Thank you for visiting nature.com. You are using a browser version with limited support for CSS. To obtain the best experience, we recommend you use a more up to date browser (or turn off compatibility mode in Internet Explorer). In the meantime, to ensure continued support, we are displaying the site without styles and JavaScript.
- View all journals
- Explore content
- About the journal
- Publish with us
- Sign up for alerts
- Published: 22 October 2021

High carrier mobility in graphene doped using a monolayer of tungsten oxyselenide
- Min Sup Choi 1 , 2 na1 ,
- Ankur Nipane 3 na1 ,
- Brian S. Y. Kim 1 na1 ,
- Mark E. Ziffer 4 ,
- Ipshita Datta 3 ,
- Abhinandan Borah 3 ,
- Younghun Jung 1 ,
- Bumho Kim 1 ,
- Daniel Rhodes 1 ,
- Apoorv Jindal 5 ,
- Zachary A. Lamport 3 ,
- Myeongjin Lee 2 ,
- Amirali Zangiabadi ORCID: orcid.org/0000-0003-3586-0687 6 ,
- Maya N. Nair ORCID: orcid.org/0000-0001-6532-8208 7 ,
- Takashi Taniguchi ORCID: orcid.org/0000-0002-1467-3105 8 ,
- Kenji Watanabe ORCID: orcid.org/0000-0003-3701-8119 8 ,
- Ioannis Kymissis 3 ,
- Abhay N. Pasupathy ORCID: orcid.org/0000-0002-2744-0634 5 ,
- Michal Lipson ORCID: orcid.org/0000-0003-2903-3765 3 ,
- Xiaoyang Zhu 4 ,
- Won Jong Yoo ORCID: orcid.org/0000-0002-3767-7969 2 ,
- James Hone ORCID: orcid.org/0000-0002-8084-3301 1 &
- James T. Teherani ORCID: orcid.org/0000-0002-7778-8073 3
Nature Electronics volume 4 , pages 731–739 ( 2021 ) Cite this article
8559 Accesses
42 Citations
113 Altmetric
Metrics details
- Electronic devices
- Optical properties and devices
An Author Correction to this article was published on 24 November 2021
This article has been updated
Doped graphene could be of use in next-generation electronic and photonic devices. However, chemical doping cannot be precisely controlled in the material and leads to external disorder that diminishes carrier mobility and conductivity. Here we show that graphene can be efficiently doped using a monolayer of tungsten oxyselenide (TOS) that is created by oxidizing a monolayer of tungsten diselenide. When the TOS monolayer is in direct contact with graphene, a room-temperature mobility of 2,000 cm 2 V −1 s −1 at a hole density of 3 × 10 13 cm −2 is achieved. Hole density and mobility can also be controlled by inserting tungsten diselenide interlayers between TOS and graphene, where increasing the layers reduces the disorder. With four layers, a mobility value of around 24,000 cm 2 V −1 s −1 is observed, approaching the limit set by acoustic phonon scattering, resulting in a sheet resistance below 50 Ω sq −1 . To illustrate the potential of our approach, we show that TOS-doped graphene can be used as a transparent conductor in a near-infrared (1,550 nm) silicon nitride photonic waveguide and ring resonator.
This is a preview of subscription content, access via your institution
Access options
Access Nature and 54 other Nature Portfolio journals
Get Nature+, our best-value online-access subscription
24,99 € / 30 days
cancel any time
Subscribe to this journal
Receive 12 digital issues and online access to articles
111,21 € per year
only 9,27 € per issue
Buy this article
- Purchase on Springer Link
- Instant access to full article PDF
Prices may be subject to local taxes which are calculated during checkout
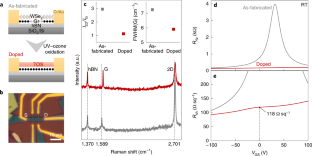
Similar content being viewed by others
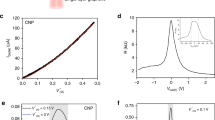
Ultra-long carrier lifetime in neutral graphene-hBN van der Waals heterostructures under mid-infrared illumination
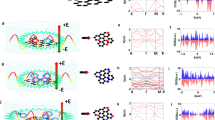
Microwave graphitic nitrogen/boron ultradoping of graphene
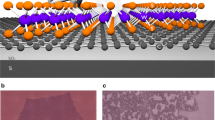
Ultrafast hot carrier transfer in WS2/graphene large area heterostructures
Data availability.
The data that support the findings within this paper are available from the corresponding authors upon reasonable request.
Change history
24 november 2021.
A Correction to this paper has been published: https://doi.org/10.1038/s41928-021-00696-5
Pierret, R. F. Semiconductor Device Fundamentals (Addison-Wesley, 1996).
Seabaugh, A. C. & Zhang, Q. Low-voltage tunnel transistors for beyond CMOS logic. Proc. IEEE 98 , 2095–2110 (2010).
Article Google Scholar
Shen, L. et al. Ohmic contacts with ultra-low optical loss on heavily doped n-type InGaAs and InGaAsP for InP-based photonic membranes. IEEE Photon. J. 8 , 4500210 (2016).
Ma, L.-P. et al. Pushing the conductance and transparency limit of monolayer graphene electrodes for flexible organic light-emitting diodes. Proc. Natl Acad. Sci. USA 117 , 25991–25998 (2020).
Low, T. & Avouris, P. Graphene plasmonics for terahertz to mid-infrared applications. ACS Nano 8 , 1086–1101 (2014).
Jena, D. & Konar, A. Enhancement of carrier mobility in semiconductor nanostructures by dielectric engineering. Phys. Rev. Lett. 98 , 136805 (2007).
Schedin, F. et al. Detection of individual gas molecules adsorbed on graphene. Nat. Mater. 6 , 652–655 (2007).
D’Arsié, L. et al. Stable, efficient p-type doping of graphene by nitric acid. RSC Adv. 6 , 113185–113192 (2016).
Yager, T. et al. High mobility epitaxial graphene devices via aqueous-ozone processing. Appl. Phys. Lett. 106 , 063503 (2015).
Mehta, A. N. et al. Understanding noninvasive charge transfer doping of graphene: a comparative study. J. Mater. Sci.: Mater. Electron. 29 , 5239–5252 (2018).
Google Scholar
Lee, H., Paeng, K. & Kim, I. S. A review of doping modulation in graphene. Synthetic Met. 244 , 36–47 (2018).
Chen, K. et al. Air stable n-doping of WSe 2 by silicon nitride thin films with tunable fixed charge density. APL Mater. 2 , 092504 (2014).
McClellan, C. J., Yalon, E., Smithe, K. K. H., Suryavanshi, S. V. & Pop, E. High current density in monolayer MoS 2 doped by AlO x . ACS Nano 15 , 1587–1596 (2021).
Nipane, A. et al. Damage-free atomic layer etch of WSe 2 : a platform for fabricating clean two-dimensional devices. ACS Appl. Mater. Interfaces 13 , 1930–1942 (2021).
Pang, C.-S. et al. Atomically controlled tunable doping in high-performance WSe 2 devices. Adv. Electron. Mater. 6 , 1901304 (2020).
Dingle, R., Störmer, H. L., Gossard, A. C. & Wiegmann, W. Electron mobilities in modulation-doped semiconductor heterojunction superlattices. Appl. Phys. Lett. 33 , 665–667 (1978).
Son, S. et al. Strongly adhesive dry transfer technique for van der Waals heterostructure. 2D Mater. 7 , 041005 (2020).
Wang, L. et al. One-dimensional electrical contact to a two-dimensional material. Science 342 , 614–617 (2013).
Yamamoto, M. et al. Self-limiting layer-by-layer oxidation of atomically thin WSe 2 . Nano Lett. 15 , 2067–2073 (2015).
Wang, H., Wu, Y., Cong, C., Shang, J. & Yu, T. Hysteresis of electronic transport in graphene transistors. ACS Nano 4 , 7221–7228 (2010).
Das, A. et al. Monitoring dopants by Raman scattering in an electrochemically top-gated graphene transistor. Nat. Nanotechnol. 3 , 210–215 (2008).
Finney, N. R. et al. Tunable crystal symmetry in graphene–boron nitride heterostructures with coexisting moiré superlattices. Nat. Nanotechnol. 14 , 1029–1034 (2019).
Sen, H. S., Sahin, H., Peeters, F. M. & Durgun, E. Monolayers of MoS 2 as an oxidation protective nanocoating material. J. Appl. Phys. 116 , 083508 (2014).
Yamamoto, M., Nakaharai, S., Ueno, K. & Tsukagoshi, K. Self-limiting oxides on WSe 2 as controlled surface acceptors and low-resistance hole contacts. Nano Lett. 16 , 2720–2727 (2016).
Wang, M. et al. Breaking the doping limit in silicon by deep impurities. Phys. Rev. Appl. 11 , 054039 (2019).
Amet, F. et al. Composite fermions and broken symmetries in graphene. Nat. Commun. 6 , 5838 (2015).
Dankerl, M. et al. Graphene solution-gated field-effect transistor array for sensing applications. Adv. Funct. Mater. 20 , 3117–3124 (2010).
Zhao, J. et al. Application of sodium-ion-based solid electrolyte in electrostatic tuning of carrier density in graphene. Sci. Rep. 7 , 3168 (2017).
Hess, L. H. et al. High-transconductance graphene solution-gated field effect transistors. Appl. Phys. Lett. 99 , 033503 (2011).
Brown, M. A., Crosser, M. S., Leyden, M. R., Qi, Y. & Minot, E. D. Measurement of high carrier mobility in graphene in an aqueous electrolyte environment. Appl. Phys. Lett. 109 , 093104 (2016).
Adam, S., Hwang, E. H., Galitski, V. M. & Sarma, S. D. A self-consistent theory for graphene transport. Proc. Natl Acad. Sci. USA 104 , 18392–18397 (2007).
Browning, A. et al. Evaluation of disorder introduced by electrolyte gating through transport measurements in graphene. Appl. Phys. Express 9 , 065102 (2016).
Hwang, E. H., Adam, S. & Sarma, S. D. Carrier transport in two-dimensional graphene layers. Phys. Rev. Lett. 98 , 186806 (2007).
Xu, Y. et al. Creation of moiré bands in a monolayer semiconductor by spatially periodic dielectric screening. Nat. Mater. 20 , 645–649 (2021).
Ruta, F. L., Sternbach, A. J., Dieng, A. B., McLeod, A. S. & Basov, D. N. Quantitative nanoinfrared spectroscopy of anisotropic van der Waals materials. Nano Lett. 20 , 7933–7940 (2020).
Sze, S. M. & Ng, K. K. Physics of Semiconductor Devices (John Wiley & Sons, 2006).
Mews, M., Korte, L. & Rech, B. Oxygen vacancies in tungsten oxide and their influence on tungsten oxide/silicon heterojunction solar cells. Sol. Energy Mater. Sol. Cells 158 , 77–83 (2016).
Lee, J. E., Ahn, G., Shim, J., Lee, Y. S. & Ryu, S. Optical separation of mechanical strain from charge doping in graphene. Nat. Commun. 3 , 1024 (2012).
Nouchi, R. & Ikeda, K. I. Adsorbates as a charge-carrier reservoir for electrostatic carrier doping to graphene. Appl. Phys. Express 13 , 015005 (2020).
Wu, G. et al. Programmable transition metal dichalcogenide homojunctions controlled by nonvolatile ferroelectric domains. Nat. Electron. 3 , 43–50 (2020).
Borah, A., Sebastian, P. J., Nipane, A. & Teherani, J. T. An intuitive equivalent circuit model for multilayer van der Waals heterostructures. IEEE Tran. Electron Devices 65 , 4209–4215 (2018).
Edelberg, D. et al. Approaching the intrinsic limit in transition metal diselenides via point defect control. Nano Lett. 19 , 4371–4379 (2019).
Micallef, F. G. et al. Transparent conductors for mid-infrared liquid crystal spatial light modulators. Thin Solid Films 660 , 411–420 (2018).
Tohsophon, T., Dabirian, A., De Wolf, S., Morales-Masis, M. & Ballif, C. Environmental stability of high-mobility indium-oxide based transparent electrodes. APL Mater. 3 , 116105 (2015).
Morales-Masis, M. et al. Highly conductive and broadband transparent Zr-doped In 2 O 3 as front electrode for solar cells. IEEE J. Photovolt. 8 , 1202–1207 (2018).
Silva, É. Pd, Chaves, M., Durrant, S. F., Lisboa-Filho, P. N. & Bortoleto, J. R. R. Morphological and electrical evolution of ZnO: Al thin films deposited by RF magnetron sputtering onto glass substrates. Mater. Res. 17 , 1384–1390 (2014).
Dhakal, T. et al. Transmittance from visible to mid infra-red in AZO films grown by atomic layer deposition system. Sol. Energy 86 , 1306–1312 (2012).
Datta, I., Phare, C. T., Dutt, A., Mohanty, A. & Lipson, M. Integrated graphene electro-optic phase modulator. In Conference on Lasers Electro-Optics , STu3N.5 (OSA, Washington DC, 2017).
Yariv, A. Universal relations for coupling of optical power between microresonators and dielectric waveguides. Electron. Lett. 36 , 321–322 (2000).
Amin, R. et al. Sub-wavelength GHz-fast broadband ITO Mach–Zehnder modulator on silicon photonics. Optica 7 , 333–335 (2020).
Download references
Acknowledgements
We acknowledge fruitful discussions with S. Chae, X. Xu, K. Yao, J. Schuck and E. Hwang, as well as experimental support from L. D’Cruz. This work was primarily supported by the NSF MRSEC Program at Columbia in the Center for Precision-Assembled Quantum Materials (DMR-2011736 and DMR-1420634), in partnership with the National Research Foundation of Korea through the Global Research Laboratory (GRL) program (2016K1A1A2912707) and Research Fellow program (2018R1A6A3A11045864). Device characterization was supported by the National Science Foundation through the CAREER Award (ECCS-1752401). This work was performed in part at the Advanced Science Research Center NanoFabrication Facility at the Graduate Center of the City University of New York and in part at the Columbia Nano Initiative (CNI) Shared Lab Facilities at Columbia University. Synthesis of hBN (K.W. and T.T.) was supported by the MEXT Element Strategy Initiative to Form Core Research Center (grant number JPMXP0112101001) and the CREST(JPMJCR15F3), JST.
Author information
These authors contributed equally: Min Sup Choi, Ankur Nipane, Brian S. Y. Kim.
Authors and Affiliations
Department of Mechanical Engineering, Columbia University, New York, NY, USA
Min Sup Choi, Brian S. Y. Kim, Younghun Jung, Bumho Kim, Daniel Rhodes & James Hone
SKKU Advanced Institute of Nano Technology, Sungkyunkwan University, Suwon, Korea
Min Sup Choi, Myeongjin Lee & Won Jong Yoo
Department of Electrical Engineering, Columbia University, New York, NY, USA
Ankur Nipane, Ipshita Datta, Abhinandan Borah, Zachary A. Lamport, Ioannis Kymissis, Michal Lipson & James T. Teherani
Department of Chemistry, Columbia University, New York, NY, USA
Mark E. Ziffer & Xiaoyang Zhu
Department of Physics, Columbia University, New York, NY, USA
Apoorv Jindal & Abhay N. Pasupathy
Department of Applied Physics and Applied Mathematics, Columbia University, New York, NY, USA
Amirali Zangiabadi
Nanoscience Initiative, Advanced Science Research Center, CUNY, New York, NY, USA
Maya N. Nair
National Institute for Materials Science, Tsukuba, Japan
Takashi Taniguchi & Kenji Watanabe
You can also search for this author in PubMed Google Scholar
Contributions
M.S.C., A.N. and Y.J. designed and conducted the initial experiments under the supervision of J.H. and J.T.T. M.S.C. and A.N. stacked, fabricated and conducted the electrical, optical and material characterizations of the samples with B.S.Y.K.’s contribution. B.S.Y.K. established a model for a work-function-mismatch-based doping mechanism. M.E.Z. and X.Z. contributed to the transmittance measurement of CVD samples. I.D. and M. Lipson contributed to the optical loss measurements of TOS-doped graphene integrated into the SiN waveguide. A.B. contributed to the doping study of DNTT. B.K. and D.R. provided flux-grown WSe 2 crystals. A.J. and A.N.P. provided the SWCNT grown on SiO 2 /Si. Z.A.L. and I.K. provided the grown DNTT on TOS. M. Lee contributed to the sample preparation. A.Z. contributed to the transmission electron microscopy, SAED and energy-dispersive X-ray spectroscopy measurements. M.N.N. contributed to the XPS measurements of CVD samples. T.T. and K.W. provided the hBN crystals. W.J.Y. contributed to discussions of the results. M.S.C., A.N. and B.S.Y.K. wrote the manuscript under the supervision of J.H. and J.T.T.
Corresponding authors
Correspondence to James Hone or James T. Teherani .
Ethics declarations
Competing interests.
M.S.C., A.N., A.B., Y.J., J.H. and J.T.T. have filed for a US non-provisional patent application no. 17/236,404 regarding the technology reported in this article. The remaining authors declare no competing interests.
Additional information
Peer review information Nature Electronics thanks Jin Sik Choi and the other, anonymous, reviewer(s) for their contribution to the peer review of this work.
Publisher’s note Springer Nature remains neutral with regard to jurisdictional claims in published maps and institutional affiliations.
Supplementary information
Supplementary information.
Supplementary Figs. 1–16 and Tables 1 and 2.
Rights and permissions
Reprints and permissions
About this article
Cite this article.
Choi, M.S., Nipane, A., Kim, B.S.Y. et al. High carrier mobility in graphene doped using a monolayer of tungsten oxyselenide. Nat Electron 4 , 731–739 (2021). https://doi.org/10.1038/s41928-021-00657-y
Download citation
Received : 14 December 2020
Accepted : 15 September 2021
Published : 22 October 2021
Issue Date : October 2021
DOI : https://doi.org/10.1038/s41928-021-00657-y
Share this article
Anyone you share the following link with will be able to read this content:
Sorry, a shareable link is not currently available for this article.
Provided by the Springer Nature SharedIt content-sharing initiative
This article is cited by
Ultrafast charge transfer in mixed-dimensional wo3-x nanowire/wse2 heterostructures for attomolar-level molecular sensing.
- Junyang Tan
Nature Communications (2023)
Exploring the electronic and thermal properties of S-graphene: robustness effects, magnetic fields, and doping
- Raad Chegel
Applied Physics A (2023)
Ambipolar charge-transfer graphene plasmonic cavities
- Brian S. Y. Kim
- Aaron J. Sternbach
- D. N. Basov
Nature Materials (2023)
Surface plasmons induce topological transition in graphene/α-MoO3 heterostructures
- Francesco L. Ruta
Nature Communications (2022)
Synthesis, properties, and applications of MBenes (two-dimensional metal borides) as emerging 2D materials: a review
- Ashish Sharma
- V. S. Rangra
- Anupma Thakur
Journal of Materials Science (2022)
Quick links
- Explore articles by subject
- Guide to authors
- Editorial policies
Sign up for the Nature Briefing newsletter — what matters in science, free to your inbox daily.

2014 Theses Doctoral
Towards inducing superconductivity into graphene
Efetov, Dmitri K.
Graphenes transport properties have been extensively studied in the 10 years since its discovery in 2004, with ground-breaking experimental observations such as Klein tunneling, fractional quantum Hall effect and Hofstadters butterfly. Though, so far, it turned out to be rather poor on complex correlated electronic ground states and phase transitions, despite various theoretical predictions. The purpose of this thesis is to help understanding the underlying theoretical and experimental reasons for the lack of strong electronic interactions in graphene, and, employing graphenes high tunability and versatility, to identify and alter experimental parameters that could help to induce stronger correlations. In particular graphene holds one last, not yet experimentally discovered prediction, namely exhibiting intrinsic superconductivity. With its vanishingly small Fermi surface at the Dirac point, graphene is a semi-metal with very weak electronic interactions. Though, if it is doped into the metallic regime, where the size of the Fermi surface becomes comparable to the size of the Brillouin zone, the density of states becomes sizeable and electronic interactions are predicted to be dramatically enhanced, resulting in competing correlated ground states such as superconductivity, magnetism and charge density wave formation. Following these predictions, this thesis first describes the creation of metallic graphene at high carrier doping via electrostatic doping techniques based on electrolytic gates. Due to graphenes surface only properties, we are able to induce carrier densities above n>10¹⁴cm⁻²(εF>1eV) into the chemically inert graphene. While at these record high carrier densities we yet do not observe superconductivity, we do observe fundamentally altered transport properties as compared to semi-metallic graphene. Here, detailed measurements of the low temperature resistivity reveal that the electron-phonon interactions are governed by a reduced, density dependent effective Debey temperature - the so-called Bloch-Grüneisen temperature ΘBG. We also probe the transport properties of the high energy sub-bands in bilayer graphene by electrolyte gating. Furthermore we demonstrate that electrolyte gates can be used to drive intercalation reactions in graphite and present an all optical study of the reaction kinetics during the creation of the graphene derived graphite intercalation compound LiC₆, and show the general applicability of the electrolyte gates to other 2-dimensional materials such as thin films of complex oxides, where we demonstrate gating dependent conductance changes in the spin-orbit Mott insulator Sr₂IrO₄. Another, entirely different approach to induce superconducting correlations into graphene is by bringing it into proximity to a superconductor. Although not intrinsic to graphene, Cooper pairs can leak in from the superconductor and exist in graphene in the form of phase-coherent electron-hole states, the so-called Andreev states. Here we demonstrate a new way of fabricating highly transparent graphene/superconductor junctions by vertical stacking of graphene and the type-II van der Waals superconductor NbSe₂. Due to NbSe₂'s high upper critical field of Hc₂= 4 T we are able to test a long proposed and yet not well understood regime, where proximity effect and quantum Hall effect coexist.
- Nanoscience
- Superconductivity
- Fermi surfaces
- Dirac equation
- Quantum Hall effect
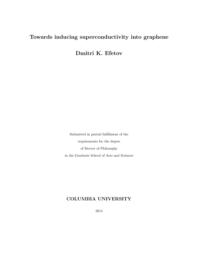

More About This Work
- DOI Copy DOI to clipboard
Alkali Metal Doped Graphene: Superconductivity, Structural, Magnetic and Optical Properties
- James Chapman
- Department of Physics & Astronomy
Student thesis : Phd
- Superconductivity
File : application/pdf, -1 bytes
Type : Thesis
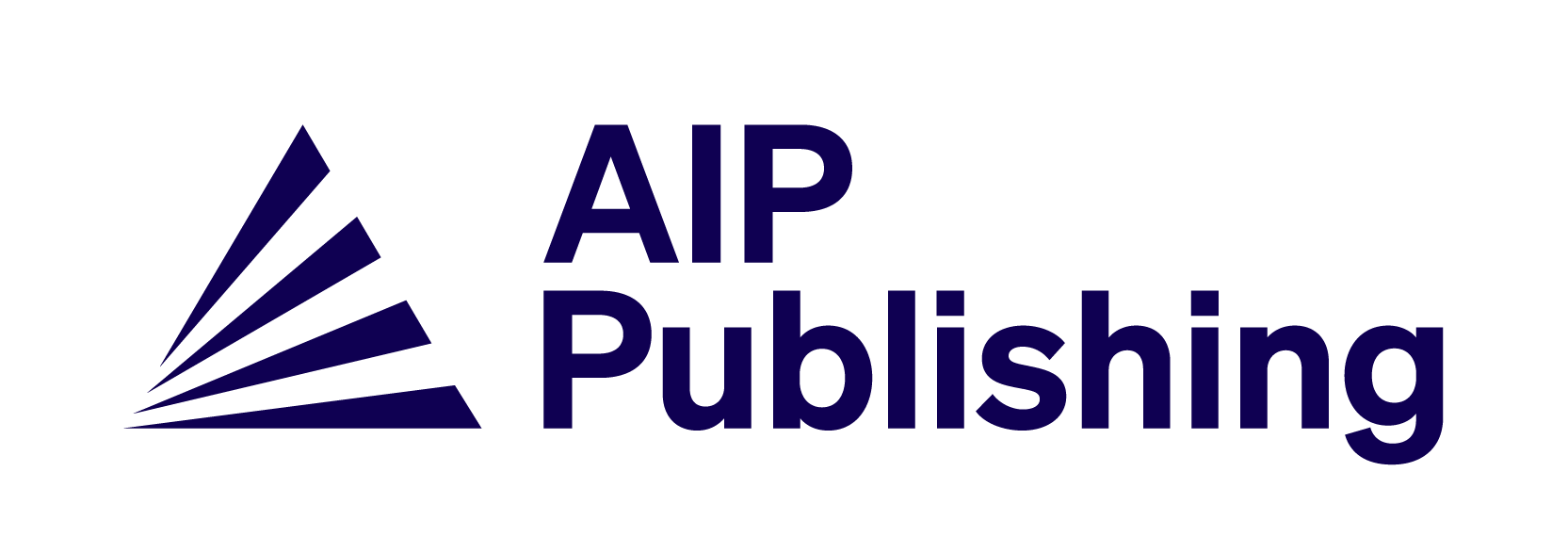
Surface transfer hole doping of epitaxial graphene using MoO 3 thin film
Author to whom correspondence should be addressed. Electronic mail: [email protected] .
- Article contents
- Figures & tables
- Supplementary Data
- Peer Review
- Reprints and Permissions
- Cite Icon Cite
- Search Site
Zhenyu Chen , Iman Santoso , Rui Wang , Lan Fei Xie , Hong Ying Mao , Han Huang , Yu Zhan Wang , Xing Yu Gao , Zhi Kuan Chen , Dongge Ma , Andrew Thye Shen Wee , Wei Chen; Surface transfer hole doping of epitaxial graphene using MoO 3 thin film. Appl. Phys. Lett. 24 May 2010; 96 (21): 213104. https://doi.org/10.1063/1.3441263
Download citation file:
- Ris (Zotero)
- Reference Manager
Synchrotron-based in situ photoelectron spectroscopy investigations demonstrate effective surface transfer p-type doping of epitaxial graphene (EG) thermally grown on 4H–SiC(0001) via the deposition of MoO 3 thin film on top. The large work function difference between EG and MoO 3 facilitates electron transfer from EG to the MoO 3 thin film. This leads to hole accumulation in the EG layer with an areal hole density of about 1.0 × 10 13 cm − 2 , and places the Fermi level 0.38 eV below the graphene Dirac point.
Sign in via your Institution
Citing articles via, submit your article.
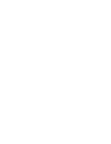
Sign up for alerts
- Online ISSN 1077-3118
- Print ISSN 0003-6951
- For Researchers
- For Librarians
- For Advertisers
- Our Publishing Partners
- Physics Today
- Conference Proceedings
- Special Topics
pubs.aip.org
- Privacy Policy
- Terms of Use
Connect with AIP Publishing
This feature is available to subscribers only.
Sign In or Create an Account
Nitrogen-Doped Graphene Quantum Dots for Efficient Detection of Toxic Gas
- Topical Collection: Low-Energy Digital Devices and Computing 2023
- Published: 07 May 2024
Cite this article
- Modhurima Bhuiya 1 ,
- Saurav Kumar 1 ,
- Aman Kumar 1 &
- Neha Agnihotri ORCID: orcid.org/0000-0003-0500-2905 1
Owing to their adjustable optoelectronic and adsorption characteristics, graphene quantum dots (GQDs) have become a potential material to manufacture effective gas sensors. Our present work deals with the study of interaction of a pristine graphene quantum dot (GQD) and a pyridinic nitrogen-doped defective graphene quantum dot (4N-GQD) with various toxic gases such as sulfur dioxide (SO 2 ), hydrogen fluoride (HF), hydrogen chloride (HCl), methane (CH 4 ), and carbon monoxide (CO). The 4N-GQD system has revealed strong interaction with these toxic gases in comparison to their pristine counterpart, which is because of the formation of an active region due to charge accumulation at the nitrogen-doped site. The adsorbate–adsorbent interaction in these systems has been studied at the molecular level employing density functional theory (DFT). Our findings suggest that the 4N-GQD system would be an effective adsorbent for a variety of toxic gases. These findings will help to steer the development of gas sensors with desirable features.
This is a preview of subscription content, log in via an institution to check access.
Access this article
Price includes VAT (Russian Federation)
Instant access to the full article PDF.
Rent this article via DeepDyve
Institutional subscriptions
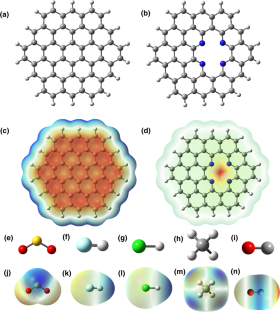
I. Manisalidis, E. Stavropoulou, A. Stavropoulos, and E. Bezirtzoglou, Environmental and health impacts of air pollution: a review. Front. Public Heal. 8, 1 (2020).
Google Scholar
J. Rentschler and N. Leonova, Global air pollution exposure and poverty. Nat. Commun. 14, 1 (2023).
Article Google Scholar
A.K. Jorgenson, Global warming and the neglected greenhouse gas: a cross-national study of the social causes of methane emissions intensity, 1995. Soc. Forces 84, 1779 (2006).
K.A. Mar, C. Unger, L. Walderdorff, and T. Butler, Beyond CO 2 equivalence: the impacts of methane on climate, ecosystems, and health. Environ. Sci. Policy 134, 127 (2022).
Article CAS Google Scholar
K. Sobieraj, S. Stegenta-Dąbrowska, G. Luo, J.A. Koziel, and A. Białowiec, Carbon monoxide fate in the environment as an inspiration for biorefinery industry: a review. Front. Environ. Sci. 10, 1 (2022).
J.-Y. Na, K.-H. Woo, S.-Y. Yoon, S.-Y. Cho, I.-U. Song, J.-A. Kim, and J.-S. Kim, Acute symptoms after a community hydrogen fluoride spill. Ann. Occup. Environ. Med. 25, 17 (2013).
Article PubMed PubMed Central Google Scholar
F.Z. Cui, J.J. Xie, S.Y. Jiang, S.X. Gan, D.L. Ma, R.R. Liang, G.F. Jiang, and X. Zhao, A gaseous hydrogen chloride chemosensor based on a 2D covalent organic framework. Chem. Commun. 55, 4550 (2019).
T.M. Chen, J. Gokhale, S. Shofer, and W.G. Kuschner, Outdoor air pollution: nitrogen dioxide, sulfur dioxide, and carbon monoxide health effects. Am. J. Med. Sci. 333, 249 (2007).
Article PubMed Google Scholar
D.A. Lashof and D.R. Ahuja, Relative contributions of greenhouse gas emissions to global warming. Nat. 344, 529 (1990).
C.S. Snyder, T.W. Bruulsema, T.L. Jensen, and P.E. Fixen, Review of greenhouse gas emissions from crop production systems and fertilizer management effects. Agric. Ecosyst. Environ. 133, 247 (2009).
X. Liu, S. Cheng, H. Liu, S. Hu, D. Zhang, and H. Ning, A survey on gas sensing technology. Sensors (Switzerland) 12, 9635 (2012).
T. Seiyama, K. Fujiishi, M. Nagatani, and A. Kato, A new detector for gaseous components using zinc oxide thin films. J. Soc. Chem. Ind. Japan 66, 652 (1963).
CAS Google Scholar
J.R. Li, Y. Tao, Q. Yu, X.H. Bu, H. Sakamoto, and S. Kitagawa, Selective gas adsorption and unique structural topology of a highly stable guest-free zeolite-type MOF material with n-rich chiral open channels. Chem. A Eur. J. 14, 2771 (2008).
E. Barea, C. Montoro, and J.A.R. Navarro, Toxic gas removal-metal-organic frameworks for the capture and degradation of toxic gases and vapours. Chem. Soc. Rev. 43, 5419 (2014).
Article CAS PubMed Google Scholar
Q. Li, Y. Li, and W. Zeng, Preparation and application of 2D MXene-based gas sensors: a review. Chemosensors 9, 225 (2021).
A. Bandyopadhyay, D. Ghosh, and S.K. Pati, Shining light on new-generation two-dimensional materials from a computational viewpoint. J. Phys. Chem. Lett. 9, 1605 (2018).
S.D. Lawaniya, S. Kumar, Y. Yu, H.G. Rubahn, Y.K. Mishra, and K. Awasthi, Functional nanomaterials in flexible gas sensors: recent progress and future prospects. Mater. Today Chem. 29, 101428 (2023).
R. Deji, A. Verma, N. Kaur, B.C. Choudhary, and R.K. Sharma, Density functional theory study of carbon monoxide adsorption on transition metal doped armchair graphene nanoribbon. Mater. Today Proc. 54, 771 (2022).
M. Bacon, S.J. Bradley, and T. Nann, Graphene quantum dots. Part. Part. Syst. Charact. 31, 415 (2014).
K.S. Novoselov, A.K. Geim, S.V. Morozov, D. Jiang, M.I. Katsnelson, I.V. Grigorieva, S.V. Dubonos, and A.A. Firsov, Two-dimensional gas of massless dirac fermions in graphene. Nature 438, 197 (2005).
P. Tian, L. Tang, K.S. Teng, and S.P. Lau, Graphene quantum dots from chemistry to applications. Mater. Today Chem. 10, 221 (2018).
W. Chen, G. Lv, W. Hu, D. Li, S. Chen, and Z. Dai, Synthesis and applications of graphene quantum dots: a review. Nanotechnol. Rev. 7, 157 (2018).
M. Li, W. Ni, B. Kan, X. Wan, L. Zhang, Q. Zhang, G. Long, Y. Zuo, and Y. Chen, Graphene quantum dots as the hole transport layer material for high-performance organic solar cells. Phys. Chem. Chem. Phys. 15, 18973 (2013).
F. Schedin, A.K. Geim, S.V. Morozov, E.W. Hill, P. Blake, M.I. Katsnelson, and K.S. Novoselov, Detection of individual gas molecules adsorbed on graphene. Nat. Mater. 6, 652 (2007).
I.K. Petrushenko, N.A. Ivanov, and K.B. Petrushenko, Theoretical investigation of carbon dioxide adsorption on Li+-decorated nanoflakes. Molecules 26, 1 (2021).
R. Krishnakumar, A. James, and R.S. Swathi, Metal-decorated crown ether-embedded graphene nanomeshes for enhanced molecular adsorption. Adv. Theory Simulat. 4, 1 (2021).
N. Promthong, C. Tabtimsai, W. Rakrai, and B. Wanno, Transition metal-doped graphene nanoflakes for CO and CO 2 Storage and sensing applications: a DFT study. Struct. Chem. 31, 2237 (2020).
K. Ghosh, N.K. Mridha, A.A. Khan, N. Baildya, T. Dutta, K. Biswas, and N.N. Ghosh, CO 2 activation on transition metal decorated graphene quantum dots: an insight from first principles. Phys. E Low-Dimensional Syst. Nanostructures 135, 114993 (2022).
Y.H. Zhang, Y. Bin Chen, K.G. Zhou, C.H. Liu, J. Zeng, H.L. Zhang, and Y. Peng, Improving gas sensing properties of graphene by introducing dopants and defects: a first-principles study. Nanotechnology 20, 185504 (2009).
E.C. Agwamba, H. Louis, P.O. Olagoke, T.E. Gber, G.A. Okon, C.F. Fidelis, and A.S. Adeyinka, Modeling of magnesium-decorated graphene quantum dot nanostructure for trapping AsH3, PH3 and NH3 gases. RSC Adv. 13, 13624 (2023).
Article CAS PubMed PubMed Central Google Scholar
S. Motahari, F. Shayeganfar, and M. Neek-Amal, Van der waals energy surface of a carbon nanotube sheet. Solid State Commun. 152, 225 (2012).
B.E. Inah, J.F. Eze, H. Louis, H.O. Edet, T.E. Gber, E.A. Eno, A.N. Etim, and A.S. Adeyinka, Adsorption and gas-sensing investigation of oil dissolved gases onto nitrogen and sulfur doped graphene quantum dots. Chem. Phys. Impact 7, 100265 (2023).
H.R. Balangi and A.A. Shokri, An Ab-initio study of adsorption of gaseous molecules on doped graphene structures. Phys. E Low-Dimensional Syst. Nanostructures 74, 515 (2015).
D.E. Jiang, V.R. Cooper, and S. Dai, Porous graphene as the ultimate membrane for gas separation. Nano Lett. 9, 4019 (2009).
E. Velez-Fort, C. Mathieu, E. Pallecchi, M. Pigneur, M.G. Silly, R. Belkhou, M. Marangolo, A. Shukla, F. Sirotti, and A. Ouerghi, Epitaxial graphene on 4H-SiC(0001) grown under nitrogen flux: evidence of low nitrogen doping and high charge transfer. ACS Nano 6, 10893 (2012).
O. Leenaerts, B. Partoens, and F.M. Peeters, Adsorption of H 2 O, NH 3 , CO, NO 2 , and NO on graphene: a first-principles study. Phys. Rev. B Condens. Matter. Mater. Phys. 77, 1 (2008).
D. Ghosh, and S.K. Pati, Trapping of gaseous pollutants on defective N-doped graphene. Phys. Chem. Chem. Phys. 19, 636 (2017).
U. Nath and M. Sarma, Pyridinic dominance n-doped graphene: a potential material for SO 2 gas detection. J. Phys. Chem. A 127, 1112 (2023).
P. Lazar, R. Mach, and M. Otyepka, Spectroscopic fingerprints of graphitic, pyrrolic, pyridinic, and chemisorbed nitrogen in n-doped graphene. J. Phys. Chem. C 123, 10695 (2019).
D. Guo, R. Shibuya, C. Akiba, S. Saji, T. Kondo, and J. Nakamura, Active sites of nitrogen-doped carbon materials for oxygen reduction reaction clarified using model catalysts. Science 351, 361 (2016).
Z. Luo, S. Lim, Z. Tian, J. Shang, L. Lai, B. MacDonald, C. Fu, Z. Shen, T. Yu, and J. Lin, Pyridinic N doped graphene: synthesis, electronic structure, and electrocatalytic property. J. Mater. Chem. 21, 8038 (2011).
L. Tang, R. Ji, X. Li, K.S. Teng, and S.P. Lau, Energy-level structure of nitrogen-doped graphene quantum dots. J. Mater. Chem. C 1, 4908 (2013).
M.J. Frisch Gaussian 16, Revision C.01, M.J. Frisch, G.W. Trucks, H.B. Schlegel, G.E. Scuseria, M.A. Robb, J.R. Cheeseman, G. Scalmani, V. Barone, G.A. Petersson, H. Nakatsuji, X. Li, M. Caricato, A.V. Marenich, J. Bloino, B.G. Janesko, R. Gomperts, B. Mennucci, H.P. Hratchian, J.V. Ortiz, A.F. Izmaylov, J.L. Sonnenberg, D. Williams-Young, F. Ding, F. Lipparini, F. Egidi, J. Goings, B. Peng, A. Petrone, T. Henderson, D. Ranasinghe, V.G. Zakrzewski, J. Gao, N. Rega, G. Zheng, W. Liang, M. Hada, M. Ehara, K. Toyota, R. Fukuda, J. Hasegawa, M. Ishida, T. Nakajima, Y. Honda, O. Kitao, H. Nakai, T. Vreven, K. Throssell, J. A. Montgomery, Jr., J. E. Peralta, F. Ogliaro, M. J. Bearpark, J.J. Heyd, E. N. Brothers, K.N. Kudin, V.N. Staroverov, T. A. Keith, R. Kobayashi, J. Normand, K. Raghavachari, A.P. Rendell, J.C. Burant, S.S. Iyengar, J. Tomasi, M. Cossi, J.M. Millam, M. Klene, C. Adamo, R. Cammi, J.W. Ochterski, R.L. Martin, K. Morokuma, O. Farkas, J.B. Foresman, and D.J. Fox, Gaussian, Inc., Wallingford CT, 2016, Citation|Gaussian.Com , https://gaussian.com/citation/ .
J. Da Chai and M. Head-Gordon, Long-range corrected hybrid density functionals with damped atom-atom dispersion corrections. Phys. Chem. Chem. Phys. 10, 6615 (2008).
D. Ghosh, G. Periyasamy, and S.K. Pati, Adsorption of HF pollutant on single vacant 2D nanosheets: ab initio molecular dynamics study. J. Phys. Chem. C 117, 21700 (2013).
T. Koopmans, Über die zuordnung von wellenfunktionen und eigenwerten zu den einzelnen elektronen eines atoms. Physica 1, 104 (1934).
E.R. Johnson, S. Keinan, P. Mori-Sánchez, J. Contreras-García, A.J. Cohen, and W. Yang, Revealing noncovalent interactions. J. Am. Chem. Soc. 132, 6498 (2010).
T. Lu, and F. Chen, Multiwfn: a multifunctional wavefunction analyzer. J. Comput. Chem. 33, 580 (2012).
H.L. Williams, T. Kelley, C. Bersch, H.B. Bröker, J. Campbell, R. Cunningham, D. Denholm, G. Elber, R. Fearick, C. Grammes, Gnuplot 5.2. An interactive plotting program. Available online: http://www.gnuplot.info/docs_5 . 2 (2017)
W. Humphrey, A. Dalke, and K. Schulten, VMD: visual molecular dynamics. J. Mol. Graph. 14, 33 (1996).
Y. Wang, Y. Shao, D.W. Matson, J. Li, and Y. Lin, Nitrogen-doped graphene and its application in electrochemical biosensing. ACS Nano 4, 1790 (2010).
H.R. Ghenaatian, M. Shakourian-Fard, M.R. Moghadam, G. Kamath, and M. Rahmanian, Tailoring of graphene quantum dots for toxic heavy metals detection. Appl. Phys. A Mater. Sci. Process. 125, 1 (2019).
P. Recum and T. Hirsch, Graphene-based chemiresistive gas sensors. Nanoscale Adv. 6, 11 (2023).
M.M. Francl, W.J. Pietro, W.J. Hehre, J.S. Binkley, M.S. Gordon, D.J. DeFrees, and J.A. Pople, Self-consistent molecular orbital methods XXIII. A polarization-type basis set for second-row elements. J. Chem. Phys. 77, 3654 (1982).
D.R. Roy, M.A. Zaeem, S. Thomas, and V. Kumar, Two-dimensional boron−phosphorus monolayer for reversible NO 2 gas sensing. ACS Appl. Nano Mater. 3, 10073 (2020).
C.M. Masemola, N. Moloto, Z.N. Tetana, S.S. Gqoba, P.K. Mubiayi, and E.C. Linganiso, N-doped graphene quantum dot-modified polyaniline for room-temperature sensing of alcohol vapors. Mater. Chem. Phys. 287, 126229 (2022).
V.S. Anithaa, R. Shankar, and S. Vijayakumar, DFT-based investigation on adsorption of methane on pristine and defected graphene. Struct. Chem. 28, 1935 (2017).
Download references
Acknowledgments
The authors would like to express their gratitude to the Science and Engineering Research Board (SERB), New Delhi, India for providing the financial support.
Author information
Authors and affiliations.
Department of Physics, National Institute of Technology, Jamshedpur, 831014, India
Modhurima Bhuiya, Saurav Kumar, Aman Kumar & Neha Agnihotri
You can also search for this author in PubMed Google Scholar
Contributions
MB: Data analysis, Visualization, Writing-original draft. SK: Investigation, Validation. AK: Visualization. NA: Conceptualization, Supervision, Writing-review & editing, Funding.
Corresponding author
Correspondence to Neha Agnihotri .
Ethics declarations
Conflict of interest.
On behalf of all authors, the corresponding author states that there is no conflict of interest.
Ethical Approval
Not Applicable.
Additional information
Publisher's note.
Springer Nature remains neutral with regard to jurisdictional claims in published maps and institutional affiliations.
Rights and permissions
Springer Nature or its licensor (e.g. a society or other partner) holds exclusive rights to this article under a publishing agreement with the author(s) or other rightsholder(s); author self-archiving of the accepted manuscript version of this article is solely governed by the terms of such publishing agreement and applicable law.
Reprints and permissions
About this article
Bhuiya, M., Kumar, S., Kumar, A. et al. Nitrogen-Doped Graphene Quantum Dots for Efficient Detection of Toxic Gas. J. Electron. Mater. (2024). https://doi.org/10.1007/s11664-024-11123-8
Download citation
Received : 30 September 2023
Accepted : 15 April 2024
Published : 07 May 2024
DOI : https://doi.org/10.1007/s11664-024-11123-8
Share this article
Anyone you share the following link with will be able to read this content:
Sorry, a shareable link is not currently available for this article.
Provided by the Springer Nature SharedIt content-sharing initiative
- Nitrogen-doped graphene quantum dots
- toxic gases
- density functional theory
Advertisement
- Find a journal
- Publish with us
- Track your research

Green Chemistry
Boosting the catalytic performance of ru nanoparticles in the cleavage of β-o-4 linkages in lignin by doping mo †.

* Corresponding authors
a Department of Electronic Engineering, School of Electronic Information and Electrical Engineering, Shanghai Jiao Tong University, Shanghai 200240, P. R. China E-mail: [email protected]
In this work, Ru nanoparticles doped with Mo on reduced graphene oxide (RuMo/rGO) are prepared through synchronous reduction of RuCl 3 , MoCl 5 , and graphene oxide. The compositions, morphologies, and structures of the as-prepared RuMo/rGO composites are fully characterized. Their catalytic properties in oxidative cleavage of β-O-4 linkages in lignin model compounds and lignin are explored. It is illustrated that with O 2 as the oxidant RuMo/rGO shows overall better catalytic performance in the oxidative cleavage of β-O-4 linkages than the catalysts, such as Ru/rGO and RuCo/rGO, reported in our previous work. The catalytic mechanism is elaborated through experiments and density functional theory (DFT) calculation. Pronouncedly, we show that the as-doped Mo can effectively boost the molecular oxygen activation process and the adsorption of β-O-4 model compounds on the Ru nanoparticle surface on rGO, and thus enhance the catalytic activity. It is demonstrated meanwhile that RuMo/rGO can catalyze the depolymerization of organosolv birch lignin yielding 17.1% aromatic compounds under relatively mild conditions (100 °C, 0.3 MPa O 2 , 12 h, pH = 12).
Supplementary files
- Supplementary information PDF (1796K)
Article information
Download citation, permissions.

Boosting the catalytic performance of Ru nanoparticles in the cleavage of β-O-4 linkages in lignin by doping Mo
P. Lei, J. Zhang, W. Shen, M. Zhong and S. Guo, Green Chem. , 2024, Advance Article , DOI: 10.1039/D4GC00294F
To request permission to reproduce material from this article, please go to the Copyright Clearance Center request page .
If you are an author contributing to an RSC publication, you do not need to request permission provided correct acknowledgement is given.
If you are the author of this article, you do not need to request permission to reproduce figures and diagrams provided correct acknowledgement is given. If you want to reproduce the whole article in a third-party publication (excluding your thesis/dissertation for which permission is not required) please go to the Copyright Clearance Center request page .
Read more about how to correctly acknowledge RSC content .
Social activity
Search articles by author.
This article has not yet been cited.
Advertisements

IMAGES
VIDEO
COMMENTS
We first determine the type of carrier induced in our TOS-doped monolayer graphene using Raman spectroscopy, as shown in Fig. 1c.We find a clear blueshift in both G and 2D peaks (18.1 and 4.3 cm ...
Figure 4 Gated electrical testing of a graphene device. The devices in this thesis are exfoliated onto 300 nm of SiO2, which functions as a gate dielectric. ... Figure 15 Demonstration of tunable complimentary doping of graphene via HSQ films on the basal plane. The cross-linking in the HSQ is controlled through the incident irradiation ...
This article presents a systematic, critical, and more informative review of the recent advancement in the chemical doping of graphene and its applications. Starting with a brief history along with the properties and synthesis of graphene, different experimental approaches to chemical doping and their outcomes reported so far are systematically ...
Recently, a lot of effort has been focused on improving the performance and exploring the electric properties of graphene.This article presents a summary of chemical doping of graphene aimed at tuning the electronic properties of graphene. p-Type and n-type doping of graphene achieved through surface transfer doping or substitutional doping and their applications based on doping are reviewed.
With this method, Kim et al. [28] determined charge carrier concentration in single layer graphene doped by an organic molecule exhibiting a large dipole moment.The dipolar molecule highly p-dopes graphene, resulting in upshifts of both Raman G and 2D bands. By further careful quantitative analyses with the position of G band and electrical measurements, they showed that charge carrier ...
a highly sensitive all-graphene solution-processed thermocouple. Laminates of large aspect ratio bilayer graphene nanosheets . All laminates discussed in this report were prepared by blade coating of a Polyethylene terephthalate (PET) film with an additive-free graphene slurry with a solid content of around 50 g·L-1 in N-Methyl-2-pyrrolidone ...
Graphene, a new material for the electron-device community, has many extraordinary properties. Especially, it provides a perfect platform to explore the unique electronic property in absolutely two-dimensions. However, most electronic applications are handicapped by the absence of a semiconducting gap in pristine graphene. To control the semiconducting properties of graphene, doping is ...
Atmospheric doping effects in epitaxial graphene: correlation of local and global electrical measurements Vishal Panchal1, Cristina E. Giusca1, Arseniy Lartsev2, Nicholas A. Martin1, Nathan Cassidy1,3, Rachael L. Myers-Ward4, D. Kurt Gaskill4, and Olga Kazakova1* 1National Physical Laboratory, Teddington, TW11 0LW, UK 2Chalmers University of Technology, Gothenburg, S-412 96, Sweden
Graphene based materials can be effectively modified by doping in order to specifically tailor their properties toward specific applications. So far the most used and widely investigated dopant heteroatom is probably nitrogen. However, boron is also an equally important element that can induce novel and comp 2016 Journal of Materials Chemistry A HOT Papers 2016 Journal of Materials Chemistry A ...
Due to its ultrabroadband optical absorption, large theoretical surface area, outstanding thermal conductivity and massless electron transportation, graphene has been considered as a prospective candidate in the applications of energy conversion and storage. However, the gapless energy band structure and the Journal of Materials Chemistry A Recent Review Articles
1 Uniform doping of graphene close to the charge neutrality point by polymer-assisted spontaneous assembly of molecular dopants Hans He1, Kyung Ho Kim1,2, Andrey Danilov1, Domenico Montemurro1, Liyang Yu3, Yung Woo Park2,4,5, Floriana Lombardi1, Thilo Bauch1, Kasper Moth-Poulsen3, Tihomir Iakimov6, Rositsa Yakimova6, Per Malmberg3, Christian Müller3, Sergey Kubatkin1 & Samuel Lara-Avila1,7
Following these predictions, this thesis first describes the creation of metallic graphene at high carrier doping via electrostatic doping techniques based on electrolytic gates. Due to graphenes surface only properties, we are able to induce carrier densities above n>10¹⁴cm⁻²(εF>1eV) into the chemically inert graphene. ...
When graphene is electron-doped, the electrons occupy the conduction-band bottom and increase the Fermi energy (as shown in Figure 1 b). The Fermi energy is proportional to the square-root of charge density (E F = ℏ v F π n).This relationship can be derived from the DOS formula in Figure 1 b [5].Furthermore, negative charge lowers the electrostatic potential (φ) of graphene, which ...
to tune the graphene doping. However, the method is limited by the charge that can be intro-duced in the material. This limitation depends on the dielectric constant of the layer that needs to be deposited: on top of the graphene (when the substrate is glass, "top-gating"), or below the graphene (when the substrate is Si, "back-gating").
Figure 3. Raman ( a) and XRD ( b) patterns of undoped graphene aerogels prepared with dilution 20, and nickel−doped graphene aerogels with 5% wt. of nickel. The structure of the graphene aerogels was further analyzed by X-ray diffraction, and the XRD patterns are shown in Figure 3 b. The XRD pattern of the graphene aerogel (GA-20) shows two ...
Three nitrogen-doped graphene samples were synthesized by the hydrothermal method using urea as doping/reducing agent for graphene oxide (GO), previously dispersed in water. The mixture was poured into an autoclave and placed in the oven at 160 °C for 3, 8 and 12 h. The samples were correspondingly denoted NGr-1, NGr-2 and NGr-3. The effect of the reaction time on the morphology, structure ...
This thesis consist of 8 sections. The second section gives the necessary theoretical background and discusses graphene electronic and optical properties, photodetection ... where graphene doping theoretically is zero. In real graphene devices the situation of zero doping can not be observed due to impurities and disorder. Residual doping also
Nitrogen-doped graphene can be synthesized at lower temperatures under hydrothermal or solvothermal conditions. Bao and co-workers [21] synthesized nitrogen-doped graphene containing 13 at.% by reacting tertachloromethane with lithium nitride. Long et al. [22] were able to dope up to 5 at.% nitrogen by reduction of GO with hydrazine hydrate and ammonia while the wet chemical reaction between ...
Ca-doped graphene paper exhibited a 20% lower carrier concentration than Ca-intercalated graphite, offering an explanation for the lower value of TC. To allow insight into the partial doping of graphene papers, samples were exposed to air and monitored via dynamic x-ray diffraction techniques and optical analysis during degradation.
The chemical doping of foreign atoms and functional moieties is a significant strategy for tailoring the electronic properties and enhancing the catalytic ability of graphene. However, the general approaches to the synthesis of heteroatom-doped graphene often involve chemical vapor deposition (CVD) and/or th
Graphene is a carbon nanomaterial made of two-dimensional layers of a single atom thick planar sheet of sp 2-bonded carbon atoms packed tightly in a honeycomb lattice crystal [13], [17].Graphene's structure is similar to lots of benzene rings jointed where hydrogen atoms are replaced by the carbon atoms Fig. 1 a and is considered as hydrophobic because of the absence of oxygen groups [10].
Synchrotron-based in situ photoelectron spectroscopy investigations demonstrate effective surface transfer p-type doping of epitaxial graphene (EG) thermally grown on 4H-SiC(0001) via the deposition of MoO 3 thin film on top. The large work function difference between EG and MoO 3 facilitates electron transfer from EG to the MoO 3 thin film. This leads to hole accumulation in the EG layer ...
Owing to their adjustable optoelectronic and adsorption characteristics, graphene quantum dots (GQDs) have become a potential material to manufacture effective gas sensors. Our present work deals with the study of interaction of a pristine graphene quantum dot (GQD) and a pyridinic nitrogen-doped defective graphene quantum dot (4N-GQD) with various toxic gases such as sulfur dioxide (SO2 ...
FPSs based on GR/KNN/P(VDF-TrFE) three-phase composites are fabricated via doping a mass fraction of 15 wt% potassium sodium niobate (KNN) ceramic powder and various contents of graphene (GR) nanosheets into a P(VDF-TrFE) matrix. We find that an appropriate amount of GR is responsible for the enhanced crystallinity and β-phase of P(VDF-TrFE).
In this work, Ru nanoparticles doped with Mo on reduced graphene oxide (RuMo/rGO) are prepared through synchronous reduction of RuCl3, MoCl5, and graphene oxide. The compositions, morphologies, and structures of the as-prepared RuMo/rGO composites are fully characterized. Their catalytic properties in oxidative cle