, Habben JE (2017) ARGOS 8 variants generated by CRISPR-Cas9 improve maize grain yield under field drought stress conditions. Plant Biotechnol J 15(2):207–216
Sinebo W, Maredia K (2016) Innovative farmers and regulatory gatekeepers: genetically modified crops regulation and adoption in developing countries. GM Crops Food 7(1):1–11
Smith C, Watson C, Ray J, Bird C, Morris P, Schuch W, Grierson D (1988) Antisense RNA inhibition of polygalacturonase gene expression in transgenic tomatoes. Nature 334(6184):724–726
Svitashev S, Young JK, Schwartz C, Gao H, Falco SC, Cigan AM (2015) Targeted mutagenesis, precise gene editing, and site-specific gene insertion in maize using Cas9 and guide RNA. Plant Physiol 169(2):931–945
The Guardian (2013) GM crops: the British public still need convincing. http://www.theguardian.com/environment/blog/2013/jun/20/owen-paterson-gm
The Independent (2015) The GM crops debate moves to Africa - and it’s just as noisy. http://www.independent.co.uk/news/world/africa/the-gm-crops-debate-moves-to-africa–and-its-justas-noisy-10126512.html
The New York Times (2013) Strong support for labeling modified foods. http://www.nytimes.com/2013/07/28/science/strong-support-for-labeling-modified-foods.html?_r=1
Thomazella D, Brail Q, Dahlbeck D, Staskawic Z (2016) CRISPR-Cas9 mediated mutagenesis of a DMR6 ortholog in tomato confers broad-spectrum disease resistance. bioRxiv preprint
Transkript (2018) Bundesrat Setzt Genscheren auf Agenda. https://transkript.de/meldungen-des-tages/detail/bundesrat-setztgenscheren-auf-agenda.html . Accessed 10 Oct 2019
Tsatsakis AM, Nawaz MA, Kouretas D, Balias G, Savolainen K, Tutelyan VA, Golokhvast KS, Lee JD, Yang SH, Chung G (2017) Environmental impacts of genetically modified plants: a review. Environ Res 156:818–833
Waltz E (2016) CRISPR-edited crops free to enter market, skip regulation. Nature 4:582
Wang Y, Cheng X, Shan Q, Zhang Y, Liu J, Gao C, Qiu J-L (2014) Simultaneous editing of three homoeoalleles in hexaploid bread wheat confers heritable resistance to powdery mildew. Nat Biotechnol 32(9):947–951
Warrier R, Pande H (2016) Genetically engineered plants in the product development pipeline in India. GM Crops Food 7(1):12–19
Zhang Y, Massel K, Godwin ID, Gao C (2018) Applications and potential of genome editing in crop improvement. Genome Biol 19(1):1–11
Download references
Author information
Authors and affiliations.
Dr Y.S. Parmar University of Horticulture and Forestry, Solan, Himachal Pradesh, India
Vipasha Verma, Shivanti Negi, Pankaj Kumar & Dinesh Kumar Srivastava
You can also search for this author in PubMed Google Scholar
Corresponding author
Correspondence to Pankaj Kumar .
Editor information
Editors and affiliations.
Department of Biotechnology, Dr. Yashwant Singh Parmar University of Horticulture and Forestry, Solan, Himachal Pradesh, India
Dinesh Kumar Srivastava
Biotechnology Division, ICAR-Directorate of Rapeseed-Mustard Research, Bharatpur, Rajasthan, India
Ajay Kumar Thakur
Pankaj Kumar
Rights and permissions
Reprints and permissions
Copyright information
© 2021 The Author(s), under exclusive license to Springer Nature Singapore Pte Ltd.
About this chapter
Verma, V., Negi, S., Kumar, P., Srivastava, D.K. (2021). Global Status of Genetically Modified Crops. In: Kumar Srivastava, D., Kumar Thakur, A., Kumar, P. (eds) Agricultural Biotechnology: Latest Research and Trends . Springer, Singapore. https://doi.org/10.1007/978-981-16-2339-4_13
Download citation
DOI : https://doi.org/10.1007/978-981-16-2339-4_13
Published : 08 January 2022
Publisher Name : Springer, Singapore
Print ISBN : 978-981-16-2338-7
Online ISBN : 978-981-16-2339-4
eBook Packages : Biomedical and Life Sciences Biomedical and Life Sciences (R0)
Share this chapter
Anyone you share the following link with will be able to read this content:
Sorry, a shareable link is not currently available for this article.
Provided by the Springer Nature SharedIt content-sharing initiative
- Publish with us
Policies and ethics
- Find a journal
- Track your research
This page has been archived and is no longer updated
Genetically Modified Organisms (GMOs): Transgenic Crops and Recombinant DNA Technology

People have been altering the genomes of plants and animals for many years using traditional breeding techniques. Artificial selection for specific, desired traits has resulted in a variety of different organisms, ranging from sweet corn to hairless cats. But this artificial selection , in which organisms that exhibit specific traits are chosen to breed subsequent generations, has been limited to naturally occurring variations. In recent decades, however, advances in the field of genetic engineering have allowed for precise control over the genetic changes introduced into an organism . Today, we can incorporate new genes from one species into a completely unrelated species through genetic engineering, optimizing agricultural performance or facilitating the production of valuable pharmaceutical substances. Crop plants, farm animals, and soil bacteria are some of the more prominent examples of organisms that have been subject to genetic engineering.
Current Use of Genetically Modified Organisms
Table 1: Examples of GMOs Resulting from Agricultural Biotechnology
The pharmaceutical industry is another frontier for the use of GMOs. In 1986, human growth hormone was the first protein pharmaceutical made in plants (Barta et al ., 1986), and in 1989, the first antibody was produced (Hiatt et al ., 1989). Both research groups used tobacco, which has since dominated the industry as the most intensively studied and utilized plant species for the expression of foreign genes (Ma et al ., 2003). As of 2003, several types of antibodies produced in plants had made it to clinical trials. The use of genetically modified animals has also been indispensible in medical research. Transgenic animals are routinely bred to carry human genes, or mutations in specific genes, thus allowing the study of the progression and genetic determinants of various diseases.
Potential GMO Applications
Many industries stand to benefit from additional GMO research. For instance, a number of microorganisms are being considered as future clean fuel producers and biodegraders. In addition, genetically modified plants may someday be used to produce recombinant vaccines. In fact, the concept of an oral vaccine expressed in plants (fruits and vegetables) for direct consumption by individuals is being examined as a possible solution to the spread of disease in underdeveloped countries, one that would greatly reduce the costs associated with conducting large-scale vaccination campaigns. Work is currently underway to develop plant-derived vaccine candidates in potatoes and lettuce for hepatitis B virus (HBV), enterotoxigenic Escherichia coli (ETEC), and Norwalk virus. Scientists are also looking into the production of other commercially valuable proteins in plants, such as spider silk protein and polymers that are used in surgery or tissue replacement (Ma et al ., 2003). Genetically modified animals have even been used to grow transplant tissues and human transplant organs, a concept called xenotransplantation. The rich variety of uses for GMOs provides a number of valuable benefits to humans, but many people also worry about potential risks.
Risks and Controversies Surrounding the Use of GMOs
Despite the fact that the genes being transferred occur naturally in other species, there are unknown consequences to altering the natural state of an organism through foreign gene expression . After all, such alterations can change the organism's metabolism , growth rate, and/or response to external environmental factors. These consequences influence not only the GMO itself, but also the natural environment in which that organism is allowed to proliferate. Potential health risks to humans include the possibility of exposure to new allergens in genetically modified foods, as well as the transfer of antibiotic-resistant genes to gut flora.
Horizontal gene transfer of pesticide, herbicide, or antibiotic resistance to other organisms would not only put humans at risk , but it would also cause ecological imbalances, allowing previously innocuous plants to grow uncontrolled, thus promoting the spread of disease among both plants and animals. Although the possibility of horizontal gene transfer between GMOs and other organisms cannot be denied, in reality, this risk is considered to be quite low. Horizontal gene transfer occurs naturally at a very low rate and, in most cases, cannot be simulated in an optimized laboratory environment without active modification of the target genome to increase susceptibility (Ma et al ., 2003).
In contrast, the alarming consequences of vertical gene transfer between GMOs and their wild-type counterparts have been highlighted by studying transgenic fish released into wild populations of the same species (Muir & Howard, 1999). The enhanced mating advantages of the genetically modified fish led to a reduction in the viability of their offspring . Thus, when a new transgene is introduced into a wild fish population, it propagates and may eventually threaten the viability of both the wild-type and the genetically modified organisms.
Unintended Impacts on Other Species: The Bt Corn Controversy
One example of public debate over the use of a genetically modified plant involves the case of Bt corn. Bt corn expresses a protein from the bacterium Bacillus thuringiensis . Prior to construction of the recombinant corn, the protein had long been known to be toxic to a number of pestiferous insects, including the monarch caterpillar, and it had been successfully used as an environmentally friendly insecticide for several years. The benefit of the expression of this protein by corn plants is a reduction in the amount of insecticide that farmers must apply to their crops. Unfortunately, seeds containing genes for recombinant proteins can cause unintentional spread of recombinant genes or exposure of non-target organisms to new toxic compounds in the environment.
The now-famous Bt corn controversy started with a laboratory study by Losey et al . (1999) in which the mortality of monarch larvae was reportedly higher when fed with milkweed (their natural food supply) covered in pollen from transgenic corn than when fed milkweed covered with pollen from regular corn. The report by Losey et al . was followed by another publication (Jesse & Obrycki, 2000) suggesting that natural levels of Bt corn pollen in the field were harmful to monarchs.
Debate ensued when scientists from other laboratories disputed the study, citing the extremely high concentration of pollen used in the laboratory study as unrealistic, and concluding that migratory patterns of monarchs do not place them in the vicinity of corn during the time it sheds pollen. For the next two years, six teams of researchers from government, academia, and industry investigated the issue and concluded that the risk of Bt corn to monarchs was "very low" (Sears et al ., 2001), providing the basis for the U.S. Environmental Protection Agency to approve Bt corn for an additional seven years.
Unintended Economic Consequences
Another concern associated with GMOs is that private companies will claim ownership of the organisms they create and not share them at a reasonable cost with the public. If these claims are correct, it is argued that use of genetically modified crops will hurt the economy and environment, because monoculture practices by large-scale farm production centers (who can afford the costly seeds) will dominate over the diversity contributed by small farmers who can't afford the technology. However, a recent meta-analysis of 15 studies reveals that, on average, two-thirds of the benefits of first-generation genetically modified crops are shared downstream, whereas only one-third accrues upstream (Demont et al ., 2007). These benefit shares are exhibited in both industrial and developing countries. Therefore, the argument that private companies will not share ownership of GMOs is not supported by evidence from first-generation genetically modified crops.
GMOs and the General Public: Philosophical and Religious Concerns
In a 2007 survey of 1,000 American adults conducted by the International Food Information Council (IFIC), 33% of respondents believed that biotech food products would benefit them or their families, but 23% of respondents did not know biotech foods had already reached the market. In addition, only 5% of those polled said they would take action by altering their purchasing habits as a result of concerns associated with using biotech products.
According to the Food and Agriculture Organization of the United Nations, public acceptance trends in Europe and Asia are mixed depending on the country and current mood at the time of the survey (Hoban, 2004). Attitudes toward cloning, biotechnology, and genetically modified products differ depending upon people's level of education and interpretations of what each of these terms mean. Support varies for different types of biotechnology; however, it is consistently lower when animals are mentioned.
Furthermore, even if the technologies are shared fairly, there are people who would still resist consumable GMOs, even with thorough testing for safety, because of personal or religious beliefs. The ethical issues surrounding GMOs include debate over our right to "play God," as well as the introduction of foreign material into foods that are abstained from for religious reasons. Some people believe that tampering with nature is intrinsically wrong, and others maintain that inserting plant genes in animals, or vice versa, is immoral. When it comes to genetically modified foods, those who feel strongly that the development of GMOs is against nature or religion have called for clear labeling rules so they can make informed selections when choosing which items to purchase. Respect for consumer choice and assumed risk is as important as having safeguards to prevent mixing of genetically modified products with non-genetically modified foods. In order to determine the requirements for such safeguards, there must be a definitive assessment of what constitutes a GMO and universal agreement on how products should be labeled.
These issues are increasingly important to consider as the number of GMOs continues to increase due to improved laboratory techniques and tools for sequencing whole genomes, better processes for cloning and transferring genes, and improved understanding of gene expression systems. Thus, legislative practices that regulate this research have to keep pace. Prior to permitting commercial use of GMOs, governments perform risk assessments to determine the possible consequences of their use, but difficulties in estimating the impact of commercial GMO use makes regulation of these organisms a challenge.
History of International Regulations for GMO Research and Development
In 1971, the first debate over the risks to humans of exposure to GMOs began when a common intestinal microorganism, E. coli , was infected with DNA from a tumor-inducing virus (Devos et al ., 2007). Initially, safety issues were a concern to individuals working in laboratories with GMOs, as well as nearby residents. However, later debate arose over concerns that recombinant organisms might be used as weapons. The growing debate, initially restricted to scientists, eventually spread to the public, and in 1974, the National Institutes of Health (NIH) established the Recombinant DNA Advisory Committee to begin to address some of these issues.
In the 1980s, when deliberate releases of GMOs to the environment were beginning to occur, the U.S. had very few regulations in place. Adherence to the guidelines provided by the NIH was voluntary for industry. Also during the 1980s, the use of transgenic plants was becoming a valuable endeavor for production of new pharmaceuticals, and individual companies, institutions, and whole countries were beginning to view biotechnology as a lucrative means of making money (Devos et al ., 2007). Worldwide commercialization of biotech products sparked new debate over the patentability of living organisms, the adverse effects of exposure to recombinant proteins, confidentiality issues, the morality and credibility of scientists, the role of government in regulating science, and other issues. In the U.S., the Congressional Office of Technology Assessment initiatives were developed, and they were eventually adopted worldwide as a top-down approach to advising policymakers by forecasting the societal impacts of GMOs.
Then, in 1986, a publication by the Organization for Economic Cooperation and Development (OECD), called "Recombinant DNA Safety Considerations," became the first intergovernmental document to address issues surrounding the use of GMOs. This document recommended that risk assessments be performed on a case-by-case basis. Since then, the case-by-case approach to risk assessment for genetically modified products has been widely accepted; however, the U.S. has generally taken a product-based approach to assessment, whereas the European approach is more process based (Devos et al ., 2007). Although in the past, thorough regulation was lacking in many countries, governments worldwide are now meeting the demands of the public and implementing stricter testing and labeling requirements for genetically modified crops.
Increased Research and Improved Safety Go Hand in Hand
Proponents of the use of GMOs believe that, with adequate research, these organisms can be safely commercialized. There are many experimental variations for expression and control of engineered genes that can be applied to minimize potential risks. Some of these practices are already necessary as a result of new legislation, such as avoiding superfluous DNA transfer (vector sequences) and replacing selectable marker genes commonly used in the lab (antibiotic resistance) with innocuous plant-derived markers (Ma et al ., 2003). Issues such as the risk of vaccine-expressing plants being mixed in with normal foodstuffs might be overcome by having built-in identification factors, such as pigmentation, that facilitate monitoring and separation of genetically modified products from non-GMOs. Other built-in control techniques include having inducible promoters (e.g., induced by stress, chemicals, etc.), geographic isolation, using male-sterile plants, and separate growing seasons.
GMOs benefit mankind when used for purposes such as increasing the availability and quality of food and medical care, and contributing to a cleaner environment. If used wisely, they could result in an improved economy without doing more harm than good, and they could also make the most of their potential to alleviate hunger and disease worldwide. However, the full potential of GMOs cannot be realized without due diligence and thorough attention to the risks associated with each new GMO on a case-by-case basis.
References and Recommended Reading
Barta, A., et al . The expression of a nopaline synthase-human growth hormone chimaeric gene in transformed tobacco and sunflower callus tissue. Plant Molecular Biology 6 , 347–357 (1986)
Beyer, P., et al . Golden rice: Introducing the β-carotene biosynthesis pathway into rice endosperm by genetic engineering to defeat vitamin A deficiency. Journal of Nutrition 132 , 506S–510S (2002)
Demont, M., et al . GM crops in Europe: How much value and for whom? EuroChoices 6 , 46–53 (2007)
Devlin, R., et al . Extraordinary salmon growth. Nature 371 , 209–210 (1994) ( link to article )
Devos, Y., et al . Ethics in the societal debate on genetically modified organisms: A (re)quest for sense and sensibility. Journal of Agricultural and Environmental Ethics 21 , 29–61 (2007) doi:10.1007/s10806-007-9057-6
Guerrero-Andrade, O., et al . Expression of the Newcastle disease virus fusion protein in transgenic maize and immunological studies. Transgenic Research 15 , 455–463(2006) doi:10.1007/s11248-006-0017-0
Hiatt, A., et al . Production of antibodies in transgenic plants. Nature 342 , 76–79 (1989) ( link to article )
Hoban, T. Public attitudes towards agricultural biotechnology. ESA working papers nos. 4-9. Agricultural and Development Economics Division, Food and Agricultural Organization of the United Nations (2004)
Jesse, H., & Obrycki, J. Field deposition of Bt transgenic corn pollen: Lethal effects on the monarch butterfly. Oecologia 125 , 241–248 (2000)
Losey, J., et al . Transgenic pollen harms monarch larvae. Nature 399 , 214 (1999) doi:10.1038/20338 ( link to article )
Ma, J., et al . The production of recombinant pharmaceutical proteins in plants. Nature Reviews Genetics 4 , 794–805 (2003) doi:10.1038/nrg1177 ( link to article )
Muir, W., & Howard, R. Possible ecological risks of transgenic organism release when transgenes affect mating success: Sexual selection and the Trojan gene hypothesis. Proceedings of the National Academy of Sciences 96 , 13853–13856 (1999)
Sears, M., et al . Impact of Bt corn on monarch butterfly populations: A risk assessment. Proceedings of the National Academy of Sciences 98 , 11937–11942 (2001)
Spurgeon, D. Call for tighter controls on transgenic foods. Nature 409 , 749 (2001) ( link to article )
Takeda, S., & Matsuoka, M. Genetic approaches to crop improvement: Responding to environmental and population changes. Nature Reviews Genetics 9 , 444–457 (2008) doi:10.1038/nrg2342 ( link to article )
United States Department of Energy, Office of Biological and Environmental Research, Human Genome Program. Human Genome Project information: Genetically modified foods and organisms, (2007)
- Add Content to Group
Article History
Flag inappropriate.


Email your Friend

- | Lead Editor: Bob Moss

Within this Subject (34)
- Applications in Biotechnology (4)
- Discovery of Genetic Material (4)
- DNA Replication (6)
- Gene Copies (5)
- Jumping Genes (4)
- RNA (7)
- Transcription & Translation (4)
Other Topic Rooms
- Gene Inheritance and Transmission
- Gene Expression and Regulation
- Nucleic Acid Structure and Function
- Chromosomes and Cytogenetics
- Evolutionary Genetics
- Population and Quantitative Genetics
- Genes and Disease
- Genetics and Society
- Cell Origins and Metabolism
- Proteins and Gene Expression
- Subcellular Compartments
- Cell Communication
- Cell Cycle and Cell Division

© 2014 Nature Education
- Press Room |
- Terms of Use |
- Privacy Notice |

Visual Browse
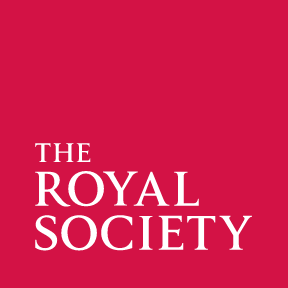
What new GM crops are being developed?
GM crops are being developed to be more disease-resistant, to have enhanced nutritional value, increased drought tolerance and improved uptake of nutrients such as nitrogen. They are being tested in the laboratory or in contained field trials – in which plants are grown in an area to prevent spread into the environment.
Crop disease is a big problem for farmers and GM can be used to produce disease resistant plants. Disease resistance genes from wild relatives can be transferred into commercial crops using GM. For example there have recently been very promising field trials of a GM blight-resistant potato. A gene from a wild relative in South America has been introduced which triggers the potatoes’ immune system to recognise blight. The potatoes have not been commercialised yet and would be labelled GM if they were in supermarkets.
GM can also be used to enhance the nutritional value of crops in the human or animal diet. The Golden Rice project, to make rice a source of vitamin A, is one such example. The World Health Organization estimates that up to half a million children become blind every year due to Vitamin A deficiency and Vitamin A deficiency can also reduce resilience to infection. Golden Rice is in field trials in the Philippines and Bangladesh and is completing the regulatory requirements in these two countries.
GM crops are also being developed to help decrease pollution of rivers and seas. One such crop aims to reduce the environmental impact of manure. Cereal and grain based animal feed often contains seeds with high levels of a compound called phytic acid, which passes into manure undigested. This can contaminate soil and waterways like rivers with phosphate, which harms fish and aquatic life. GM has been used to produce seeds low in phytic acid content so that this pollution risk could be reduced.
There are other long term GM projects that aim to produce nitrogen fixing cereals (plants that will harness nitrogen from the air as a nutrient), improve the efficiency of photosynthesis and produce perennial crops that would not need planting each year. Examples of both medium and long term projects are described in more detail in a recent report by the Council for Science and Technology.
‘Making farmed fish more nutritious’
An example of the use of GM to enhance the nutritional value of food involves the “good fats” we normally obtain from eating oily fish like salmon. These long chain omega-3 fatty acids are healthy for the heart and brain.
Fish need these Omega-3 fatty acids to stay healthy but do not produce them naturally themselves. They get them from marine algae that are eaten by small fish and passed up the food chain. Farmed fish consume large quantities of fish oils mainly through fish meal and it is possible that conventional sources of fish oil may not be able to meet future demand.
Scientists have used GM to transfer the algal genes that make these fatty acids into oilseed crops. These could be fed to farmed fish and in the longer term, the fortified crops could be used to supplement other foods with omega-3 fatty acids for human consumption.
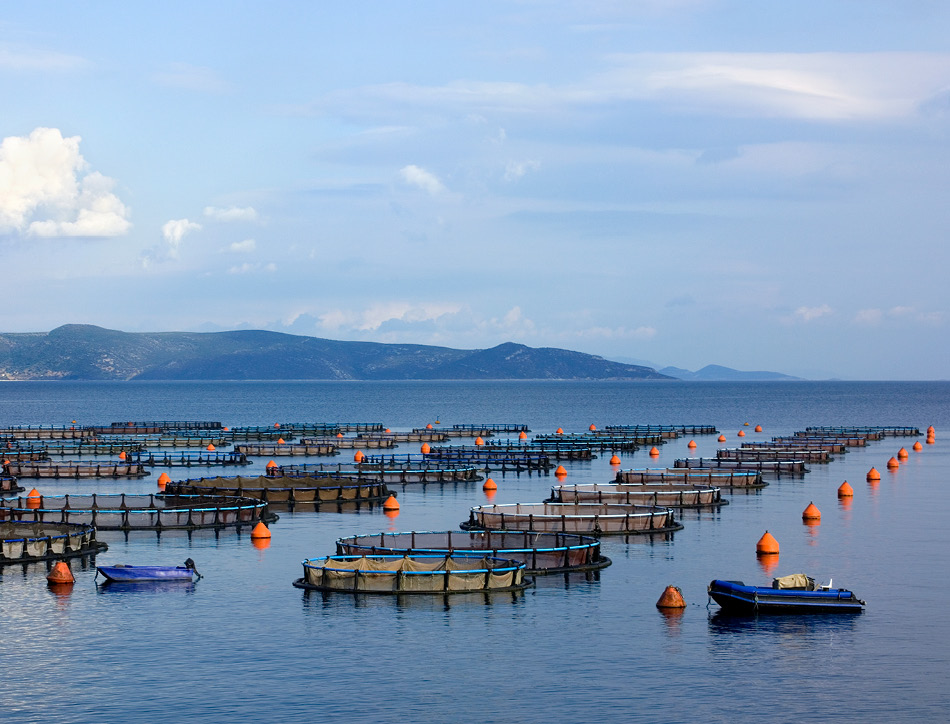
See related questions
- Which genes have been introduced into GM crops so far and why?
- Are there examples where GM has not delivered the promised improvements in crops?
- What methods other than genetic improvement can improve crop performance?
Page last updated: May 2016
Download all questions and answers (PDF)
Genetic technologies
Reading, interpreting and editing genetic material is getting faster, cheaper, and more precise. Using the power of genetic technologies in living things has enormous implications and raises important ethical issues for discussion, so it is important they are used appropriately and society is involved in the big decisions about their use.
Email updates
We promote excellence in science so that, together, we can benefit humanity and tackle the biggest challenges of our time.
Subscribe to our newsletters to be updated with the latest news on innovation, events, articles and reports.
What subscription are you interested in receiving? (Choose at least one subject)
[X] CLOSE MAIN MENU
- Our Mission
- Board Members
- Annual Report & Giving
- Agricultural Justice
- BEE Protective: Pollinators and Pesticides
- Biodiversity
- Center for Community Pesticide and Alternatives Information
- Children and Schools
- Fund for Independent Science
- Invasive Weed Management
- National Pesticide Forum
- Organic Agriculture
- Rodenticides
- Wood Preservatives
- Beyond Pesticides Resources
- Parks for a Sustainable Future
- Non-Toxic Lawns and Landscapes
- 40 Common Garden Pesticides
- ManageSafe�
- Golf, Pesticides, and Organic Practices
- Safer Mosquito Management
- Safety Source on Pest Management Providers
- Be Aware: Contaminated Waters
- Eating with a Conscience
- Pesticide-Induced Diseases Database
- Breast Cancer Prevention
- Disproportionate Risks
- Gateway on Pesticide Hazards and Safe Pest Management
- Herbicide Analysis
- Safer Sanitizers and Disinfectants
- Pets and Pesticides
- The Safer Choice
- Healthy Healthcare
- Pesticide Emergencies
- U.S. Pesticide Reform Policies
- State Regulations
- Pesticides and You " > Pesticides and You
- News & Press
- Spring Into Action
- EPA Contacts
- State Agency Contacts
- Fill Out an Incident Report
- Find an Attorney
- Daily News Blog
- Become a Member!
- Action Alerts Sign-up
- Please Help Sustain Our Critical Work in 2024�Give Today!
- Gift Memberships
- Take Action
- BEE Protective
- BEE Protective Ambassadors
- Polli-NATION
- Seeds That Poison
- Pollinator Week
- Resources and Educational Materials
- What Can You Do?
- What the Science Shows
- Chemicals Implicated
- Regulatory Action
- Press Releases and Media
- Pollinator Curriculum
- Have a Pest Problem?
- Pesticide-Free Holidays
- Hazards of Pesticides
- Alternatives at Schools
- Model Policies for State and Local Action
- Publications and Reports
- 2023 National Forum Series
- Past Conferences
- Forum Videos
- Why Organic?
- Save Our Organic
- Keeping Organic Strong
- Buying Organic Products
- Growing Your Own Organic Food
- Celebrate Organic
- Pandemic Resources for Farmers
- Companies that Care
- Organizational Sign-ons
- Amphibians/Reptiles
- Fish and Other Aquatics
- Pollinators
- Non-Target Insects and Beneficial Species
- Publications
- Become a Parks Advocate
- Municipal Officials
- In Your Community
- Tools for Change
- Choose a Pest
- Environmental Principles
- Green Your Local Course
- Find a Service Provider
- Talking to Service Providers
- What Is Integrated Pest Management?
- Pest Management Providers: Register Today!
- Regulatory Issues
- Choose a Crop
- Farmworkers
- Methodology
- Search the Database
- Asthma and Respiratory Effects
- Birth Defects
- Body Burden
- Brain and Nervous System Disorders
- Endocrine Disruption
- Immune System Disorders
- Learning/Developmental
- Sexual and Reproductive Dysfunction
- Be Aware: Genetic Engineering
- About the Gateway
- What Is a Pesticide?
- Disinfectants and Sanitizers
- Information for New Parents
- Drawing, Paintings, and Digital Art
- Writing (e.g., Poems, Music, Short Stories)
- Pesticides and You
- Rodale Institute Honors 2021 Organic Pioneer Award Winners
- EPA's Pesticides Office Labeled as a Failure
- Beyond Pesticides Makes Science-based Case that It Is Imperative to�Phase Out Pesticides in a Decade
- On Earth Day, Coalition Calls on NYC Parks Department to Set Up Demonstration Sites
- The 2022 National Forum Series Launches Beyond Pesticides� Campaign to Eliminate Fossil Fuel-Based Pesticide Use Within the Next Decade
- Statement: On War in Ukraine
- Statement: Beyond Pesticides Stands with Black Lives Matter

- Hydroponics (13)
- air pollution (8)
- National Organic Standards Board/National Organic Program (105)
- Organic Foods Production Act OFPA (8)
- Parks for a Sustainable Future (6)
- Regenerative (21)
- Announcements (601)
- nanosilver (4)
- Antibiotic Resistance (41)
- Antimicrobial (18)
- Aquaculture (30)
- Aquatic Organisms (35)
- Beneficials (51)
- mayflies (2)
- Soil microbiome (8)
- Biofuels (6)
- Biological Control (34)
- Biomonitoring (40)
- hydroxyatrazine (1)
- btomsfiolone (1)
- Bug Bombs (2)
- Canada (10)
- Cannabis (29)
- Centers for Disease Control and Prevention (CDC) (9)
- Chemical Mixtures (5)
- 1, 3-dichloropropene (4)
- 2,4-D (136)
- 4-aminopyridine (2)
- Abamectin (5)
- Acephate (10)
- acetamiprid (20)
- Acetochlor (3)
- Agent Orange (4)
- Alachlor (7)
- Aldicarb (18)
- aluminum phosphide (4)
- Aminocyclopyrachlor (8)
- aminomethylphosphonic acid (AMPA) (1)
- aminopyralid (3)
- amitraz (2)
- Antibiotic (9)
- arsenic (4)
- Atrazine (122)
- azamethiphos (2)
- Azinphos-methyl (8)
- Azoxystrobin (8)
- bacillus thuringiensis (2)
- Bendiocarb (2)
- Benomyl (4)
- Bensulide (2)
- Bentazon (1)
- Bifenthrin (26)
- boscalid (1)
- Brodificoum (7)
- Bromacil (2)
- bromoxynil (1)
- cacodylic acid (1)
- carbamate (1)
- Carbamates (7)
- Carbaryl (34)
- Carbendazim (3)
- Carbofuran (18)
- Chlordane (20)
- Chlordecone (1)
- chlormequat (1)
- chloropicrin (15)
- Chlorothalonil (22)
- Chlorpyrifos (198)
- clopyralid (9)
- Clothianidin (117)
- Compound 1080 (2)
- copper sulfate (4)
- Coumaphos (8)
- creosote (8)
- Cyanazine (1)
- Cyanide (2)
- Cyantraniliprole (4)
- Cyfluthrin (13)
- cypermethrin (19)
- Daconil (1)
- dacthal (3)
- Dazomet (3)
- Deltamethrin (19)
- Diazinon (49)
- Dicamba (71)
- dichlorophenol (1)
- Dichlorvos (4)
- dicofol (3)
- Dieldrin (18)
- difenoconazole (2)
- Diflubenzuron (2)
- Dimethoate (11)
- dinotefuron (34)
- dipropylene glycol (3)
- Diquat dibromide (1)
- Dithiocarbamates (1)
- Dursban (1)
- emamectin (2)
- Endosulfan (43)
- endothall (1)
- esfenvalerate (7)
- essential oils (1)
- Ethoprop (4)
- ethoxylates (1)
- fenbuconazole (2)
- Fenoxycarb (3)
- Fenthion (2)
- Fipronil (29)
- fludioxonil (5)
- Flumethrin (5)
- fluoride (2)
- flupyradifurone (9)
- fluridone (1)
- fluvalinate (6)
- fluxapyroxad (1)
- Fumigants (8)
- glufosinate (11)
- Glyphosate (387)
- Heptachlor (5)
- hexachlorobenzene (HCB) (10)
- hexazinone (1)
- hydramethylnon (1)
- Idomethane (1)
- Imazapyr (6)
- Imidacloprid (147)
- indaziflam (2)
- indoxacarb (1)
- phthalates (4)
- α-(p-nonylphenyl)-ω-hydroxypoly(oxyethylene) (APNOHO) (2)
- iron phosphate (1)
- Isoxafutole (1)
- Ivermectin (1)
- lead arsenate (2)
- Ledprona (1)
- Lindane (28)
- Linuron (4)
- magnesium phosphide (2)
- Malathion (62)
- mancozeb (1)
- Metalaxyl (3)
- metaldehyde (1)
- Metam Potassium (2)
- Metam Sodium (9)
- Methamidophos (6)
- Methidathion (2)
- methionine (1)
- Methomyl (21)
- Methoprene (8)
- Methoxyfenozide (2)
- methyl bromide (38)
- methyl iodide (24)
- methyl isocyanate (MIC) (2)
- methyl mercaptan (1)
- Metolachlor (12)
- Mevinphos (1)
- Myclobutanil (8)
- Nanosilver (1)
- Napthalene (2)
- neonicotinoids (316)
- Nitrates (3)
- Nitrites (2)
- o-phenylphenol (1)
- organoarsenic (1)
- organochlorines (31)
- organophosphate (91)
- Oryzalin (1)
- oxyfluorfen (1)
- paldoxins (1)
- Para-dichlorobenzene (PDCB) (1)
- Paraquat (44)
- Parathion (4)
- Pendimethalin (5)
- Pentachlorophenol (20)
- Permethrin (55)
- Phenothrin (2)
- Phorate (4)
- Phosmet (2)
- Phosphine (3)
- Piperonyl butoxide (PBO) (9)
- polyethoxylated tallowamine (2)
- Prometon (2)
- Propargite (6)
- Propazine (3)
- Propetamphos (1)
- Propiconazole (5)
- Propoxur (11)
- propyzamide (1)
- pyraclostrobin (1)
- Pyrethrin (27)
- pyrethroids (14)
- pyrimethanil (2)
- Pyriproxyfen (1)
- Rotenone (8)
- simazine (11)
- Sodium hypochlorite (1)
- spinosad (2)
- spiromesifen (1)
- streptomycin (2)
- strychnine (1)
- Sulfoxaflor (28)
- sulfuryl fluoride (19)
- surfactants (1)
- Synthetic Pyrethroid (37)
- Taurine (1)
- tebuconazole (6)
- Tebuthiuron (3)
- terbufos (2)
- Tetrachlorvinphos (TCVP) (2)
- tetraconazole (1)
- thiabendazole (1)
- thiacloprid (16)
- Thiamethoxam (95)
- thiodicarb (3)
- toxaphene (1)
- Triazines (2)
- Tributyltin (3)
- Trichlorfon (5)
- Triclopyr (15)
- Triclosan (100)
- Trifluralin (6)
- Warfarin (2)
- Zinc Phosphide (1)
- Children (112)
- Children/Schools (240)
- cicadas (1)
- Climate (30)
- Climate Change (85)
- compost (5)
- Congress (17)
- contamination (154)
- Ag-Mart (4)
- Allied BioScience (1)
- Arysta LifeScience Corporation (4)
- Aventis CropScience (1)
- Bayer (155)
- Cargill (2)
- Chem-China (2)
- Corteva (8)
- Dow Chemical (116)
- Driscoll’s (1)
- DuPont (40)
- Forage Genetics (1)
- General Mills (6)
- GreenLight Biosciences (1)
- Hartz Mountain (1)
- Home Depot (20)
- Inhance Technologies (2)
- McDonald’s (2)
- Monsanto (241)
- Montrose (4)
- Natural Grocers (1)
- Novartis (1)
- Organic Trade Association OTA (1)
- PG&E (2)
- Promiseland (1)
- Reckitt Benckiser (6)
- Scotts MiracleGro (5)
- Sel-Cor Bean and Pea Inc (1)
- Seresto (3)
- Syngenta (84)
- TruGreen (7)
- Uncle Matt’s Organic (1)
- Velsicol (1)
- Wal-Mart (11)
- Whole Foods (5)
- deethylatrazine (1)
- Allergy (1)
- Alzheimers’s (15)
- Amyotrophic Lateral Sclerosis (ALS) (5)
- Arthritis/Joint Inflammation (1)
- Asthma (23)
- Autism (30)
- Avian Flu (1)
- behavioral and cognitive effects (9)
- Birth defects (69)
- Blood Disorders (3)
- Body Burden (6)
- Brain Effects (44)
- Breast Cancer (30)
- Cancer (185)
- Cardiovascular Disease (20)
- Celiac Disease (1)
- chemical sensitivity (4)
- Chronic Kidney Disease (7)
- coronavirus (17)
- COVID-19 (10)
- cryptorchidism (2)
- Depression (8)
- Dermatitis (2)
- Developmental Disorders (39)
- Diabetes (33)
- DNA Damage (1)
- Ear Infection (1)
- Metabolic Disorders (4)
- Endometrial (Uterine) Cancer (1)
- endometriosis (1)
- Epigenetic (10)
- Food Borne Illness (1)
- Gulf War Syndrome (7)
- Gut Dysbiosis (6)
- Hearing Loss (2)
- Hematological Malignancies (1)
- Hypersensitivity (2)
- Immunotoxicity (5)
- Infertility (18)
- Inflammatory bowel disease (IBD) (4)
- IQ Loss (2)
- Kidney failure (12)
- Learning Disabilities (24)
- Leukemia (16)
- Lewy Body Disease (LBD) (2)
- Liver Damage (17)
- Liver failure (12)
- Lung Cancer (4)
- Lymphoma (6)
- Malaria (10)
- MCS/chem. sensitivity (3)
- metabolic syndrome (7)
- Microcephaly (1)
- Miscarriage (5)
- Motor Development Effects (3)
- Motor neuron disease (5)
- multi-generational effects (15)
- Multiple Myeloma (4)
- Multiple Sclerosis (MS) (1)
- Nervous System Effects (79)
- non-Hodgkin’s Lymphoma (48)
- Obesity (24)
- olfactory effects (2)
- Oxidative Stress (14)
- Parkinson’s (52)
- Prostate Cancer (10)
- Reproductive Health (63)
- Respiratory Diseases (11)
- Respiratory Problems (24)
- Rheumatoid arthritis (1)
- schistosomiasis (1)
- Seizures/Epilepsy (1)
- Skin Reactions (4)
- Sleep Disorders (1)
- Suicide (5)
- Thyroid Disease (16)
- Disinfectants & Sanitizers (18)
- Drinking Water (15)
- Ecosystem Services (12)
- Emergency Exemption (3)
- Environmental Justice (166)
- Environmental Protection Agency (EPA) (517)
- Events (88)
- Farm Bill (21)
- Farmworkers (195)
- Centers for Disease Control and Prevention (11)
- National Environmental Policy Act (2)
- Department of Defense (2)
- Department of Interior (16)
- Department of Justice (2)
- Fish and Wildlife Service (FWS) (58)
- Food and Drug Administration (FDA) (15)
- Health Canada (3)
- National Institute for Environmental Health Sciences (3)
- NOSB National Organic Standards Board (3)
- Occupational Safety and Health Administration (1)
- U.S. Agency for International Development (USAID) (1)
- U.S. Geological Survey (12)
- U.S. Trade Representative (1)
- US Department of Agriculture (USDA) (133)
- Veterans Administraton (3)
- Biosolids (7)
- Sewage Sludge (6)
- Synthetic Fertilizer (4)
- farmed fish (1)
- Forestry (5)
- Fracking (4)
- Fungal Resistance (6)
- Fungicides (25)
- Contamination (81)
- Labeling (61)
- Mutagenesis (3)
- Greenhouse (1)
- Groundwater (14)
- Health care (32)
- Herbicides (38)
- Holidays (37)
- Household Use (9)
- Indigenous People (6)
- Indoor Air Quality (6)
- Infectious Disease (4)
- Integrated and Organic Pest Management (71)
- United Nations (15)
- Invasive Species (35)
- Label Claims (49)
- Lawns/Landscapes (248)
- Litigation (342)
- Livestock (9)
- men’s health (1)
- metabolic syndrome (3)
- Metabolites (4)
- Microbiata (22)
- Microbiome (28)
- molluscicide (1)
- Nanosilver (2)
- Nanotechnology (54)
- National Politics (388)
- Native Americans (3)
- Occupational Health (15)
- Oceans (10)
- Office of Inspector General (3)
- perennial crops (1)
- Pesticide Drift (162)
- Pesticide Efficacy (10)
- Pesticide Mixtures (9)
- Pesticide Regulation (776)
- Pesticide Residues (183)
- Bedbugs (19)
- brown marmorated stink bug (2)
- citrus greening–Huanglongbing (HLB) (3)
- Cockroaches (7)
- coffee berry borer (CBB) (1)
- Colorado Potato Beetle (1)
- corn rootworm (4)
- Feral Hogs (1)
- Fire Ants (1)
- Grasshoppers (2)
- Gypsy Moth (2)
- Head lice (10)
- Insect-Borne Disease (16)
- Japanese Beetle (1)
- Mosquitoes (77)
- Nematodes (1)
- Pigeons (1)
- Powdery Mildew (1)
- Rodents (17)
- spider mites (1)
- termites (6)
- Plant Incorporated Protectants (1)
- Plastic (7)
- Poisoning (20)
- Habitat Protection (66)
- Increased Vulnerability to Diseases from Chemical Exposure (98)
- Persistence (46)
- Preemption (43)
- President-elect Transition (2)
- Repellent (4)
- Resistance (118)
- Rights-of-Way (1)
- Rodenticide (33)
- Seasonal (3)
- soil health (17)
- Alabama (3)
- Alaska (13)
- Arizona (7)
- Arkansas (17)
- California (266)
- Colorado (45)
- Connecticut (33)
- Deleware (3)
- Florida (52)
- Georgia (9)
- Hawaii (50)
- Illinois (26)
- Indiana (7)
- Kentucky (3)
- Louisiana (5)
- Maryland (50)
- Massachusetts (23)
- Michigan (22)
- Minnesota (48)
- Mississippi (3)
- Missouri (17)
- Montana (5)
- Nebraska (7)
- Nevada (11)
- New Hampshire (3)
- New Jersey (27)
- New Mexico (12)
- New York (70)
- North Carolina (18)
- North Dakota (13)
- Oklahoma (5)
- Oregon (94)
- Pennsylvania (11)
- Puerto Rico (1)
- Rhode Island (5)
- South Carolina (6)
- South Dakota (7)
- Tennessee (5)
- Vermont (17)
- Virginia (10)
- Washington (59)
- Washington D.C. (35)
- West Virginia (8)
- Wisconsin (9)
- Wyoming (4)
- Superfund (4)
- synergistic effects (19)
- Synthetic Pyrethroids (16)
- Synthetic Turf (3)
- Take Action (588)
- Textile/Apparel/Fashion Industry (1)
- Toxic Waste (11)
- Biosolids/Sewage Sludge (2)
- Volatile Organic Compounds (1)
- Water Regulation (50)
- Endangered Species Act (ESA) (2)
- Women’s Health (25)
- Wood Preservatives (36)
- World Health Organization (10)
- Year in Review (2)
Most Viewed Posts
- On Earth Day, Especially, Take Action to Ensure a Sustainable Future (326,095)
- Research Links Parkinson’s and Lewy Body Disease with Chemical Effects on Brain and Gut (317,790)
- Lawsuit Challenges TruGreen Chemical Lawn Care Company for Deceptive Safety Claims; Pesticide Applications Stopped by Some States During COVID-19 Crisis as Nonessential (124,735)
- Glyphosate Causes Changes to DNA Function Resulting in Chronic Disease, According to Study (62,725)
- Over Two Million Bees Killed after Aerial Mosquito Spraying in South Carolina (58,102)
- Glyphosate Classified Carcinogenic by International Cancer Agency, Group Calls on U.S. to End Herbicide’s Use and Advance Alternatives (55,766)
- Community Passes Resolution Banning Neonicotinoids (53,288)
- Cosmetic Lawn Pesticide Use Outlawed In Takoma Park, MD, First Local Ban Of Its Type in U.S. (49,896)
- Roundup Shown to Kill Bees—But Not How You Might Expect (49,690)
- EPA Permits Experimental Release of 2.5 Billion Genetically Engineered Mosquitoes in California and Florida (46,405)
Using the Blog
- About the Daily News Blog
- How to Comment
- Recent Posts
Recent Comments
- P. Bailey on U.S. Residents Urged Not to Spray Pesticides for Periodical Cicadas
- Torre on Multiple Chemical Sensitivity Recognized by State of Massachusetts
- Steve on Pesticide Use Again Linked to Inflammatory Bowel Disease, This Time Among Applicators and Their Spouses
Admin / Feeds
- Entries feed
- Comments feed
- WordPress.org
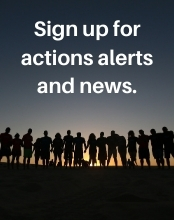
Government Report Pushes Genetically Engineered Crops, Despite Failure and Effective Alternatives
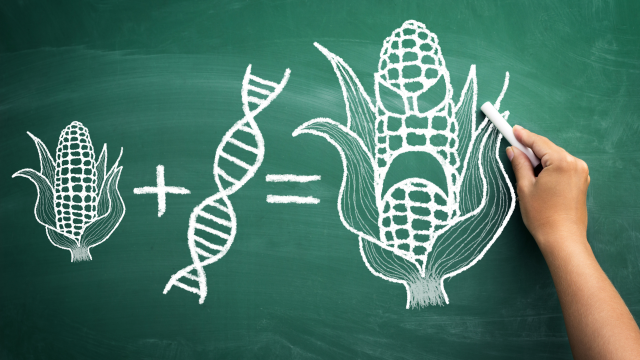
( Beyond Pesticides , May 31, 2024) Among the many promises that have been made by agribusiness to farmers and consumers, the glories of crops genetically engineered (GE) to resist pests stand out. GE tools—genes—were touted as “natural,” and promised to reduce the use of toxic pesticides. The first such plants incorporating DNA or RNA from other organisms hit the market in the 1990s. Today more than 70% of all GE organisms are engineered to tolerate herbicides, and the overwhelming majority of corn, soybean and cotton varieties are engineered to to be toxic to insects. See Beyond Pesticides’ backgrounder on GE here .
Despite a dramatic increase in the use of herbicides and the fast development of weed and insect resistance to plant incorporated pesticides, this month the U.S. Department of Agriculture (USDA), the Food and Drug Administration (FDA) and the U.S. Environmental Protection Agency (EPA) jointly released a document entitled “The Coordinated Framework for the Regulation of Biotechnology – Plan for Regulatory Reform under the Coordinated Framework for the Regulation of Biotechnology.” It responds to a 2022 executive order by President Biden to “accelerate biotechnology innovation” and “support the safe use of biotechnology products” by using a “science- and risk-based, predictable, efficient, and transparent regulatory system.”
The most common GE insecticide is the class of Bt toxins derived from the soil bacterium Bacillus thuringiensis , which are commonly used on the various moth species whose larvae plague corn, soybeans and cotton. Genes for the toxins are inserted into the crop genomes so that each part of the plant will express them. But nature evolves inexorably: USDA knew at least by 1998 that resistance to Bt toxins was going to be a problem, and within five years growers reported that the first Bt toxin was becoming ineffective against target insects. Currently, resistance has been documented to at least three Bt toxins.
Although many voices objected to using genes as pesticides in agriculture when the idea was first proposed, technologies for doing so have proliferated while regulation has been criticized as being slipshod at best. It has also been duplicative, inefficient and confusing, causing the chemical industry—principally Monsanto (now Bayer), Dow Chemical, and Syngenta to complain about red tape while also feeling pressure to invent new GE insecticidal technologies faster than they become obsolete.
Much of the multi-agency document demonstrates that it is aimed at reducing obstacles to industry’s further development, marketing and dissemination of GE products. For example, interstate transport of some GE plants will no longer need permits. EPA has recently implemented a final rule regarding “plant-incorporated protectants” (PIPs) that exempts some PIPS from regulation under the Federal Insecticide, Fungicide and Rodenticide Act (FIFRA). The FDA will assist developers of genome-edited plants to “voluntarily interact with FDA prior to marketing foods from their new varieties.”
In a rare acknowledgment of actual hazards and risks, the document also states that FDA and USDA “intend to collaborate” to create “stewardship” of food crops that may be unsafe, such as those with genes inserted from other species that may be allergenic. The document also addresses the use of modified microorganisms, again devoting much of its consideration to making it easier for commercial interests to develop and market their creations rather than protecting public health or sustainable agriculture.
While GE technologies have reduced the use of field-applied pesticides somewhat, the Darwinian development of pest resistance remains a glaring problem. After resistance to Bt toxins appeared, biotech companies started “stacking” genes for more toxins into their seeds. This merely delayed the inevitable. Currently the widely-used “pyramid” of three toxins is reaching the end of its useful life.
Because resistance emerged almost immediately during initial product development, biotech interests and regulators began telling farmers to include refugia on the edges of fields, and eventually within fields. Refugia would be planted on the edges of non-Bt crops, with the idea that the resistant and non-resistant bugs would interbreed, thus maintaining genetic susceptibility to Bt in the overall population.
This has not worked well. For example, adult females of the western corn rootworm (Diabrotica virgifera virgifera) don’t travel outside the refugia , so they do not mate with the resistant males from the main fields. This seems like something that could have been checked in a field trial before promising farmers refugia would solve their problems, but apparently this did not happen. An additional problem is that refuge strategies are not always practicable. According to the Malaysian NGO Third World Network , “Over-enthusiastic modelling of efficacy under ideal conditions has been one factor that has promoted the reduction in refuge requirements in the US, but the claimed efficacy has not been reflected in real-world conditions.”
In 2021 an Iowa State University entomologist described a further problem emerging from the use of Bt products against the western corn rootworm: Monoculture—vast areas planted with one crop—creates “the ideal habitat for this pest, and can be associated with large populations…and high levels of larval feeding injury.” Thus not only monoculture per se, but monoculture over time exacerbates the convoluted contradictions of industrial agriculture.
Industrial geneticists may believe they understand the genetic and environmental factors that determine genetic modifications’ effectiveness and the development of resistance by pests, but they have to-date failed. Research by Chinese scientists reported in 2021 provides a striking example. Helicoverpa armigera , a cotton bollworm, is a rapidly spreading pest throughout Europe, Africa and Asia. The Chinese scientists found that the bollworms infected by a densovirus known to be beneficial to the insect had higher resistance to Bt crops, and that areas in China planted with Bt crops had a higher rate of viral infection. The researchers suggest that the mutualistic relationship between the virus and the insect led to enhanced insect survival after Bt exposure. The mutualism reduces the fitness cost to the bollworm for developing resistance as the bollworm outcompetes the toxin. The potential for microbial involvement in Bt resistance had not occurred to the industry.
This study demonstrates the ultimate folly of corporate biotechnological thinking. Changing a few genes in one organism may have far-reaching ripple effects that are likely unpredictable. The ways in which various species, such as insects and their microbial companions, negotiate the spectrum between pathogenicity, mutualism, and symbiosis is poorly understood, and these relationships interact with environmental conditions. Pest resistance to GE technologies may be only one of a cascade of unintended consequences. For example, there is evidence that GE pest resistance genes affect product quality, as illustrated by Burkina Faso’s renowned cotton industry suffering severe losses after adopting Bt seeds, which produced inferior cotton.
Yet the agricultural biotech industry is busily trying to develop new generations of the same idea, including higher pyramids of stacked insecticidal traits. SmartStax PRO, a new GE corn product devised jointly by Bayer and Dow Chemical, is just being introduced into U.S agriculture. It combines six different toxin genes, five derived from Bt (one of which does not exist in nature, according to a backgrounder from the Brussels-based Institute for Independent Impact Assessment of Biotechnology (Test BioTech)). It also contains an RNA interference component that lethally silences an essential gene in the rootworm. Bayer markets Intacta 2 Xtend for soybeans, which contains a stack of three toxin genes along with tolerance to glyphosate, glufosinate and dicamba. These novel combinations of toxins and pesticide tolerance genes may produce sharply higher toxicity and affect far more species than the targets. It is unclear whether the manufacturers have investigated the broader effects of combining all these mechanisms, but likely have, as usual, looked at each one in isolation.
A 2022 Test BioTech report stresses that new gene editing techniques pose a profound risk to the planetary ecosystem because “an increasing number of projects [are] looking at wild populations and a broad range of organisms such as microorganisms, insects, rodents and trees…similarly to environmental pollution with plastics and chemicals, it is not always an individual NGT-GMO which may create the real problems, but rather the sum of diverse effects on the environment.”
What seems obvious is that either or both of two outcomes are likely: pests, which are simply availing themselves of a bountiful, convenient food source—will again, as ever, develop resistance to humans’ technological wonders; or the unintended consequences of each technology and their combinations will produce the very food crises the industry claims to be preventing. As the Biden administration’s biotechnology modernization document shows, industry is proceeding apace with the help of government policies. We wonder whether these interests are so grotesquely deluded as to their power over nature, or simply cynically looking for products “good enough” to delay the inevitable through a few more rounds of profit-taking at the expense of farmers, consumers, and the natural world.
See Beyond Pesticides organic agriculture page and efforts to continually build organic integrity and the organic sector as what appears to be the only alternative to the current existential crises challenged by pesticide-induced diseases, biodiversity collapse, and the climate emergency.
All unattributed positions and opinions in this piece are those of Beyond Pesticides.
What You Can Do:
- Tell the U.S. Trade Representative and the U.S. Secretary of State to withdraw opposition to Mexico’s ban on imported GE corn . In 2023, Mexico banned genetically modified corn, including Bt corn, and ordered the phaseout of glyphosate, a move that the U.S. has challenged under the Canada-US-Mexico Trade Agreement , which replaced the North American Free Trade Act in 2018. See Beyond Pesticides’ coverage of this issue for more detail.
- Tell USDA Secretary Tom Vilsack and members of Congress to require USDA agencies to honestly disclose genetically engineered ingredients and carry out its modernization goals.
- Urge your U.S. Senators and Representative to ask Agriculture Committees to hold oversight hearings to ensure that USDA, FDA and EPA hold to those goals.
The Coordinated Framework for the Regulation of Biotechnology – Plan for Regulatory Reform under the Coordinated Framework for the Regulation of Biotechnology U.S. Department of Agriculture, Environmental Protection Agency, and Food and Drug Administration May 2024 https://usbiotechnologyregulation.mrp.usda.gov/eo14081-section8c-plan-reg-reform.pdf
Rapid spread of a densovirus in a major crop pest following wide-scale adoption of Bt-cotton in China Xiao et al. eLife 2021 https://elifesciences.org/articles/66913
Canada & US vs. Mexico’s Ban on GM Corn The CUSMA trade dispute champions the biotech industry over food sovereignty Canadian Biotechnology Action Network Brief January 29, 2024 https://cban.ca/wp-content/uploads/CBAN-Brief-Canada-vs-Mexico-Jan-2024.pdf
Bt Crops Past Their Sell-By Date: A Failing Technology Searching for New Markets? Third World Network Bhd (198701004592 (163262-P) 2022 www.twn.my
https://wp.twnnews.net/wp-content/uploads/2022/09/BiotechnBiosafe19complete.pdf

This entry was posted on Friday, May 31st, 2024 at 12:01 am and is filed under Agriculture , Bayer , Dow Chemical , Environmental Protection Agency (EPA) , Food and Drug Administration (FDA) , Genetic Engineering , Syngenta , Uncategorized , US Department of Agriculture (USDA) . You can follow any responses to this entry through the RSS 2.0 feed. You can skip to the end and leave a response. Pinging is currently not allowed.
Leave a Reply
Name (required)
Mail (will not be published) (required)
XHTML: You can use these tags: <a href="" title=""> <abbr title=""> <acronym title=""> <b> <blockquote cite=""> <cite> <code> <del datetime=""> <em> <i> <q cite=""> <s> <strike> <strong>
Contact | News & Press | Site Map | ManageSafe™ | Tools for Change | File a Pesticide Incident Report | Pesticide Gateway | Privacy Policy | Submit News, Studies & Stories
- Agriculture ›
Genetically modified crops - statistics & facts
Acreage of gm crops, gm crops in the u.s., gm crops in canada, consumer attitudes and understanding, key insights.
Detailed statistics
Acreage of genetically modified crops worldwide 2003-2019
Acreage of genetically modified crops by species 2003-2019
Acreage of genetically modified crops 2015-2019, by country
Editor’s Picks Current statistics on this topic
Crop Production
Global genetically modified crops by countries 2019, based on acreage
Global adoption rate for major biotech crops worldwide 2019, by type
Biotech and non-biotech corn areas in the U.S. 2022
Further recommended statistics
Global overview.
- Premium Statistic Acreage of genetically modified crops worldwide 2003-2019
- Premium Statistic Acreage of genetically modified crops 2015-2019, by country
- Premium Statistic Global genetically modified crops by countries 2019, based on acreage
- Premium Statistic Acreage of genetically modified crops by species 2003-2019
- Basic Statistic Global adoption rate for major biotech crops worldwide 2019, by type
Acreage of genetically modified crops worldwide from 2003 to 2019 (in million hectares)
Acreage of genetically modified crops worldwide from 2015 to 2019, by leading country (in million hectares)*
Area of genetically modified (GM) crops worldwide in 2019, by country (in million hectares)
Acreage of genetically modified crops from 2003 to 2019, by species (in million hectares)
Adoption of GM technology among selected major crops worldwide in 2019, by type*
- Premium Statistic Acreage of major genetically engineered crops in the U.S. by type 2019
- Premium Statistic Percentage of genetically modified crops in the U.S. by type 1997, 2018, 2019 & 2020
- Premium Statistic U.S. acreage of genetically modified corn 2014-2019
- Premium Statistic Biotech and non-biotech corn areas in the U.S. 2022
- Basic Statistic Distribution of U.S. biotech corn acres 2006-2022
- Premium Statistic U.S. acreage of genetically modified soybeans 2014-2019
- Premium Statistic U.S. acreage of genetically modified cotton 2014-2019
Acreage of major genetically engineered crops in the U.S. by type 2019
Major genetically modified (GM) crops in the United States in 2019, by crop type (in million hectares)*
Percentage of genetically modified crops in the U.S. by type 1997, 2018, 2019 & 2020
Percentage of genetically modified crops in the U.S. in 1997, 2018, 2019, and 2020, by type (as percent of total acreage)
U.S. acreage of genetically modified corn 2014-2019
Acreage of genetically modified (GM) corn in the United States from 2014 to 2019 (in million hectares)
Biotech and non-biotech corn areas in the U.S. in 2022 (in 1,000 acres)*
Distribution of U.S. biotech corn acres 2006-2022
Distribution of U.S. biotech and non-biotech corn acreage from 2006 to 2022, by type
U.S. acreage of genetically modified soybeans 2014-2019
Acreage of genetically modified (GM) soybeans in the United States from 2014 to 2019 (in million hectares)
U.S. acreage of genetically modified cotton 2014-2019
Acreage of genetically modified (GM) cotton in the United States from 2014 to 2019 (in million acres hectares)
- Premium Statistic Seeded area of genetically engineered crops in Canada 2012-2023
- Premium Statistic Seeded area of genetically engineered canola in Canada 2012-2023
- Premium Statistic Genetically engineered canola share of seeded canola area in Canada 2012-2023
- Premium Statistic Canada's seeded area of genetically engineered soybeans 2012-2023
- Premium Statistic Genetically engineered soybeans share of seeded soybeans area in Canada 2012-2023
- Premium Statistic Seeded area of genetically engineered corn in Canada 2011-2023
- Premium Statistic Genetically engineered corn share of seeded corn area in Canada 2012-2023
- Premium Statistic Seeded area of genetically modified soybeans in Ontario 2011-2023
- Premium Statistic Seeded area of genetically modified soybeans in Quebec 2011-2023
- Premium Statistic Seeded area of genetically modified corn for grains in Ontario 2011-2023
- Premium Statistic Seeded area of genetically modified corn for grains in Quebec 2011-2023
Seeded area of genetically engineered crops in Canada 2012-2023
Seeded area of genetically engineered crops in Canada from 2012 to 2023 (in million hectares)
Seeded area of genetically engineered canola in Canada 2012-2023
Seeded area of genetically engineered canola in Canada from 2012 to 2023 (in million hectares)
Genetically engineered canola share of seeded canola area in Canada 2012-2023
Genetically engineered canola share of seeded canola area in Canada from 2012 to 2023
Canada's seeded area of genetically engineered soybeans 2012-2023
Seeded area of genetically engineered soybeans in Canada from 2012 to 2023 (in million hectares)
Genetically engineered soybeans share of seeded soybeans area in Canada 2012-2023
Genetically engineered soybeans share of seeded soybeans area in Canada from 2012 to 2023
Seeded area of genetically engineered corn in Canada 2011-2023
Seeded area of genetically engineered corn in Canada from 2011 to 2023 (in million hectares)
Genetically engineered corn share of seeded corn area in Canada 2012-2023
Genetically engineered corn share of seeded corn area in Canada from 2012 to 2023
Seeded area of genetically modified soybeans in Ontario 2011-2023
Seeded area of genetically modified soybeans in Ontario from 2011 to 2023 (in 1,000 hectares)
Seeded area of genetically modified soybeans in Quebec 2011-2023
Seeded area of genetically modified soybeans in Quebec from 2011 to 2023 (in 1,000 hectares)
Seeded area of genetically modified corn for grains in Ontario 2011-2023
Seeded area of genetically modified corn for grains in Ontario from 2011 to 2023 (in 1,000 hectares)
Seeded area of genetically modified corn for grains in Quebec 2011-2023
Seeded area of genetically modified corn for grains in Quebec from 2011 to 2023 (in 1,000 hectares)
Mon - Fri, 9am - 6pm (EST)
Mon - Fri, 9am - 5pm (SGT)
Mon - Fri, 10:00am - 6:00pm (JST)
Mon - Fri, 9:30am - 5pm (GMT)
September 1, 2013
13 min read
The Truth about Genetically Modified Food
Proponents of genetically modified crops say the technology is the only way to feed a warming, increasingly populous world. Critics say we tamper with nature at our peril. Who is right?
By David H. Freedman
Robert Goldberg sags into his desk chair and gestures at the air. “Frankenstein monsters, things crawling out of the lab,” he says. “This the most depressing thing I've ever dealt with.”
Goldberg, a plant molecular biologist at the University of California, Los Angeles, is not battling psychosis. He is expressing despair at the relentless need to confront what he sees as bogus fears over the health risks of genetically modified (GM) crops. Particularly frustrating to him, he says, is that this debate should have ended decades ago, when researchers produced a stream of exonerating evidence: “Today we're facing the same objections we faced 40 years ago.”
Across campus, David Williams, a cellular biologist who specializes in vision, has the opposite complaint. “A lot of naive science has been involved in pushing this technology,” he says. “Thirty years ago we didn't know that when you throw any gene into a different genome, the genome reacts to it. But now anyone in this field knows the genome is not a static environment. Inserted genes can be transformed by several different means, and it can happen generations later.” The result, he insists, could very well be potentially toxic plants slipping through testing.
On supporting science journalism
If you're enjoying this article, consider supporting our award-winning journalism by subscribing . By purchasing a subscription you are helping to ensure the future of impactful stories about the discoveries and ideas shaping our world today.
Williams concedes that he is among a tiny minority of biologists raising sharp questions about the safety of GM crops. But he says this is only because the field of plant molecular biology is protecting its interests. Funding, much of it from the companies that sell GM seeds, heavily favors researchers who are exploring ways to further the use of genetic modification in agriculture. He says that biologists who point out health or other risks associated with GM crops—who merely report or defend experimental findings that imply there may be risks—find themselves the focus of vicious attacks on their credibility, which leads scientists who see problems with GM foods to keep quiet.
Whether Williams is right or wrong, one thing is undeniable: despite overwhelming evidence that GM crops are safe to eat, the debate over their use continues to rage, and in some parts of the world, it is growing ever louder. Skeptics would argue that this contentiousness is a good thing—that we cannot be too cautious when tinkering with the genetic basis of the world's food supply. To researchers such as Goldberg, however, the persistence of fears about GM foods is nothing short of exasperating. “In spite of hundreds of millions of genetic experiments involving every type of organism on earth,” he says, “and people eating billions of meals without a problem, we've gone back to being ignorant.”
So who is right: advocates of GM or critics? When we look carefully at the evidence for both sides and weigh the risks and benefits, we find a surprisingly clear path out of this dilemma.
Benefits and worries
The bulk of the science on GM safety points in one direction. Take it from David Zilberman, a U.C. Berkeley agricultural and environmental economist and one of the few researchers considered credible by both agricultural chemical companies and their critics. He argues that the benefits of GM crops greatly outweigh the health risks, which so far remain theoretical. The use of GM crops “has lowered the price of food,” Zilberman says. “It has increased farmer safety by allowing them to use less pesticide. It has raised the output of corn, cotton and soy by 20 to 30 percent, allowing some people to survive who would not have without it. If it were more widely adopted around the world, the price [of food] would go lower, and fewer people would die of hunger.”
In the future, Zilberman says, those advantages will become all the more significant. The United Nations Food and Agriculture Organization estimates that the world will have to grow 70 percent more food by 2050 just to keep up with population growth. Climate change will make much of the world's arable land more difficult to farm. GM crops, Zilberman says, could produce higher yields, grow in dry and salty land, withstand high and low temperatures, and tolerate insects, disease and herbicides.
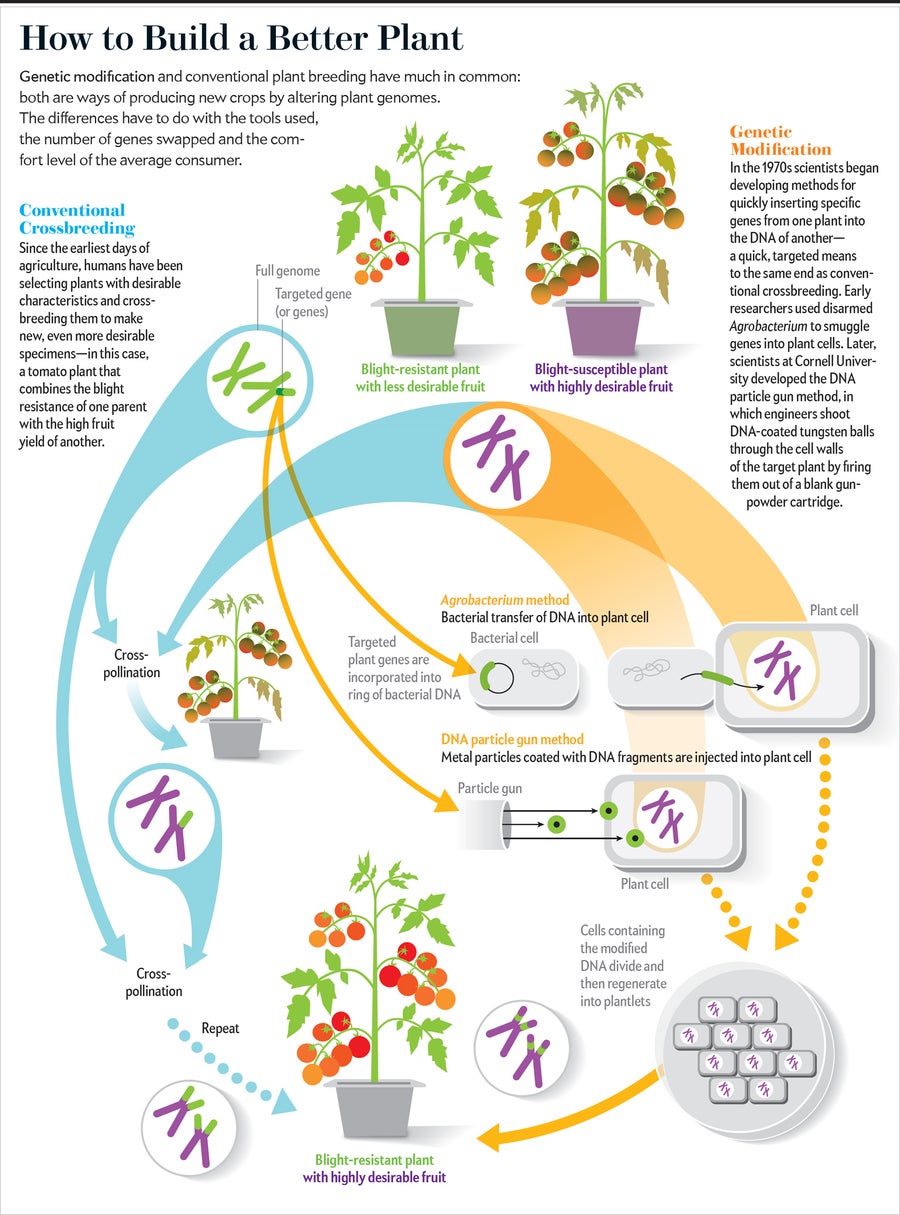
Credit: Jen Christiansen
Despite such promise, much of the world has been busy banning, restricting and otherwise shunning GM foods. Nearly all the corn and soybeans grown in the U.S. are genetically modified, but only two GM crops, Monsanto's MON810 maize and BASF's Amflora potato, are accepted in the European Union. Ten E.U. nations have banned MON810, and although BASF withdrew Amflora from the market in 2012, four E.U. nations have taken the trouble to ban that, too. Approval of a few new GM corn strains has been proposed there, but so far it has been repeatedly and soundly voted down. Throughout Asia, including in India and China, governments have yet to approve most GM crops, including an insect-resistant rice that produces higher yields with less pesticide. In Africa, where millions go hungry, several nations have refused to import GM foods in spite of their lower costs (the result of higher yields and a reduced need for water and pesticides). Kenya has banned them altogether amid widespread malnutrition. No country has definite plans to grow Golden Rice, a crop engineered to deliver more vitamin A than spinach (rice normally has no vitamin A), even though vitamin A deficiency causes more than one million deaths annually and half a million cases of irreversible blindness in the developing world.
Globally, only a tenth of the world's cropland includes GM plants. Four countries—the U.S., Canada, Brazil and Argentina—grow 90 percent of the planet's GM crops. Other Latin American countries are pushing away from the plants. And even in the U.S., voices decrying genetically modified foods are becoming louder. In 2016 the U.S. federal government passed a law requiring labeling of GM ingredients in food products, replacing GM-labeling laws in force or proposed in several dozen states.
The fear fueling all this activity has a long history. The public has been worried about the safety of GM foods since scientists at the University of Washington developed the first genetically modified tobacco plants in the 1970s. In the mid-1990s, when the first GM crops reached the market, Greenpeace, the Sierra Club, Ralph Nader, Prince Charles and a number of celebrity chefs took highly visible stands against them. Consumers in Europe became particularly alarmed: a survey conducted in 1997, for example, found that 69 percent of the Austrian public saw serious risks in GM foods, compared with only 14 percent of Americans.
In Europe, skepticism about GM foods has long been bundled with other concerns, such as a resentment of American agribusiness. Whatever it is based on, however, the European attitude reverberates across the world, influencing policy in countries where GM crops could have tremendous benefits. “In Africa, they don't care what us savages in America are doing,” Zilberman says. “They look to Europe and see countries there rejecting GM, so they don't use it.” Forces fighting genetic modification in Europe have rallied support for “the precautionary principle,” which holds that given the kind of catastrophe that would emerge from loosing a toxic, invasive GM crop on the world, GM efforts should be shut down until the technology is proved absolutely safe.
But as medical researchers know, nothing can really be “proved safe.” One can only fail to turn up significant risk after trying hard to find it—as is the case with GM crops.
A clean record
The human race has been selectively breeding crops, thus altering plants' genomes, for millennia. Ordinary wheat has long been strictly a human-engineered plant; it could not exist outside of farms, because its seeds do not scatter. For some 60 years scientists have been using “mutagenic” techniques to scramble the DNA of plants with radiation and chemicals, creating strains of wheat, rice, peanuts and pears that have become agricultural mainstays. The practice has inspired little objection from scientists or the public and has caused no known health problems.
The difference is that selective breeding or mutagenic techniques tend to result in large swaths of genes being swapped or altered. GM technology, in contrast, enables scientists to insert into a plant's genome a single gene (or a few of them) from another species of plant or even from a bacterium, virus or animal. Supporters argue that this precision makes the technology much less likely to produce surprises. Most plant molecular biologists also say that in the highly unlikely case that an unexpected health threat emerged from a new GM plant, scientists would quickly identify and eliminate it. “We know where the gene goes and can measure the activity of every single gene around it,” Goldberg says. “We can show exactly which changes occur and which don't.”
And although it might seem creepy to add virus DNA to a plant, doing so is, in fact, no big deal, proponents say. Viruses have been inserting their DNA into the genomes of crops, as well as humans and all other organisms, for millions of years. They often deliver the genes of other species while they are at it, which is why our own genome is loaded with genetic sequences that originated in viruses and nonhuman species. “When GM critics say that genes don't cross the species barrier in nature, that's just simple ignorance,” says Alan McHughen, a plant molecular geneticist at U.C. Riverside. Pea aphids contain fungi genes. Triticale is a century-plus-old hybrid of wheat and rye found in some flours and breakfast cereals. Wheat itself, for that matter, is a cross-species hybrid. “Mother Nature does it all the time, and so do conventional plant breeders,” McHughen says.
Could eating plants with altered genes allow new DNA to work its way into our own? It is possible but hugely improbable. Scientists have never found genetic material that could survive a trip through the human gut and make it into cells. Besides, we are routinely exposed to—and even consume—the viruses and bacteria whose genes end up in GM foods. The bacterium Bacillus thuringiensis , for example, which produces proteins fatal to insects, is sometimes enlisted as a natural pesticide in organic farming. “We've been eating this stuff for thousands of years,” Goldberg says.
In any case, proponents say, people have consumed as many as trillions of meals containing genetically modified ingredients over the past few decades. Not a single verified case of illness has ever been attributed to the genetic alterations. Mark Lynas, a prominent anti-GM activist who in 2013 publicly switched to strongly supporting the technology, has pointed out that every single news-making food disaster on record has been attributed to non-GM crops, such as the Escherichia coli –infected organic bean sprouts that killed 53 people in Europe in 2011.
Critics often disparage U.S. research on the safety of genetically modified foods, which is often funded or even conducted by GM companies, such as Monsanto. But much research on the subject comes from the European Commission, the administrative body of the E.U., which cannot be so easily dismissed as an industry tool. The European Commission has funded 130 research projects, carried out by more than 500 independent teams, on the safety of GM crops. None of those studies found any special risks from GM crops.
Plenty of other credible groups have arrived at the same conclusion. Gregory Jaffe, director of biotechnology at the Center for Science in the Public Interest, a science-based consumer-watchdog group in Washington, D.C., takes pains to note that the center has no official stance, pro or con, with regard to genetically modifying food plants. Yet Jaffe insists the scientific record is clear. “Current GM crops are safe to eat and can be grown safely in the environment,” he says. The American Association for the Advancement of Science, the American Medical Association and the National Academy of Sciences have all unreservedly backed GM crops. The U.S. Food and Drug Administration, along with its counterparts in several other countries, has repeatedly reviewed large bodies of research and concluded that GM crops pose no unique health threats. Dozens of review studies carried out by academic researchers have backed that view.
Opponents of genetically modified foods point to a handful of studies indicating possible safety problems. But reviewers have dismantled almost all of those reports. For example, a 1998 study by plant biochemist Árpád Pusztai, then at the Rowett Institute in Scotland, found that rats fed a GM potato suffered from stunted growth and immune system–related changes. But the potato was not intended for human consumption—it was, in fact, designed to be toxic for research purposes. The Rowett Institute later deemed the experiment so sloppy that it refuted the findings and charged Pusztai with misconduct.
Similar stories abound. Most recently, a team led by Gilles-Éric Séralini, a researcher at the University of Caen Lower Normandy in France, found that rats eating a common type of GM corn contracted cancer at an alarmingly high rate. But Séralini has long been an anti-GM campaigner, and critics charged that in his study, he relied on a strain of rat that too easily develops tumors, did not use enough rats, did not include proper control groups and failed to report many details of the experiment, including how the analysis was performed. After a review, the European Food Safety Authority dismissed the study's findings. Several other European agencies came to the same conclusion. “If GM corn were that toxic, someone would have noticed by now,” McHughen says. “Séralini has been refuted by everyone who has cared to comment.”
Some scientists say the objections to GM food stem from politics rather than science—that they are motivated by an objection to large multinational corporations having enormous influence over the food supply; invoking risks from genetic modification just provides a convenient way of whipping up the masses against industrial agriculture. “This has nothing to do with science,” Goldberg says. “It's about ideology.” Former anti-GM activist Lynas agrees. He has gone as far as labeling the anti-GM crowd “explicitly an antiscience movement.”
Persistent doubts
Not all objections to genetically modified foods are so easily dismissed, however. Long-term health effects can be subtle and nearly impossible to link to specific changes in the environment. Scientists have long believed that Alzheimer's disease and many cancers have environmental components, but few would argue we have identified all of them.
And opponents say that it is not true that the GM process is less likely to cause problems simply because fewer, more clearly identified genes are replaced. David Schubert, an Alzheimer's researcher who heads the Cellular Neurobiology Laboratory at the Salk Institute for Biological Studies in La Jolla, Calif., asserts that a single, well-characterized gene can still settle in the target plant's genome in many different ways. “It can go in forward, backward, at different locations, in multiple copies, and they all do different things,” he says. And as U.C.L.A.'s Williams notes, a genome often continues to change in the successive generations after the insertion, leaving it with a different arrangement than the one intended and initially tested. There is also the phenomenon of “insertional mutagenesis,” Williams adds, in which the insertion of a gene ends up quieting the activity of nearby genes.
True, the number of genes affected in a GM plant most likely will be far, far smaller than in conventional breeding techniques. Yet opponents maintain that because the wholesale swapping or alteration of entire packages of genes is a natural process that has been happening in plants for half a billion years, it tends to produce few scary surprises today. Changing a single gene, on the other hand, might turn out to be a more subversive action, with unexpected ripple effects, including the production of new proteins that might be toxins or allergens.
Opponents also point out that the kinds of alterations caused by the insertion of genes from other species might be more impactful, more complex or more subtle than those caused by the intraspecies gene swapping of conventional breeding. And just because there is no evidence to date that genetic material from an altered crop can make it into the genome of people who eat it does not mean such a transfer will never happen—or that it has not already happened and we have yet to spot it. These changes might be difficult to catch; their impact on the production of proteins might not even turn up in testing. “You'd certainly find out if the result is that the plant doesn't grow very well,” Williams says. “But will you find the change if it results in the production of proteins with long-term effects on the health of the people eating it?”
It is also true that many pro-GM scientists in the field are unduly harsh—even unscientific—in their treatment of critics. GM proponents sometimes lump every scientist who raises safety questions together with activists and discredited researchers. And even Séralini, the scientist behind the study that found high cancer rates for GM-fed rats, has his defenders. Most of them are nonscientists, or retired researchers from obscure institutions, or nonbiologist scientists, but the Salk Institute's Schubert also insists the study was unfairly dismissed. He says that as someone who runs drug-safety studies, he is well versed on what constitutes a good-quality animal toxicology study and that Séralini's makes the grade. He insists that the breed of rat in the study is commonly used in respected drug studies, typically in numbers no greater than in Séralini's study; that the methodology was standard; and that the details of the data analysis are irrelevant because the results were so striking.
Schubert joins Williams as one of a handful of biologists from respected institutions who are willing to sharply challenge the GM-foods-are-safe majority. Both charge that more scientists would speak up against genetic modification if doing so did not invariably lead to being excoriated in journals and the media. These attacks, they argue, are motivated by the fear that airing doubts could lead to less funding for the field. Says Williams: “Whether it's conscious or not, it's in their interest to promote this field, and they're not objective.”
Both scientists say that after publishing comments in respected journals questioning the safety of GM foods, they became the victims of coordinated attacks on their reputations. Schubert even charges that researchers who turn up results that might raise safety questions avoid publishing their findings out of fear of repercussions. “If it doesn't come out the right way,” he says, “you're going to get trashed.”
There is evidence to support that charge. In 2009 Nature detailed the backlash to a reasonably solid study published in the Proceedings of the National Academy of Sciences USA by researchers from Loyola University Chicago and the University of Notre Dame. The paper showed that GM corn seemed to be finding its way from farms into nearby streams and that it might pose a risk to some insects there because, according to the researchers' lab studies, caddis flies appeared to suffer on diets of pollen from GM corn. Many scientists immediately attacked the study, some of them suggesting the researchers were sloppy to the point of misconduct.
A way forward
There is a middle ground in this debate. Many moderate voices call for continuing the distribution of GM foods while maintaining or even stepping up safety testing on new GM crops. They advocate keeping a close eye on the health and environmental impact of existing ones. But they do not single out GM crops for special scrutiny, the Center for Science in the Public Interest's Jaffe notes: all crops could use more testing. “We should be doing a better job with food oversight altogether,” he says.
Even Schubert agrees. In spite of his concerns, he believes future GM crops can be introduced safely if testing is improved. “Ninety percent of the scientists I talk to assume that new GM plants are safety-tested the same way new drugs are by the FDA,” he says. “They absolutely aren't, and they absolutely should be.”
Stepped-up testing would pose a burden for GM researchers, and it could slow down the introduction of new crops. “Even under the current testing standards for GM crops, most conventionally bred crops wouldn't have made it to market,” McHughen says. “What's going to happen if we become even more strict?”
That is a fair question. But with governments and consumers increasingly coming down against GM crops altogether, additional testing may be the compromise that enables the human race to benefit from those crops' significant advantages.
David H. Freedman is a journalist who has been covering science, business and technology for more than 30 years.
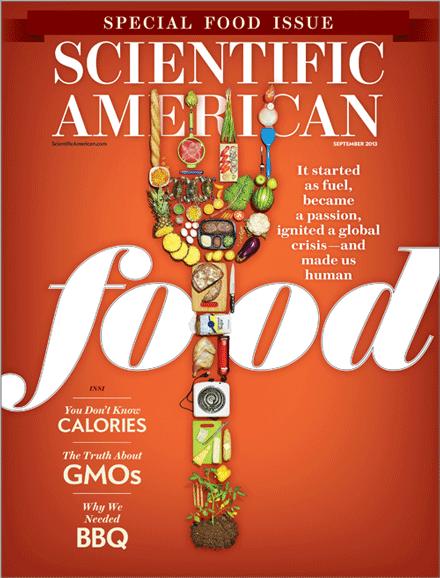
- Skip to main content
- Keyboard shortcuts for audio player

Your Health
- Treatments & Tests
- Health Inc.
- Public Health
Gardeners can now grow a genetically modified purple tomato made with snapdragon DNA
Sasa Woodruff

The Purple Tomato, a genetically modified crop created by Norfolk Plant Sciences, is available to home gardeners to start from seed. Raven Villar/Boise State Public Radio hide caption
The Purple Tomato, a genetically modified crop created by Norfolk Plant Sciences, is available to home gardeners to start from seed.
As home gardeners in the U.S. page through seed catalogs and pick out their favorite heirlooms, there's a new seed that has never been available to them before: a tomato the color of a concord grape with plum-colored flesh. It looks otherworldly, maybe Photoshopped. But it's not.
This nightshade is purple because its creators at Norfolk Plant Sciences worked for about 20 years to hack color genes from a snapdragon flower into the plant. The genes not only provide pigment, but high levels of anthocyanin, a health-promoting compound.
This dusky fruit, named the Purple Tomato, is the first genetically modified food crop to be directly marketed to home gardeners – the seeds went on sale Saturday. Last year , a handful of small farmers started growing and selling the tomatoes, but until now, genetically modified foods were generally only available to commercial producers in the U.S.
By selling direct to gardeners, Norfolk hopes to get Americans to change their perceptions of GMO foods. A 2020 Pew Research study showed that most Americans see GMOs as worse for their health than a food that has no genetic modification and just 7% see them as healthier than other foods.
"We aim to show with this product and with this company that there's a lot of benefits that can go to consumers through biotechnology, better taste, better nutrition as prime examples," says Nathan Pumplin, CEO of Norfolk Healthy Produce , a subsidiary of Norfolk Plant Sciences.
A disease-fighting tomato
The leading scientist behind the Purple Tomato is Cathie Martin, a biochemist who trained at the University of Cambridge. About 20 years ago, she set out to create a transgenic tomato, using DNA from another unrelated organism, in this case, a purple snapdragon, which is an edible flower.

Cathie Martin worked for years to develop the Purple Tomato using genes from the edible snapdragon plant to increase anthocyanin, a compound that gives a purplish hue to plants. John Innes Centre/Norfolk Plant Sciences hide caption
Cathie Martin worked for years to develop the Purple Tomato using genes from the edible snapdragon plant to increase anthocyanin, a compound that gives a purplish hue to plants.
The goal was to develop a tomato with high levels of anthocyanins , the compounds that give blueberries and blackberries, eggplant and purple cabbage their color and their status as superfoods.
Anthocyanins have been shown to have anti-cancer and anti-inflammatory effects. They're antioxidants, which can help neutralize unstable molecules in the body that can damage healthy cells and are linked with aging and disease.
"It's normal for tomatoes to make these healthy antioxidants. They typically don't make them very much in the fruit, though," Pumplin says, explaining that they normally appear in the stems and leaves . "So what Cathie [Martin] did was put the on switch into tomato."
She started with the basic technique that scientists figured out in the 1980s using a bacteria to naturally insert its DNA into host organisms.

GMO is out, 'bioengineered' is in, as new U.S. food labeling rules take effect
It's a process that can happen naturally. For example, sweet potatoes have the DNA of an agrobacterium and can technically be considered transgenic, an plant that contains genetic material of two different organisms.
Martin isolated the gene in the snapdragon flower that turned on and off the purple color. Next she took the gene and inserted it into the bacteria. The tomato could then take in the foreign genetic material and express this new gene.
"It really is a great example of understanding how the natural world functions and building on that to meet our needs," Pumplin explains.
The result? Norfolk's purple tomato has, per weight, as much anthocyanin as a blueberry or eggplant, Pumplin says. And Americans eat more tomatoes annually, so it makes the nutritional benefits more accessible.
In a research published in Nature, Martin found that mice who ate a diet supplemented with purple tomatoes lived 30% longer than those who didn't.

The Purple Tomato has deep purple flesh. Traditional breeders have grown tomatoes with purple skin before but not with this tone in the flesh. Raven Villar/Boise State Public Radio hide caption
The Purple Tomato has deep purple flesh. Traditional breeders have grown tomatoes with purple skin before but not with this tone in the flesh.
A new wave in GMO foods
The push for nutrient-dense GMOs is a recent trend, says Kathleen Hefferon, a microbiologist at Cornell University. The first wave of GMOs were for staple crops that were easier to grow.
"There was a real push of trying to achieve food security for a lot of populaces in developing countries and usually that involved making these staple crops that grew better, such as rice and corn and wheat and things like this," she explained.
A transgenic papaya was introduced to combat a virus that was destroying the crops in Hawaii. It's largely credited with saving the industry on the islands. There were also crops to increase nutritional value for populations in developing countries. Golden rice was developed in the late 1990s to have more beta-carotene to combat Vitamin A deficiencies. Because of practical and regulatory issues, the crop never took off.
The trend now is for biofortified foods, like the Purple Tomato.
"People have interest in their quality of life, for longevity and things like this. I think there has been just a health trend in that regard and it's going to continue," Hefferon says.
Along the same lines, California-based food company Fresh Del Monte created a pink pineapple in 2020. Its rosy flesh comes from a high level of lycopene, an antioxidant that gives peaches, tomatoes and watermelon their rosy hues.
But unlike the Purple Tomato, which the company is making widely available to both farmers and consumers, only Fresh Del Monte can grow it.

Purple Tomatoes ripening in the Norfolk Plant Sciences test garden. Norfolk Plant Sciences hide caption
Purple Tomatoes ripening in the Norfolk Plant Sciences test garden.
Traditional breeding vs. GMOs
Genetic modification in the lab isn't the only way to supercharge foods with nutrients , notes Jim Myers, a professor specializing in vegetable breeding at Oregon State University. He says in fact, traditional breeders were the first to release a tomato to the public with boosted levels of anthocyanins.
More than two decades ago Myers began using traditional plant breeding to cross genes from wild tomatoes with modern varieties.
The modern domesticated tomato originated from an 80,000 years old species from Ecuador . There are about 10,000 varieties of Solanum lycopersicum , which vary from marigold orange to celery green to khaki maroon
Domesticated tomatoes have anthocyanins only in the plant , but Myers says their wild relatives have them in the fruit.
He crossed Solanum cheesmaniae from the Galapagos and Solanum chilense from South America with a domesticated variety to ultimately create the Indigo collection of tomatoes.
In 2011, they released the 'Indigo Rose ,' which has a deep blue skin and a pinkish inside when ripe, and more anthocyanin.
His first version of the tomato wasn't perfect, he says – the taste wasn't great and it took a long time to ripen, but subsequent breeding has improved on it, and gardeners can buy it and grow it themselves.
"I don't know if supercharging is the right word, but we're definitely enhancing their potential to provide benefits to human health," Myers says of the series, which now includes varieties like 'Indigo Cherry Drops', Indigo Pear Drops' 'Indigo Kiwi' and 'Midnight Roma'.

As Biotech Crops Lose Their Power, Scientists Push For New Restrictions
Myers points out that he and the creator of the Purple Tomato began working on these tomatoes at about the same time and there are now more than 50 cultivars of the Indigos being grown and bred throughout the world, including small farms and big companies.
"There's just all this diversity in the Indigo market class that has come about through conventional breeding," he says. "With the GMO tomato, it's taken them all this time and more to get one variety out there."
He also thinks the Purple Tomato could face a battle for acceptance that the Indigos don't, given negative perceptions of GMOs.
"There's going to be this cognitive dissonance for some people in that here is a tomato that has these potential health benefits ... contrasting with the origins, which was through genetic engineering."

A caprese salad prepared with Purple Tomato. Norfolk Plant Sciences hide caption
A caprese salad prepared with Purple Tomato.
A new chapter in the GMO debate?
Some of the earliest GM crops were corn and soybeans modified to tolerate herbicides like glyphosate, known commercially as Roundup. In 2023, the USDA reports 91% of domestic corn acres used herbicide tolerant seeds .
Mark Lynas, author of Seeds of Science: Why We Got It So Wrong On GMOs says the abundance of chemical-tolerant plants has harmed the acceptance of this technology.
"It enabled people who were concerned about the technology to really draw the conclusion that this was all about increasing agrochemical use, and the capture of the seeds in the food chain by big multinational corporations," he says.
Lynas says it was a blow to their adoption because the industry could have focused on genetic modifications that would actually use less herbicide.
"GMO technology could have already transformed world agriculture in a vastly more sustainable direction," he says.
The Purple Tomato's creators hope its release to gardeners could change the conversation. Lynas called Norfolk's marketing to consumers a "stroke of genius" that could demystify the technology.
"Stop just doing the GMO stuff with these big corporate, commodity cash crops and do something ordinary people can have in their hands," he says. "You'll see, actually it's just a seed which is going to produce a purple fruit, which is probably healthier for you."
Of course, some people have raised health concerns around eating GMOs, but studies since these foods were introduced three decades ago do not show any harm. The U.S. Food and Drug Administration concludes there is not a health risk to eating GM foods currently on the market.
Lynas says GMOs could be used to improve the environment, and livelihoods of people around the globe.
"If we focus on that, then we can make sure that these biotechnologies actually have outcomes and applications which are better for the planet and better for people overall. And that's the way that science should be used," Lynas says.
Pumplin measures success by whether or not a large number of consumers will embrace the health benefits, color and taste of the new tomato.
"Then it chips away at this negative perception of GMOs and that will enable other products to get out to market that deliver really solid benefits," he says. Benefits that include climate change, sustainability, health and nutrition.
Sáša Woodruff reports on food and agriculture. She is the news director of Boise State Public Radio.
- genetically modified food
- anthocyanins
- purple tomato
- bioengineered food
Things you buy through our links may earn Vox Media a commission.
The Problem With Darling 58
The fight to save america’s iconic tree has become a civil war..
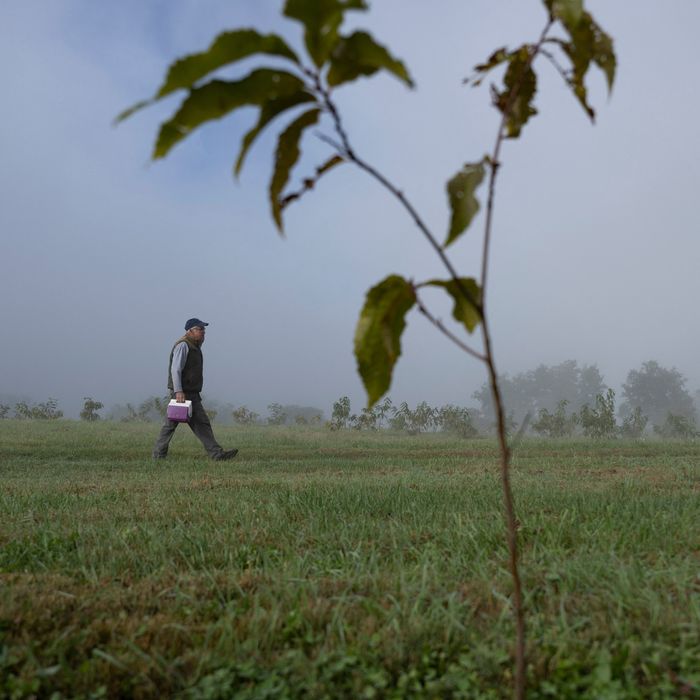
For the past two decades, Sara Fern Fitzsimmons has raised seedlings of the American chestnut in research orchards along the Eastern Seaboard, keeping them fed and hydrated and charting their growth. At the turn of the 20th century, the “redwoods of the East” dominated forests with their towering trunks, accounting for an estimated one in every four trees from southern Maine to northern Florida. They fueled a major timber industry, and their nuts were a vital source of food for both livestock and countless families. As one historian wrote, the tree “was possibly the single most important natural resource of the Appalachians.”
Last fall, Fitzsimmons noticed some of the baby trees seemed small for their age, with weak roots and curling leaves. Worse, they were getting sick as a cankerous orange fungus ate its way out of their trunks, suffering with a disease that decimated the species and to which the trees had been genetically modified to resist.
More than a few saplings died. So did the hope of rescuing the American chestnut tree from the point of near extinction, at least for now. A breakthrough in genetic engineering was intended to bring them back and transform the science of species restoration while potentially netting its inventors millions of dollars and wide acclaim. Instead, a mix-up in the lab has sparked a veritable civil war in the niche conservation community.
For the chestnut evangelists who’ve devoted years to restoration efforts, the fight to save the tree has always been personal. Now this fight is, too, amid accusations that the scientists who invented the GMO tree covered up the mistake as they sought federal approval and pursued potentially lucrative deals to sell their creation.
Tree world, says Andy Newhouse, director of the lab that invented the promised savior of the chestnut tree, “is definitely a little, little bubble. And inside that bubble, there’s a lot going on.”
In 1904, Herman W. Merkel, a forester at the Bronx Zoo, noticed chestnuts near the park’s perimeter were speckled with a strange orange fungus. Merkel called in William A. Murrill, a mycologist at the New York Botanical Garden, and the two men spent the next year identifying a fungus now known as Cryphonectria parasitica , imported on ornamental Asian chestnut trees. The blight enters via small wounds in the bark made by weather or insects and eats its way through before the trunk erupts open with a warty canker full of “yellowish-brown fruiting pustules,” which release spores to infect nearby trees, wrote Murrill. “No treatment can be suggested except the rigorous use of the pruning knife,” he determined. “The disease seems destined to run its course, as epidemics usually do.”
The blight ran through forests like a line of fire, killing close to 4 billion trees by 1940, and it still hasn’t burned out: When the viable chestnut roots below ground send up new shoots, they only live a decade or so before the fungus kills them, too. A small, determined cohort of scientists, growers, and tree lovers refused to accept the end of the chestnut epoch, and in the 1980s, two parallel rescue efforts began.
At a research farm in southwestern Virginia, growers working with the nascent American Chestnut Foundation began a breeding program, hypothesizing that crossing American chestnuts with their Chinese cousins would confer the latter’s resistance to Cryphonectria parasitica. Infected Chinese chestnuts, having evolved alongside the blight, simply wall it off and keep on growing. Subsequent “back-crossing” of the resulting hybrids over multiple generations aimed to create blight-tolerant trees that had all the characteristics of the American original.
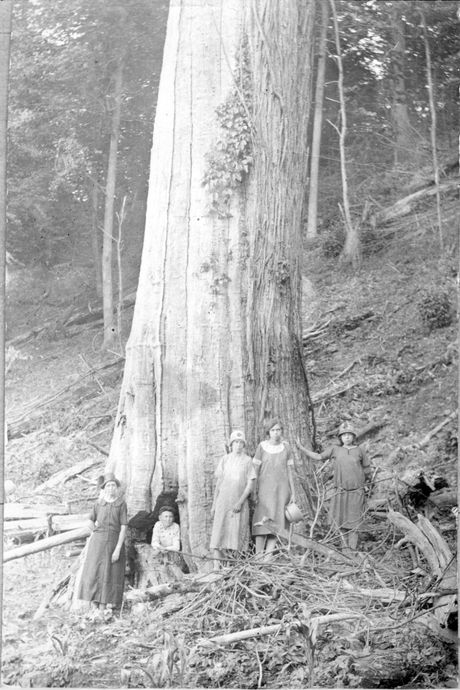
Around the same time, an engineer named Herb F. Darling Jr. found some surviving wild chestnuts on his family’s land in western New York’s Zoar Valley. He thought they might provide the basis for a much quicker solution: transgenics — inserting one organism’s DNA into another — to create a genetically modified tree. When he approached the foundation for support, it turned him away: Its official position was staunchly anti-GMO. It’s an opinion much of the conservation community has long shared. The introduction of farm GMOs like Monsanto’s “Roundup-ready” crops has increased agricultural production, but it has also created new threats to biodiversity and drastically increased usage of the trademark herbicide.
Since their inception, those commercial GMOs have been deployed with an eye toward containment. Darling was proposing using the technology much differently. “For conservation, you want it to spread,” says Will Pitt, the foundation’s current president and CEO; that only alarmed foundation leadership further.
So instead, Darling started his own organization and partnered with Bill Powell and Chuck Maynard, geneticists at the College of Environmental Science and Forestry (ESF) at the State University of New York. Powell went on to identify an enzyme in wheat plants — oxalate oxidase, or OxO — that protects them from oxalic acid, the same compound Cryphonectria parasitica produces to kill chestnuts. He would spend the next several years inserting an OxO-producing wheat gene into different places along the chestnut genome, creating iteration after iteration of what he dubbed the “Darling” line after Herb, his benefactor. In 2012, he landed on a version that seemed to convey total blight resistance without changing the American character of the trees. He dubbed the revelatory version Darling 58.
After Powell and Maynard officially published their findings in 2013, there was “a big shift” in the chestnut community, says Newhouse, who began working on Darling 58 at ESF. Supporters clamored to know when they could get seeds to plant. The foundation’s hybridization plan had produced only marginal success, and it found blight resistance more genetically complicated than expected. It announced its full support for the transgenic program and ESF, and threw its weight behind applications asking the federal government to deregulate Darling 58, allowing it to be planted, basically, anywhere and by anyone. The foundation became ESF’s primary scientific partner and financial backer, funneling the lab annual donations in the six figures.
With Darling 58’s blight-resistance properties proven in the lab, and seedlings planted at carefully monitored test sites, it was just a matter of getting the government to deregulate Powell’s creation. That would make it the first GMO designed for conservation and approved for release into wild ecosystems. The move would open a fresh chapter of species-restoration science and pave the way for transgenic solutions for all manner of endangered plants and animals. “They’re all kind of lined up behind this,” says Pitt.
In 2022, Powell was diagnosed with colon cancer and given a two-year prognosis. At the same time, he and Newhouse, his longtime protégé, began meeting with American Castanea, a newly formed company whose founders saw a huge opportunity in meeting the intense demand for seedlings they expected to follow deregulation. American Castanea would agree to pay ESF for distribution rights to sell millions of transgenic seedlings worth millions of dollars.
The foundation, however, balked at the potential involvement of a for-profit company after repeated insistence from Powell that rights to Darling 58 would remain in the public domain. Internally, the leadership referred to Powell’s deal with American Castanea as “the betrayal.” They met with SUNY leadership, threatening to dissolve the partnership if the deal was made official. Soon after, Newhouse says, he was “uninvited” from the foundation’s annual meeting. “That was definitely a big red flag.”
Meanwhile, the foundation’s scientists were growing concerned at Darling 58 test sites. Many of the trees seemed stunted and unhealthy. Their leaves were browning and folding in on themselves, and a surprising number were dying, succumbing to the fungal blight they should have been able to resist.
The scientists at the foundation raised their concerns with Newhouse and ESF and pushed for the lab’s newest research about the performance of Darling 58. What information they received felt incomplete, and some began to wonder if ESF was hiding something. “We have weekly science calls they’ve been on since 2019,” says Sarah Fern Fitzsimmons, the foundation’s chief conservation officer. “There’s a history of not being transparent with data. I look back through the reports they compiled for us for the grants we gave them, and everything’s awesome: It’s cherry-picking the good and not letting on that anything was amiss at all.”
Last spring, while foundation scientists in the field were wondering what could be wrong with the trees, Thomas Klak, an environmental-science professor at the University of New England in Portland, Maine, was struggling to produce Darling 58 plants with two copies of the OxO gene. He reached out to Ek Han Tan, a geneticist at the University of Maine who developed a test to analyze their genome.
“The line that Tom has been using — that everyone has been using — was supposedly derived from Darling 58, and there was a good genetic map of the transgene on chromosome seven,” Tan says. But when he couldn’t find that gene on any of Klak’s samples, he started to wonder if they all might have the wrong tree.
Eventually, Tan found the trees’ OxO gene on chromosome four — the insertion point for an earlier transgenic iteration called Darling 54. For the past decade, the many scientists trying to save the chestnut had been working with the wrong tree. Functionally, Darling 58, the tree touted as the great hope of the chestnut and the next frontier in species restoration, did not exist.
In October 2023, Klak and Tan broke the news to Newhouse and his ESF colleagues. Newhouse says ESF began working to confirm, as initial tests weren’t “entirely consistent” with the hypothesis that the trees were Darling 54. Nearly a month later, after following up repeatedly with the ESF team, Tan looped in the foundation’s science director. It was the first the foundation had heard of the major mix-up.
Fitzsimmons says ESF chalked it up to mistaken identity when the first generation of transgenic clone trees were made. “You think you’re getting pollen from a Darling 58 tree, but you actually got it from Darling 54,” she says. “So, you take that pollen and put it on chestnuts in the field and you assume everything subsequently will be 58. But everything derived from that initial pollination.”
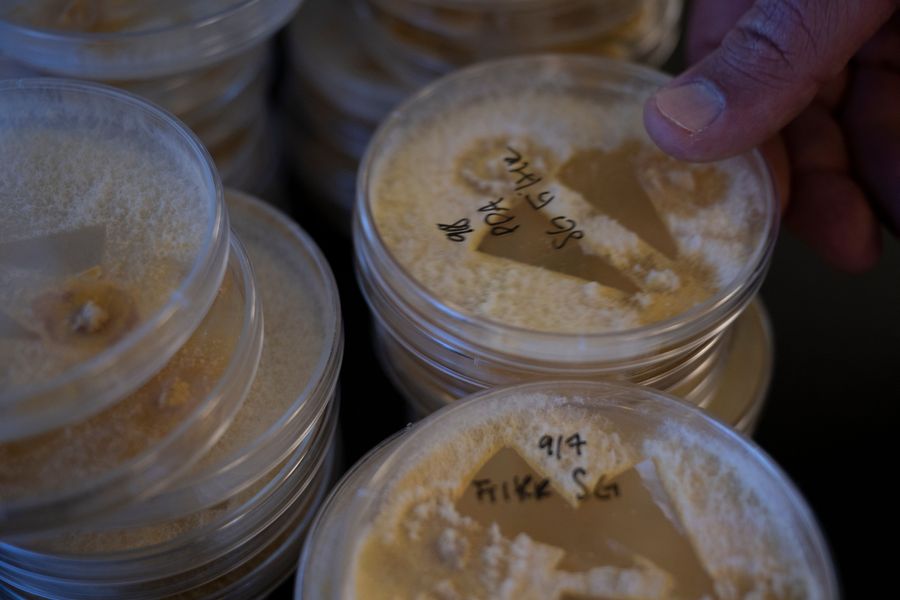
Six days after Tan alerted the foundation, on November 12, Powell died. He never knew that he’d spent years planting the wrong tree, Newhouse says. By this point, the partnership he’d forged between ESF and the foundation was collapsing over his creation.
ESF, though, was undeterred by the startling discovery about Darling 58 and announced a $636,000 grant from the USDA to support studies of the “performance of Darling 58 chestnut trees as they start to mature in real-world conditions,” but made no announcement about Klak and Tan’s discovery. It forged ahead with getting approval from the FDA and EPA as well. On December 8, the foundation decided to blow the whistle. It issued a press release calling the Darling trees “unsuitable as the basis for species restoration,” withdrawing support for deregulation, and declining to further fund the line’s development. The potential deal with American Castanea was dead.
“To this day, we’ve never heard anything directly from ESF,” says Pitt, the American Chestnut Foundation’s president. If Tan and Klak hadn’t shared their findings, Pitt wonders if ESF ever would have “told us, told the public, told anyone.” “As a nonprofit organization, we can’t hide things from our members or the public. If we wouldn’t have brought this out, we would be complicit with a cover-up.”
Pitt estimates the foundation has funneled close to $3 million to ESF over the last decade. Given that, the pending deregulation applications, the USDA grant, and rumors of an additional million dollars promised to ESF upon deregulation from another donor, he says there were “more than a million reasons” for ESF to sweep the error under the rug. “The stakes are extremely high: If this was successful, ESF would be world-renowned.”
It’s necessary to demonstrate success, Newhouse concedes, “in order to keep doing the research. But you don’t want to overstate, and that’s the tightrope.” He maintains that any delay was because ESF was doing its own testing on the trees. “It wasn’t that we sat on things or tried to cover them up,” he says. “We wanted to be sure of what we had and not share speculative information.”
But Pitt recalls a conversation in the fall — before the Darling revelation — where Newhouse and other ESF leadership were discussing plans to establish a major research institute. “I said, maybe the problem is we have two different goals,” Pitt recounts. “If you’re successful, you’re going to build a bigger forestry institute. If I’m successful, I’m going to be out of a job. That’s what success looks like to me; that I’m no longer needed. That is a very different way of looking at the world.”
Newhouse has repeatedly said the mix-up is little more than a naming error. While Darling 54 doesn’t appear to offer the same blight resistance Darling 58 promised, it might still be able to tolerate the fungus a bit longer than an entirely wild American tree. Though Newhouse concedes it’s not the tree that’ll rescue the species, it’s still “promising,” he says, and ESF is forging ahead with deregulation efforts. He has provided updated data to the USDA and said he doesn’t think the applications should be drastically affected. “The series of environmental tests we’ve done were actually done with Darling 54; some knowingly, and some when we thought it was 58,” he said. “We’ve seen that it’s not detrimental, it’s not harmful to other organisms.”
Fitzsimmons is not so sure. In addition to evidence of lower-than-expected blight resistance, Darling 54’s chromosome tweak causes the deletion of more than 1,000 DNA base pairs, the ultimate effect of which is hard to know. “It’s not something you want to deploy into a restoration population,” she says.
For a group of people who have dedicated decades to the American chestnut’s rescue, the last few months have been an emotional tumult. “It’s heartbreaking that we’re not further along,” Pitt says. “This wasn’t the silver bullet, but we thought it was a big step.”
Most Viewed Stories
- Prosecutors Got Trump — But They Also Contorted the Law
- What the Polls Are Saying After Trump’s Conviction
- Why Does the New Kate Middleton Portrait Look Nothing Like Her?
- Melania Won’t Be Bullied Into Supporting Trump After Verdict
- What It Was Like in Court the Moment Trump Was Convicted
Editor’s Picks
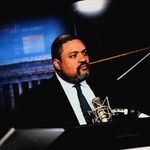
Most Popular
- Prosecutors Got Trump — But They Also Contorted the Law By Elie Honig
- What the Polls Are Saying After Trump’s Conviction By Chas Danner
- Why Does the New Kate Middleton Portrait Look Nothing Like Her? By Margaret Hartmann
- Melania Won’t Be Bullied Into Supporting Trump After Verdict By Margaret Hartmann
- What It Was Like in Court the Moment Trump Was Convicted By Andrew Rice
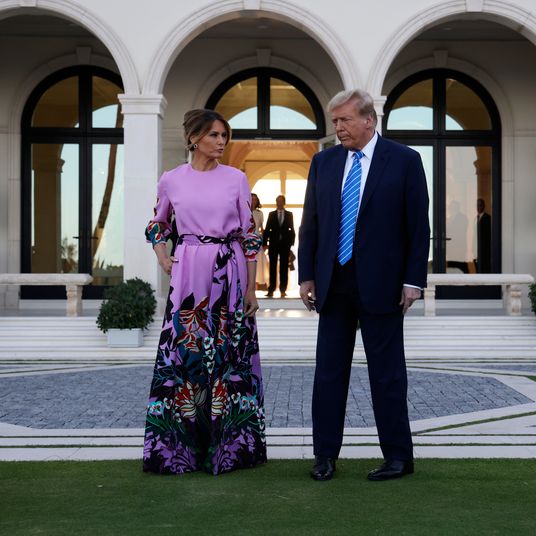
What is your email?
This email will be used to sign into all New York sites. By submitting your email, you agree to our Terms and Privacy Policy and to receive email correspondence from us.
Sign In To Continue Reading
Create your free account.
Password must be at least 8 characters and contain:
- Lower case letters (a-z)
- Upper case letters (A-Z)
- Numbers (0-9)
- Special Characters (!@#$%^&*)
As part of your account, you’ll receive occasional updates and offers from New York , which you can opt out of anytime.

Researchers expose new symbiosis origin theories, identify experimental systems for plant life
A Mississippi State faculty member's work on symbiosis -- a mutually beneficial relationship between living organisms -- is pushing back against the newer theory of a "single-origin" of root nodule symbiosis (RNS) -- that all symbiosis between plant root nodules and nitrogen-fixing bacteria stems from one point -- instead suggesting a "multiple-origin" theory of symbiosis which opens a better understanding for genetically engineering crops.
Ryan A. Folk, an assistant professor in the MSU Department of Biological Sciences and herbarium curator, is an author on a paper published this month in Nature Communications examining (RNS), which allows plants to access atmospheric nitrogen converted into usable forms through a mutualistic relationship with soil bacteria. He joins investigators at the University of Florida and an international team.
"A story of a single origin has become very popular in recent years, particularly among those hoping to genetically engineer symbiosis in crop plants, but using genomic data from 13,000 species and sophisticated statistical models, we confidently identified a scenario involving multiple origins. Symbiosis is a complex trait and our work identifies ideal experimental systems for better understanding the molecular mechanisms that led to the gain of symbiosis," Folk said. "Our work is the first major push-back against the idea of a single origin as advocated by those working on genome comparisons."
Folk said the single origin idea would suggest that the genetic engineering of crops, such as rice and maize, to work with nitrogen-fixing bacteria is a "lower hurdle" to cross.
"Our results, which point to multiple origins, complicates the picture because it suggests a lesser role for shared genetic machinery," Folk said. "This would make it harder to transform crop plants that are not legumes to engage in a similar nitrogen-fixing symbiosis, but multiple origins also means diverse machinery, or as we argue, an enhanced 'evolutionary palette' to guide such experiments," he said.
Folk's paper laid the groundwork for his fall 2023 $1.6 National Science Foundation grant for detailed study of nitrogen conversions in nature.
Folk's MSU lab uses genomic and bioinformatic techniques to document the origins of plant diversity from evolutionary and ecological perspectives using a variety of plant groups and habitats. His work is based in MSU's herbarium, housing approximately 38,000 vascular plant specimens from around the world with an emphasis on the Southeastern U.S.
- Biotechnology and Bioengineering
- Endangered Plants
- Genetically Modified
- Geochemistry
- Exotic Species
- Purple loosestrife
- Root vegetable
- Origin of life
- Microorganism
Story Source:
Materials provided by Mississippi State University . Note: Content may be edited for style and length.
Journal Reference :
- Heather R. Kates, Brian C. O’Meara, Raphael LaFrance, Gregory W. Stull, Euan K. James, Shui-Yin Liu, Qin Tian, Ting-Shuang Yi, Daniel Conde, Matias Kirst, Jean-Michel Ané, Douglas E. Soltis, Robert P. Guralnick, Pamela S. Soltis, Ryan A. Folk. Shifts in evolutionary lability underlie independent gains and losses of root-nodule symbiosis in a single clade of plants . Nature Communications , 2024; 15 (1) DOI: 10.1038/s41467-024-48036-3
Cite This Page :
Explore More
- How Statin Therapy May Prevent Cancer
- Origins of 'Welsh Dragons' Exposed
- Resting Brain: Neurons Rehearse for Future
- Observing Single Molecules
- A Greener, More Effective Way to Kill Termites
- One Bright Spot Among Melting Glaciers
- Martian Meteorites Inform Red Planet's Structure
- Volcanic Events On Jupiter's Moon Io: High Res
- What Negative Adjectives Mean to Your Brain
- 'Living Bioelectronics' Can Sense and Heal Skin
Trending Topics
Strange & offbeat.

IMAGES
VIDEO
COMMENTS
The global yearly net income increased by 34.3% in 2010-2012. 13,14 Furthermore, while increasing global yield by 22%, GM crops reduced pesticide (active ingredient) usage by 37% and environmental impact (insecticide and herbicide use) by 18%. 15 To achieve the same yield standards more than 300 million acres of conventional crops would have ...
Main conclusion While transgenic technology has heralded a new era in crop improvement, several concerns have precluded their widespread acceptance. Alternative technologies, such as cisgenesis and genome-editing may address many of such issues and facilitate the development of genetically engineered crop varieties with multiple favourable traits. Abstract Genetic engineering and plant ...
Genetically modified food, crops and GMO issues. Read current science articles on genetic engineering including mice with glowing hearts, disease-resistant mosquitos, GM bacteria chips and more.
While substantial research has been invested in producing new varieties of select crops (for example, ... Klümper, W. & Qaim, M. A meta-analysis of the impacts of genetically modified crops.
The current status and prospects of genetically modified (GM) and gene-edited (GE) food crops indicate their potential to revolutionize agriculture and address global food security challenges. These technologies have significantly enhanced crop traits, such as pest and disease resistance, improved nutritional content, and increased tolerance to ...
Background Despite the rapid adoption of genetically modified (GM) crops by farmers in many countries, controversies about this technology continue. Uncertainty about GM crop impacts is one reason for widespread public suspicion. Objective We carry out a meta-analysis of the agronomic and economic impacts of GM crops to consolidate the evidence. Data Sources Original studies for inclusion were ...
A systematic review of animal and human studies was conducted on genetically modified (GM) food consumption to assess its safety in terms of adverse effects/events to inform public concerns and future research. Seven electronic databases were searched from January 1st 1983 till July 11th 2020 for in vivo, animal and human studies on the incidence of adverse effects/events of GM products ...
The USA, Brazil, Argentina, Canada, and India planted about 91% of the global GM crop area of 190.4 million hectares. Soybean, maize, cotton, and canola are extensively grown and are of the utmost importance among all GM food crops. On six continents, approximately 32 plants were released for commercial cultivation.
However, a recent meta-analysis of 15 studies reveals that, on average, two-thirds of the benefits of first-generation genetically modified crops are shared downstream, whereas only one-third ...
Genetically Engineered (GE) seeds were commercially introduced in the United States for major field crops in 1996, with adoption rates increasing rapidly in the years that followed.Currently, over 90 percent of U.S. corn, upland cotton, and soybeans are produced using GE varieties. GE crops are broadly classified in this data product as herbicide-tolerant (HT), insect-resistant (Bt), or ...
Yet unintended consequences of any new crop cultivar (GM or non-GM) ... Genetically modified crops - expected benefits from traits. While the first GM crop ... They proposed that research should be focused on areas of plant science, e.g. nutrition, policy research, governance, and solutions close to local market conditions if the goal is to ...
GM crops are being developed to be more disease-resistant, to have enhanced nutritional value, increased drought tolerance and improved uptake of nutrients such as nitrogen. They are being tested in the laboratory or in contained field trials - in which plants are grown in an area to prevent spread into the environment.
FULL STORY. Populations of canola plants genetically engineered to be resistant to herbicides can survive outside of farms, but may be gradually losing their engineered genes, reports a new study ...
Genetic modification in plants was first recorded 10,000 years ago in Southwest Asia where humans first bred plants through artificial selection and selective breeding. Since then, advancements in agriculture science and technology have brought about the current GM crop revolution. GM crops are promising to mitigate current and future problems ...
(Beyond Pesticides, May 31, 2024) Among the many promises that have been made by agribusiness to farmers and consumers, the glories of crops genetically engineered (GE) to resist pests stand out. GE tools—genes—were touted as "natural," and promised to reduce the use of toxic pesticides. The ...
Percentage of genetically modified crops in the U.S. in 1997, 2018, 2019, and 2020, by type (as percent of total acreage) Premium Statistic U.S. acreage of genetically modified corn 2014-2019
Nearly all the corn and soybeans grown in the U.S. are genetically modified, but only two GM crops, Monsanto's MON810 maize and BASF's Amflora potato, are accepted in the European Union. Ten E.U ...
The genes not only provide pigment, but high levels of anthocyanin, a health-promoting compound. This dusky fruit, named the Purple Tomato, is the first genetically modified food crop to be ...
Magna Scientia Advanced Research and Reviews, 2023, 07(01), 103-128 ... public concern in case of edible crop plants [3]. In recent times, genome editing technique in plants has paved new ... Commercialization of genetically modified crops . It has been reported that genetic modified crops were cultivated in 1994 and subsequently, in 1996 ...
For the past decade, the many scientists trying to save the chestnut had been working with the wrong tree. Functionally, Darling 58, the tree touted as the great hope of the chestnut and the next ...
"A story of a single origin has become very popular in recent years, particularly among those hoping to genetically engineer symbiosis in crop plants, but using genomic data from 13,000 species ...
Introduction. In late December 2023, the People's Republic of China's (PRC's) Ministry of Agriculture and Rural Affairs (MARA) approved 37 genetically modified (GM) corn varieties and 14 GM soybean varieties after a three-year trial. These varieties were bred for stronger herbicide and insect resistance and produce higher yields compared ...