- Open access
- Published: 28 March 2022

Priorities for research on environment, climate and health, a European perspective
- Elina Drakvik 1 , 2 ,
- Manolis Kogevinas ORCID: orcid.org/0000-0002-9605-0461 3 , 4 , 5 , 6 ,
- Åke Bergman 1 ,
- Anais Devouge 7 &
- Robert Barouki 7
on behalf of the HERA (Health and Environment Research Agenda) Consortium
Environmental Health volume 21 , Article number: 37 ( 2022 ) Cite this article
7254 Accesses
6 Citations
1 Altmetric
Metrics details
Climate change, urbanisation, chemical pollution and disruption of ecosystems, including biodiversity loss, affect our health and wellbeing. Research is crucial to be able to respond to the current and future challenges that are often complex and interconnected by nature. The HERA Agenda, summarised in this commentary, identifies six thematic research goals in the environment, climate and health fields. These include research to 1) reduce the effects of climate change and biodiversity loss on health and environment, 2) promote healthy lives in cities and communities, 3) eliminate harmful chemical exposures, 4) improve health impact assessment and implementation research, 5) develop infrastructures, technologies and human resources and 6) promote research on transformational change towards sustainability. Numerous specific recommendations for research topics, i.e., specific research goals, are presented under each major research goal. Several methods were used to define the priorities, including web-based surveys targeting researchers and stakeholder groups as well as a series of online and face-to-face workshops, involving hundreds of researchers and other stakeholders. The results call for an unprecedented effort to support a better understanding of the causes, interlinkages and impacts of environmental stressors on health and the environment. This will require breakdown of silos within policies, research, actors as well as in our institutional arrangements in order to enable more holistic approaches and solutions to emerge. The HERA project has developed a unique and exciting opportunity in Europe to consensuate priorities in research and strengthen research that has direct societal impact.
Peer Review reports
Climate change, urbanisation, chemical pollution and disruption of ecosystems, including biodiversity loss, impact our health and quality of life. Research is instrumental to be able to respond to the current and future environmental and health challenges that are so complex and interlinked by nature. The European Commission (EC), in line with policies of the European Union and the United Nations Sustainable Development Goals [ 1 ], launched a call for proposals to define priorities for research on environment, climate and health [ 2 ]. The Health and Environment Research Agenda (HERA) project, emerging from that call, was developed by a European consortium, and recently submitted its final report entitled “EU research agenda for the environment, climate & health, 2021–2030” [ 3 ], summarised in this commentary. The HERA Agenda highlights several key areas where further research is crucial for the next decade. This article provides a topical contribution to discussion of environmental health priorities and provides opportunities to reflect on future directions of research in this field, especially in the European context.
Process for developing the European research agenda
The approach that the project followed was based on principles of transparency, inclusiveness and mutual learning [ 4 ]. During the course of the project, the HERA Consortium performed extensive reviews of current knowledge, policies and research activities (Fig. 1 ). Web-based surveys targeting research communities and other stakeholder groups were carried out, along with online and face-to-face workshops, which taken together, involved hundreds of participants. Researchers primarily identified major current areas of concern, i.e. air pollution, chemicals, climate, cities, as priorities for research. Other stakeholders mostly identified implementation science and global issues (e.g. climate change, biodiversity loss) as priorities. The stakeholder process and results are described in detail in Paloniemi et al. [ 5 ]. Responses from the surveys and workshops were discussed by the HERA working group that further identified “Gaps in gaps”, namely research areas that are not well developed but that were not identified by the researchers’ survey. Research goals were prioritised by the HERA working group using the following criteria (modified from [ 6 ]): Novelty; Importance to People, Importance to the environment on a planetary scale; Impact on Policy; and, Innovation and Sustainable Development. A consensus-based approach was used for agreeing and refining the research goals along the process, based on the input and expertise of the HERA Consortium members, editorial group and independent scientific advisory board as well as input received through a public consultation.
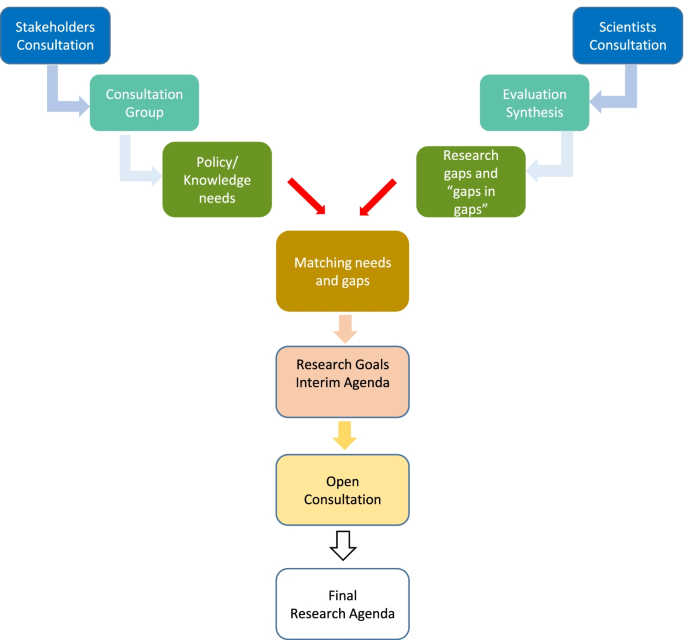
HERA framework for engaging stakeholders and scientist in the definition of Research goals (RG) for the research agenda
The EU research agenda for the environment, climate & health, 2021–2030
The EU Research Agenda developed by the HERA project covers six major research goals on environment, climate and health. Within each of them, research areas were identified and research needs specified resulting in altogether 30 specific research goals (Table 1 ). Several of the research goals are interlinked e.g. air-pollution is identified as a priority in the global environment (Research Goal 1.6 Global pollution) and the local environment, cities and communities (Research Goal 2.2 Air pollutants in indoor and outdoor environments). Moreover, the Research Agenda addresses research that can contribute to relevant policy objectives promoting health and the environment, especially in the context of the European Green Deal [ 7 ]. The Green Deal aims at achieving climate neutrality, biodiversity preservation, a circular economy and a zero pollution/toxic-free ambition as well as providing a way forward for achieving sustainable food system. The HERA agenda and the identified research needs can hence strengthen the knowledge and evidence-base in these cross-cutting policy areas, directly supporting the implementation of the Green Deal.
The six overarching Research Goals
Research goal 1 “Climate change and biodiversity loss – reduce effects on health and the environment” focuses on global interconnected issues. The consequences of climate change, biodiversity loss, disruption of food chains, emerging infectious diseases and decreased ecosystem services on health are not well understood despite evidence that they have major and persistent effects on life and the environment globally that became evident from the COVID-19 pandemic. Furthermore, more attention is required for addressing pollution, including air pollution, at a global scale. The need to promote research for effective policies on mitigation and adaptation is identified as of paramount importance, as well as investigating co-benefits with air pollution mitigation policies. Overall, the research goal highlights the need for holistic approaches such as One Health and Planetary Health.
Research goal 2 “Cities and communities – promote healthy lives in sustainable and inclusive societies” focuses on problem-based research. Living conditions in urban environments are of key concern as they impact the health and wellbeing of most European citizens. The impacts of environmental factors (e.g. air pollution, noise, digitalisation), may vary in different contexts such as the urban environment workplace or contaminated land. Research should examine the complex relationships in these environments, and evaluate and promote positive interventions.
Research goal 3 “ Chemicals and physical stressors – prevent and eliminate harmful chemical exposures to health” focuses on chemicals, other stressors and environmental media. There are still many unknowns on the hazards and risks related to stressor families including chemicals and mixtures, physical stressors such as radiation (ranging from ionising to light exposure), and the role played by the various environmental media carrying these stressors such as water. Research should cover the tens of thousands of chemicals in daily usage that we have very little health information on and interactions of environmental exposures with other factors such as genes, occupation, political and socioeconomic determinants of health, a theme covered also in RG6 on interdisciplinary research. Regulatory decisions rely heavily on additional knowledge in these specific areas. Research should effectively address the challenges of a zero pollution paradigm and a sustainable future.
Research goal 4 “Improve health impact assessment of environmental factors and promote implementation research” focuses on the need to develop new harmonized methodologies to evaluate the burden of environmental and climate change on health and to identify and assess the health benefits of human environmental interaction. Moreover, research should promote optimal ways to implement science-based decisions and policies as this is a limiting factor in many fields.
Research goal 5 “Develop infrastructures, technologies and human resources for sustainable research on environment, climate change and health” focuses on the need of European research infrastructures to be strengthened and further developed. Infrastructures provide a basis for excellent research. Key proposals are establishing harmonized coordination of ongoing large cohort studies including tens of millions of participants, exposome characterization, laboratory infrastructure, data analysis using the latest data science tools, new methods for exposure assessment (e.g. sensors) and planetary monitoring tools.
Research goal 6 “Promote research on transformational change in environment, climate change and health” focuses on the need of transformational change to address the intertwined environmental, social and health issues and reach critical global goals towards sustainability and equity. Societies will need to adapt to the challenges elicited by environmental stressors and climate change and this will require significant transformation of individual and collective behaviour and of policy making across the sectors and silos. Development of research approaches directed to finding and promoting workable solutions together is necessary for achieving such transformations.
Conclusions—a vision for future research
It is striking how the HERA surveys and stakeholder consultations pointed out such a large number of knowledge gaps, even in areas such as climate change where relevant evidence-based policies are urgently needed. The ambitious political goals set in the UN Agenda for Sustainable Development and the European Green Deal, will need major investments in research and innovation. The HERA Agenda coincides with the reports highlighting the planetary boundaries [ 8 , 9 ], and intertwined environmental pressures, the triple planetary crisis: climate change, biodiversity loss and pollution, affecting the health of the planet and of the people [ 10 ]. The Agenda reinforces the opportunity to bring together human health and environment field to work together on integrated and transformative solutions. The focus is on Europe, hence putting less emphasis on major exposures, such as indoor air pollution from biomass, that are much more prevalent in low- and middle-income countries. In fact, there is an urgent need to also develop a global Agenda since most of the problems and solutions discussed in HERA are not limited to Europe. In recent years, increases in the EU allocation to environment and health projects through the Framework Programme budgets and rise in the interest and importance of the field ([ 11 ], see page 65), have not yet managed to close the long-term gap that exists between required research and funding. It is a positive signal that the HERA Agenda has already been applied by the European Commission in recent calls for funding, as for example calls for the indoor environment, or planned calls on planetary health or the interlink of infections and the environment. Nevertheless, the vision for future research underlying this Agenda calls for an unprecedented effort to support a better understanding of the causes, interlinkages and impacts of environmental determinants on health. Integrated and holistic research should support policies and practices to protect and promote human health and well-being while simultaneously improving the critical state of the environment, including climate change mitigation and ecosystem restoration, in Europe and globally. This requires transformational change at societal level to break down the silos in policymaking, research, and institutional arrangements, enabling cross-sectoral, interdisciplinary and holistic approaches and solutions to emerge.
Availability of data and materials
Not applicable.
UN (2016): UN Agenda for Sustainable Development https://www.un.org/sustainabledevelopment/development-agenda/ . Accessed 25 Nov 2021.
EC (2018): Setting the priorities for a European environment, climate and health research agenda SC1-HCO-13–2018: https://ec.europa.eu/info/funding-tenders/opportunities/portal/screen/opportunities/topic-details/sc1-hco-13-2018 . Accessed 25 Nov 2021.
HERA Agenda (2021): https://www.heraresearcheu.eu/hera-2030-agenda ) Accessed 25 Nov 2021.
HERA Agenda Annex (2020): Interim document. https://static1.squarespace.com/static/5d6d2b4f677cfc00014c7b53/t/5e8da0050340080865cbbe8e/1586339855357/Annex+1.pdf . Accessed 25 Nov 2021.
Paloniemi R. et al. (2022): A transformative research approach addressing the interface between health, environment and climate: the state of European policy and practice (Submitted for review)
Kogevinas M. Setting priorities in environmental health research. Environ Epidemiol. 2017;1: e001. https://doi.org/10.1097/EE9.0000000000000001 .
Article Google Scholar
EC (2019): The European Green Deal. https://ec.europa.eu/info/strategy/priorities-2019-2024/european-green-deal_en . Accessed 25 Nov 2021.
Steffen W, Richardson K, Rockstrom J, Cornell SE, Fetzer I, Bennett EM, Biggs R, Carpenter SR, de Vries W, de Wit CA, Folke C, Gerten D, Heinke J, Mace GM, Persson LM, Ramanathan V, Reyers B, Sorlin S. Planetary Boundaries: Guiding Human Development on a Changing Planet. Science. 2015;347(6223):1259855–1259855. https://doi.org/10.1126/science.1259855 .
Article CAS Google Scholar
Persson L, Almroth C, Collins CD, Cornell S, de Wit C, et al. Outside the Safe Operating Space of the Planetary Boundary for Novel Entities. Environ Sci Technol. 2022. https://doi.org/10.1021/acs.est.1c04158 .
UNEP (2021): Making Peace with Nature: A scientific blueprint to tackle the climate, biodiversity and pollution emergencies. Nairobi. https://www.unep.org/resources/making-peace-nature . Accessed 11 Feb 2022.
Karjalainen T. (2021): European research on environment and health. Projects funded by Horizon 2020 (2014–2020). https://op.europa.eu/en/publication-detail/-/publication/03a2c022-9c01-11eb-b85c-01aa75ed71a1/language-en/format-PDF/source-199503195#document-info . Accessed 25 Nov 2021.
Download references
Acknowledgements
The HERA project was completed with the contributions of hundreds of researchers and stakeholders. HERA participants Robert Barouki (French National Institute of Health and Medical Research-INSERM), Manolis Kogevinas (Barcelona Institute for Global Health -ISGlobal), Åke Bergman (Stockholm University-SU), Elina Drakvik (SU) and Anaïs Devouge (INSERM)—drafted the HERA Agenda, 2021, with extensive contributions from Denis Sarigiannis (Aristotle University of Thessaloniki); Delphine Destoumieux-Garzón (The National Center for Scientific Research- CNRS); Franziska Matthies-Wiesler, Annette Peters (Helmholtz Zentrum München);Daniel Zalko (French National Institute for Agricultural Research-INRAE); Cristina Villanueva, Cathryn Tonne, Elisabeth Cardis, Elizabeth Diago-Navarro, Josep M. Antó, Maria Foraster, Mark Nieuwenhuijsen; Kurt Straif (ISGlobal); Karin van Veldhoven, Kristine Belesova, Neil Pearce, Andy Haines (London School of Hygiene & Tropical Medicine); Jana Klánová, Kateřina Šebková, Lukáš Pokorný, Klára Hilscherová (Masaryk University); Sandra Boekhold, Brigit Staatsen, Nina van der Vliet (National Institute for Public Health and The Environment-RIVM); Eeva Furman, Riikka Paloniemi, Aino Rekola, Marianne Aulake (Finnish Environment Institute-SYKE); Vivienne Byers, Alan Gilmer (Technological University of Dublin); Anke Huss, Roel Vermeulen (Utrecht University); Rémy Slama, Michel Samson (INSERM), (Sinaia Netanyahu, Julia Nowacki (WHO). Other contributors to the HERA agenda writing include Maria Albin (Karolinska Institutet), Åke Grönlund (Örebro University), and Jeanne Garric (French ministry of research)
The HERA project was funded from the European Union’s Horizon 2020 research and innovation programme under grant agreement No 825417.
Author information
Authors and affiliations.
Department of Environmental Science, Stockholm University, Stockholm, Sweden
Elina Drakvik & Åke Bergman
Department of Environmental Medicine, Karolinska Institutet, Stockholm, Sweden
Elina Drakvik
ISGlobal, Barcelona, Spain
Manolis Kogevinas
CIBER Epidemiologia Y Salud Pública (CIBERESP), Madrid, Spain
Universitat Pompeu Fabra (UPF), Barcelona, Spain
IMIM (Hospital del Mar Medical Research Institute), Barcelona, Spain
Université de Paris, Inserm Unit, 1124, Paris, France
Anais Devouge & Robert Barouki
You can also search for this author in PubMed Google Scholar
- Robert Barouki
- , Manolis Kogevinas
- , Åke Bergman
- , Elina Drakvik
- , Anaïs Devouge
- , Denis Sarigiannis
- , Delphine Destoumieux-Garzón
- , Franziska Matthies-Wiesler
- , Annette Peters
- , Daniel Zalko
- , Cristina Villanueva
- , Cathryn Tonne
- , Elisabeth Cardis
- , Elizabeth Diago-Navarro
- , Josep M. Antó
- , Maria Foraster
- , Mark Nieuwenhuijsen
- , Kurt Straif
- , Karin van Veldhoven
- , Kristine Belesova
- , Neil Pearce
- , Andy Haines
- , Jana Klánová
- , Kateřina Šebková
- , Lukáš Pokorný
- , Klára Hilscherová
- , Sandra Boekhold
- , Brigit Staatsen
- , Nina van der Vliet
- , Eeva Furman
- , Riikka Paloniemi
- , Aino Rekola
- , Marianne Aulake
- , Vivienne Byers
- , Alan Gilmer
- , Anke Huss
- , Roel Vermeulen
- , Rémy Slama
- , Michel Samson
- , Maria Albin
- , Åke Grönlund
- & Jeanne Garric
Contributions
All authors contributed equally to the manuscript. All authors read and approved the final manuscript.
Corresponding author
Correspondence to Manolis Kogevinas .
Ethics declarations
Ethics approval and consent to participate, consent for publication, competing interests.
The authors declare that they have no competing interests.
Additional information
Publisher's note.
Springer Nature remains neutral with regard to jurisdictional claims in published maps and institutional affiliations.
Rights and permissions
Open Access This article is licensed under a Creative Commons Attribution 4.0 International License, which permits use, sharing, adaptation, distribution and reproduction in any medium or format, as long as you give appropriate credit to the original author(s) and the source, provide a link to the Creative Commons licence, and indicate if changes were made. The images or other third party material in this article are included in the article's Creative Commons licence, unless indicated otherwise in a credit line to the material. If material is not included in the article's Creative Commons licence and your intended use is not permitted by statutory regulation or exceeds the permitted use, you will need to obtain permission directly from the copyright holder. To view a copy of this licence, visit http://creativecommons.org/licenses/by/4.0/ . The Creative Commons Public Domain Dedication waiver ( http://creativecommons.org/publicdomain/zero/1.0/ ) applies to the data made available in this article, unless otherwise stated in a credit line to the data.
Reprints and permissions
About this article
Cite this article.
Drakvik, E., Kogevinas, M., Bergman, Å. et al. Priorities for research on environment, climate and health, a European perspective. Environ Health 21 , 37 (2022). https://doi.org/10.1186/s12940-022-00848-w
Download citation
Received : 27 December 2021
Accepted : 10 March 2022
Published : 28 March 2022
DOI : https://doi.org/10.1186/s12940-022-00848-w
Share this article
Anyone you share the following link with will be able to read this content:
Sorry, a shareable link is not currently available for this article.
Provided by the Springer Nature SharedIt content-sharing initiative
- Research agenda
- Impact assessment
- Infrastructures
- Transformational change
Environmental Health
ISSN: 1476-069X
- General enquiries: [email protected]
The role of research in environmental science and health
Affiliation.
- 1 Department of Biochemistry, University of Texas Health Center at Tyler 75708-3154, USA.
- PMID: 10531806
Research plays a crucial central role between physicians and regulatory agencies in assessing the potential risks posed by an ever-increasing variety of environmental pollutants. The explosion in our understanding of biology and the development of the powerful tools of molecular biology during the last 50 years have provided us with a unique opportunity to apply this knowledge to predict the dangers of these pollutants and to act to protect the public where appropriate. To realize this vision requires multidisciplinary cooperation, continued research funding, and improved scientific literacy among the public. In this article, we do not attempt to review all the literature relating the role that research has played in environmental health and science, but rather we hope to provide the reader with a broad overview of the role that research has played in the past, emphasizing our own area of expertise (the respiratory system) and the role that research should play as our increasingly complex society moves into the 21st century.
- Environmental Health*
- Environmental Medicine*
- Environmental Pollution*
- Forecasting
- Interprofessional Relations
- Research / trends
Online Students
For All Online Programs
International Students
On Campus, need or have Visa
Campus Students
For All Campus Programs
What is Environmental Science?
Understanding the Numbers When reviewing job growth and salary information, it’s important to remember that actual numbers can vary due to many different factors — like years of experience in the role, industry of employment, geographic location, worker skill and economic conditions. Cited projections do not guarantee actual salary or job growth.
Ten years after the first Earth Day in 1970, environmental activist Senator Gaylord Nelson wrote a letter about the importance of the environment to the American people and the world.
“So long as the human species inhabits the Earth,” he wrote in the letter , “proper management of its resources will be the most fundamental issue we face. Our very survival will depend on whether or not we are able to preserve, protect and defend our environment.”
Thanks to activists like Gaylord and so many others, more educational studies and legal statutes were put into place to make our world cleaner and resources sustainable. The field of environmental science was expanded from these efforts, as well as the associated education that helps our communities and businesses understand how to help the environment. The more we learn about our environment, the better we can protect it for the future generations.
What's the Simple Definition of Environmental Science?
Nugent authors a recurring citizen science column for the National Science Teaching Association (NSTA) and is founder and director of The Great Outdoor Classroom Project. She said some of the disciplines integrated into environmental science include:
- Earth sciences
- Life sciences
- Physical sciences
- Social sciences
“If you want to make a difference for the health of the planet, it is an excellent field to immerse in and study,” she said.
Why is Environmental Science Important?
And she knows that firsthand. In addition to her role as an adjunct instructor at SNHU, Thrippleton-Hunter is a health risk assessor for the New Hampshire Department of Environmental Services.
“Understanding how the natural world works and how we interact with and impact that natural world can help us determine the most efficient and appropriate ways we can reduce those impacts or develop solutions to address those environmental problems,” she said.
In 1970, the Environmental Protection Agency (EPA) was created. With it came the Clear Air Act of 1970, restrictions on lead-based paint, focus on national air quality, the Clean Water Act, studies on pesticides and more, according to the EPA.
Now, continuing this research and sustainable education are crucial to keeping our ecosystems in balance, reversing damage we’ve done and preventing future destruction.
“Environmental science is important to all of us because we have no ‘Planet B’ to inhabit,” said Nugent.
Environmental Science Careers
She said you might think that the field of environmental science is only for those who intend to become environmental scientists, but that isn't your only option.
“There are also other careers in environmental science,” Thrippleton-Hunter said, “like environmental consulting, sustainability, environmental justice, environmental or urban planning, landscape architecture, environmental health, community engagement and policy analysis.”
Educational entities like schools, zoos, nature centers and museums are places those entering environmental studies careers could find a home, but this path also extends to other sectors – including government agencies on local, state and federal levels.
- Business and Government – Professionals who study sustainability and environmental compliance, such as in an MBA program , examine the effects an organization's practices have on the environment and find solutions they can implement to be more sustainable.
- Research – Environmental science researchers examine environmental issues using the scientific method, monitor these issues over time and collaborate to determine solutions. SNHU's environmental science degree offers concentrations in "Data Analytics and Science" and "Natural Resources and Conservation," both of which could offer you a path to pursue a career in environmental science research.
- Education – Environmental scientists work with everyone from politicians and journalists to health professionals and members of the community to educate and help people understand the importance of environmental sustainability , as well as how to help the environment.
“For those that enjoy nature and being outdoors, they could pursue environmental scientist or technician careers, careers as park rangers or game wardens,” Thrippleton-Hunter said. “There are so many options.”
According to the U.S. Bureau of Labor Statistics ( BLS ), most jobs for environmental scientists and specialists require at least a bachelor's degree. Growth in the field is faster than average, BLS reports, and in 2022 the median pay for these roles was $76,480.*
Find Your Program
Why should you study environmental science.
Nugent described environmental education as encompassing the practice of engaging individuals, organizations and communities to learn more about the environment by both informing them and inspiring them to act.
Whether a conservationist, ecologist or geospatial technologist, the information these environmental scientists collect helps to raise awareness of environmental problems and define the steps needed to improve our relationship with our world.
“Environmental studies can take place from a multitude of perspectives including science, social science, business and economics,” Nugent said.
▸ Benefits of an Environmental Science Degree
“Many students who complete the environmental science degree at SNHU go on to work as environmental scientists for government agencies such as the Environmental Protection Agency, their state Department of Environmental Protection and the National Park Service,” Davey said.
She noted that students in this program will learn a variety of skills, including:
- Communication
- Data collection
- Lab and field experience
According to Nugent, SNHU's program includes opportunities for hands-on experience, internships, real world projects and more. She recommended asking your academic advisor about science STEM experiential learning and internship opportunities.
After working as a dancer teacher, Mcmenemy decided to change careers and earn a degree from SNHU with plans to become a conservation officer. “I was so happy that I could prove to myself that I could actually do it,” she said.
Environmental Education in Action
Although environmental education and a field such as business may seem worlds apart, businesses can save money by educating their workers and implementing new policies focused on environment sustainability. The EPA presented a small newspaper’s practices for waste prevention as an example of how sustainability helps businesses.
By selling paper waste for packaging material, reusing ink cartridges multiple times before buying new ones and finding innovative ways to use film-developing chemicals and other materials that would have gone to waste, the newspaper was able to reduce costs.
The EPA’s big selling point for sustainable education being put into practice by businesses is simple: “The bottom line is that preventing waste will save you money," their small business waste prevention guide said.
Nugent also noted that businesses can promote environmental sustainability by making it a part of their mission and business strategy.
“Today, there are unlimited opportunities for businesses to promote environmental suitability through consumer and employee engagement, by developing innovative energy-efficient products from renewable resources and cultivating a culture of sustainable practices across the brand,” she said.
And you can make an impact as an individual, too.
“With a passion for the field combined with proactive engagement, you can make a positive difference for the health of our planet,” said Nugent.
Discover more about SNHU’s environmental science degree : Find out what courses you’ll take, skills you’ll learn and how to request information about the program.
*Cited job growth projections may not reflect local and/or short-term economic or job conditions and do not guarantee actual job growth. Actual salaries and/or earning potential may be the result of a combination of factors including, but not limited to: years of experience, industry of employment, geographic location, and worker skill.
Ashley Wallis is an Army veteran and writer with a BA in English Language and Literature from SNHU. She is currently living in the Denver area.
Explore more content like this article
What Does a Data Analyst Do?
What Can You Do With an Associate Degree in Information Technology?
How to Become a Software Engineer
About southern new hampshire university.
SNHU is a nonprofit, accredited university with a mission to make high-quality education more accessible and affordable for everyone.
Founded in 1932, and online since 1995, we’ve helped countless students reach their goals with flexible, career-focused programs . Our 300-acre campus in Manchester, NH is home to over 3,000 students, and we serve over 135,000 students online. Visit our about SNHU page to learn more about our mission, accreditations, leadership team, national recognitions and awards.
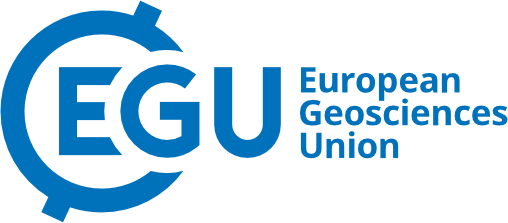
Article
- Volume 24, issue 9
- ACP, 24, 5369–5388, 2024
- Peer review
- Related articles
- 20 years of Atmospheric Chemistry and Physics
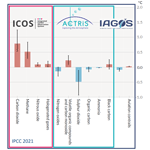
Opinion: New directions in atmospheric research offered by research infrastructures combined with open and data-intensive science
Andreas petzold, ulrich bundke, anca hienola, cathrine lund myhre, alex vermeulen, angeliki adamaki, werner kutsch, valerie thouret, damien boulanger, markus fiebig, markus stocker, zhiming zhao.
The acquisition and dissemination of essential information for understanding global biogeochemical interactions between the atmosphere and ecosystems and how climate–ecosystem feedback loops may change atmospheric composition in the future comprise a fundamental prerequisite for societal resilience in the face of climate change. In particular, the detection of trends and seasonality in the abundance of greenhouse gases and short-lived climate-active atmospheric constituents is an important aspect of climate science. Therefore, easy and fast access to reliable, long-term, and high-quality observational environmental data is recognised as fundamental to research and the development of environmental forecasting and assessment services. In our opinion article, we discuss the potential role that environmental research infrastructures in Europe (ENVRI RIs) can play in the context of an integrated global observation system. In particular, we focus on the role of the atmosphere-centred research infrastructures ACTRIS (Aerosol, Clouds and Trace Gases Research Infrastructure), IAGOS (In-service Aircraft for a Global Observing System), and ICOS (Integrated Carbon Observation System), also referred to as ATMO-RIs, with their capabilities for standardised collection and provision of long-term and high-quality observational data, complemented by rich metadata. The ATMO-RIs provide data through open access and offer data interoperability across different research fields including all fields of environmental sciences and beyond. As a result of these capabilities in data collection and provision, we elaborate on the novel research opportunities in atmospheric sciences which arise from the combination of open-access and interoperable observational data, tools, and technologies offered by data-intensive science and the emerging collaboration platform ENVRI-Hub, hosted by the European Open Science Cloud (EOSC).
- Article (PDF, 2707 KB)
- Article (2707 KB)
- Full-text XML

Petzold, A., Bundke, U., Hienola, A., Laj, P., Lund Myhre, C., Vermeulen, A., Adamaki, A., Kutsch, W., Thouret, V., Boulanger, D., Fiebig, M., Stocker, M., Zhao, Z., and Asmi, A.: Opinion: New directions in atmospheric research offered by research infrastructures combined with open and data-intensive science, Atmos. Chem. Phys., 24, 5369–5388, https://doi.org/10.5194/acp-24-5369-2024, 2024.
Beyond doubt, we have entered the Anthropocene (Steffen et al., 2011) and are now in a period where humankind is the main determinant in the fate of the planet Earth. Natural as well as anthropogenic factors lead to environmental changes on all scales from local to global. Documenting and quantifying these changes are necessary requirements for advancing our scientific understanding of Earth and its environment, including its complex feedback mechanisms. Such documentation and quantification are also needed for developing mitigation and adaptation strategies, for fact-based decision-making, and for the development of environment-friendly innovations. Furthermore, reliable predictions of environmental change must be based on trustworthy, well-documented, observational data which capture the entire complexity of the Earth system and the manifold interactions between the atmosphere, the land, and the ocean and impacts from and on life in all its forms. Ultimately, observational data from all segments of the planet Earth and its environment provide the scientific basis for analysing the physical, biological, and economic processes on our planet which are affecting all sectors of society as well as wildlife and biodiversity (IPCC, 2021a, 2023).
The requirements for a global climate observing system are now widely discussed. The Global Climate Observing System (GCOS, 2010, 2016, 2022b) international programme, co-sponsored by the World Meteorological Organization (WMO), the Intergovernmental Oceanographic Commission of the United Nations Educational, Scientific and Cultural Organization (IOC-UNESCO), the United Nations Environment Programme (UN Environment), and the International Science Council (ISC), introduced “essential climate variables” (ECVs) to provide a science-based framework for coordinated climate observations; see more on that topic in Sect. A2 in the Appendix. Founded on this framework, Weatherhead et al. (2018) proposed one potential approach towards the observing system of the future and noted that for each grand challenge identified by the World Climate Research Programme (WCRP, 2024), observations are needed for long-term monitoring, process studies, and forecasting capabilities. The proposed observing system should include satellite-borne instruments and ground-based and airborne in situ observations as well as potentially new, unidentified observational approaches.
In recent contributions, Carmichael et al. (2023) from the WMO Global Atmosphere Watch (WMO GAW) programme and Kulmala and co-workers (Kulmala, 2018; Kulmala et al., 2023a, b) discuss critically the requirements for a long-term sustained and research-driven global observation infrastructure for atmospheric composition. Carmichael and co-authors identify an “urgent need to move from opportunities-driven one-component observations to more systematic, planned multifunctional infrastructure, where the observational data flow is coupled with Earth system models to serve both operational and research purposes”. Despite their different foci, all articles conclude that comprehensive and open data are essential to meet environmental grand challenges.
To cope with the scale and complexity of the threats posed to our planet by climate change, environmental sciences need to integrate excellent curiosity-driven science in the traditional fields of Earth system sciences with science focusing on human interactions and societal grand challenges. The task is huge, but the means are there. The Strategy Working Group on Environment of the European Strategy Forum on Research Infrastructures (ESFRI) has identified in their recent landscape analysis of the environment domain (ESFRI, 2021a) that European environmental research infrastructures (ENVRI RIs) in atmospheric sciences, jointly with the other branches of environmental sciences, are well positioned to tackle the challenges of climate change at all levels.
In our opinion article, we discuss the potential role that atmosphere-centred ENVRI RIs in Europe can play with their capabilities for standardised collection of observational data complemented by rich metadata and their provision through open access. As a result of these capabilities in data collection and provision of data and services, we elaborate on the novel research opportunities and methods that arise from the combination of open-access and interoperable environmental data and the tools and technologies offered by data-intensive science. It should be noted that the focus of our opinion article is on atmospheric sciences and the atmosphere-centred research infrastructures (ATMO-RIs) providing continuous data on atmospheric composition from a European-wide network of surface-based observation stations (ACTRIS, ICOS) and a fleet of passenger aircraft equipped with scientific instrumentation on board (IAGOS). However, most of the conclusions drawn apply to other areas of environmental research as well.
2.1 Atmosphere-centred research infrastructures in Europe
Recognising that the in-depth investigation of the effects of climate change and their impacts on human health, food security, and other aspects of the United Nations Sustainable Development Goals (SDG, 2024) requires a critical mass in terms of scientific capacities and resources, the European Commission has initiated the development and implementation of environmental research infrastructures at the European level. In the early 2000s, ESFRI started a roadmap process to establish a comprehensive landscape of pan-European environmental research infrastructures (ESFRI, 2021b).
The atmosphere-centred ESFRI environmental research infrastructures include the Aerosol, Clouds and Trace Gases Research Infrastructure (ACTRIS); the In-service Aircraft for a Global Observing System (IAGOS); and the Integrated Carbon Observation System (ICOS) Atmosphere Thematic Centre. As is illustrated in Fig. 1, the ATMO-RIs cover the main contributors to the observed warming (IPCC, 2021a). In the Appendix to this opinion article, we describe in detail the atmosphere-centred RIs and their achievements; also visit the website of the community of environmental research infrastructures (ENVRI, 2024) for further information.
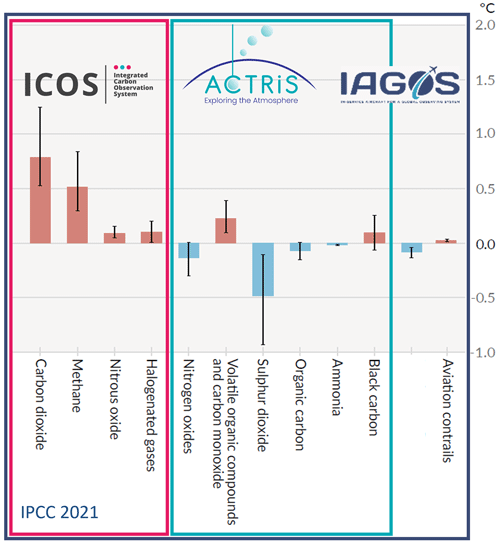
Figure 1 Coverage of the main contributors to the observed warming; only the variable “Land-use reflectance and irrigation” is not covered by the ATMO-RIs; the figure is adapted from Fig. SPM.2 in “Summary for Policymakers” (IPCC, 2021b).
The operational ATMO-RIs are comprehensively prepared to assume their role in this important field of cross-disciplinary research, jointly with life sciences and social sciences. The currently ongoing European research projects entitled Research Infrastructures Services Reinforcing Air Quality Monitoring Capacities in European Urban & Industrial AreaS (RI-URBANS, 2024) and ICOS Cities (ICOS-Cities, 2024) are driving the development and implementation of air quality and greenhouse gas monitoring systems that will allow European cities as well as health administrations and agencies to effectively mitigate the impact of poor air quality on human health and to support climate action.
2.2 Impact of cross-disciplinary research supported by ATMO-RIs
The scientific power of this kind of production and use of environmental data is impressively demonstrated by the series of reports published by the Intergovernmental Panel on Climate Change (IPCC); see the Sixth Assessment Report (IPCC, 2021a) as its latest product. The urgency of further integrating scientific, political and societal efforts in view of the rapidly advancing climate change is presented in the most recent IPCC Synthesis Report (IPCC, 2023).
The scientific importance of long-term greenhouse gas observations as they have been conducted for many years by ICOS and, more recently, by IAGOS has been confirmed in the scientific background document on the 2021 Nobel Prize in Physics, which went to Klaus Hasselmann, Syukuro Wanabe, and Giorgio Parisi “For groundbreaking contributions to our understanding of complex physical systems”. In Chap. IV, the Nobel Committee writes, “From the perspective of laboratory science, using experimental measurements to test theory is such a self-evident step in the scientific method that it goes without saying. However physical cosmology and physical climatology are observational sciences – practitioners observe that which nature allows”.
Moreover, failure to reduce greenhouse gas emissions and concentrations over the next 2 decades is perhaps the greatest threat to the future health of human society and ecosystems. The role of the ATMO-RIs is critical to achieving efficient, effective, and verifiable global emission reductions, particularly for the control of emission reductions required as part of the COP21 Paris Agreement of 2015. The mitigation measures and the speed of their implementation need to be checked by independent methods and closely monitored, while the influence of natural feedback due to ongoing climate change will require attention, as this may force a change in the speed of implementation of mitigation measures and adaptation. The contribution of ATMO-RIs to the validation exercise of emission reductions is described by Vermeulen et al. (2020).
Another success story of the cross-discipline use of atmospheric observational data is the Copernicus Atmosphere Monitoring Service (CAMS; Peuch et al., 2022). CAMS delivers numerous global and regional information products about air quality, inventory-based emissions, and observation-based surface fluxes of greenhouse gases and about biomass burning, solar energy, ozone and UV radiation, and climate forcings, with the atmosphere-centred research infrastructures being one of its prime data and service contributors.
Besides research on climate change, the scientific field of atmospheric chemistry with its links to climate change, as well as the resulting interaction of air quality and human health, constitutes another highly interdisciplinary research area in the current agenda of atmospheric sciences (von Schneidemesser et al., 2015; The Lancet Planetary, 2020; Romanello et al., 2022). A number of recent post-COVID-19 studies on the effects of reduced anthropogenic emissions on atmospheric chemical composition (e.g. Clark et al., 2021; Evangeliou et al., 2021; Gkatzelis et al., 2021; Sokhi et al., 2021) impressively illustrate the growing need for, and the great potential of, scientific approaches that cross the traditional boundaries of scientific disciplines and methods. The studies utilise long-term and high-quality observations of atmospheric composition on a global scale, combined with statistical approaches, for identifying patterns in atmospheric composition change which can be traced back to modified emission behaviours in different parts of the world.
3.1 Challenges of data-intensive science
The production of scientific knowledge has developed from experimental sciences, by conducting field observations and experiments, and through theoretical approaches using models and mathematical generalisations to simulate phenomena which are too complex for analytical solutions (Gray, 2009). Now we have entered the age of the fourth paradigm of data-intensive science, which is described as collaborative, networked, and data-driven (Bell et al., 2009; Lynch, 2009).
European environmental research infrastructures are perfectly prepared for the challenges of data-intensive science, since they combine research-driven observations with comprehensive capabilities for sustainable data management and stewardship. Astronomy and high-energy physics can serve as role models for this kind of infrastructure (Bell et al., 2009) but with the essential difference that in the majority of cases environmental observations require the operation of widely distributed measurement networks together with the handling of complex data streams from highly diverse sources and with a need for short-term data availability.
The integration of data-intensive sciences and environmental sciences is critical for improving our current understanding of the major challenges to our planet, our ability to respond to and predict natural hazards, and our understanding and prevention of ecosystem loss. This effort requires the unimpeded use of multidisciplinary data from the different spheres of the Earth system, with findability, accessibility, interoperability, and reusability of the digital (meta)data (FAIR principles; Wilkinson et al., 2016) as an absolute prerequisite, embedded in a culture of open scientific cooperation as stated by the European Commission in their European Open Science Cloud (EOSC) Declaration (EC, 2017) and outlined by the Organisation for Economic Co-operation and Development (OECD; Dai et al., 2018).
We would like to recall that the research problems behind environmental and societal challenges such as climate change, food security, and natural disasters are intrinsically interdisciplinary. Therefore, the integration of data-intensive sciences and environmental sciences is crucial to improve our skills to create multidisciplinary and cross-domain scientific knowledge which is needed to act on the grand challenges facing our planet and our societies. Modelling these processes individually is difficult enough, but modelling their interactions is complex by another order of magnitude; see, for example, Bauer et al. (2021) for a discussion of the challenges and opportunities of the digital revolution of Earth system science, using the example of reliable weather forecast and climate predictions.
Among the indispensable prerequisites for data interoperability is the organisation of data management systems at each RI in compliance with the FAIR principles. The entire ENVRI cluster has organised the implementation of the respective FAIR enabling resources along the FAIR pyramid proposed by Bailo (2019); see Fig. 2 for details. As can be seen in Fig. 2, huge and comprehensive efforts are required to accomplish FAIR scientific data management. Most of the FAIR enabling resources need to be implemented at the level of data and metadata management to comply with the respective FAIR principles. Once this foundation has been set up properly, access to science-based services and the use of services can be realised. The RIs of the ENVRI cluster, including the contributing ATMO-RIs, walked this arduous road during the European Commission cluster project ENVRI-FAIR (2019–2023). The reward at the end of the road was a previously unattainable level of data interoperability among the ATMO-RIs which now allows the development and provision of cross-RI scientific services for the scientific community.
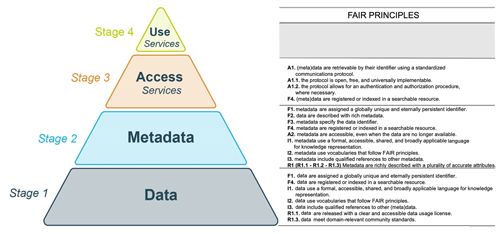
Figure 2 The four-stage FAIR roadmap – FAIR pyramid; adapted from Bailo (2019).
In today's atmospheric sciences and other research areas, scientists are challenged to collaborate across conventional disciplinary boundaries. However, first they must discover and extract data that are dispersed across many different sources and in many different formats. For this step, a data management system based on the FAIR principles will play a crucial role. In addition to this, effective research support environments are needed for various user-centralised research activities, from formulating research problems to designing experiments, discovering data and services, executing workflows, and analysing and finally publishing the results (Zhao and Hellström, 2020). Particularly, the concept of scientific workflows has developed into a powerful tool over the past decade (Atkinson et al., 2017). Scientific workflows provide a systematic way of describing data analysis with the analytical activities, methods, and data it needs (Cerezo et al., 2013). They consist of a series of activities with input and output data and are directed to the description of scientific experiments and data analysis processes. Today, data-intensive workflows are capable of exploiting rich and diverse data sources in distributed computing platforms and can be composed for reusing elements from other analytical processes in new applications; see also Chap. 5 in Bouwer et al. (2022).
FAIR-based data management and scientific workflows are key elements of the full research data life cycle for ENVRI RIs as shown in Fig. 3. The research data life cycle illustrates all elements to be contained in such research support environments, including the publication of data as a critical step for open science. Establishing those effective research support environments is best achieved through a collaborative culture of environmental scientists and computer engineers built on a co-design approach and the implementation of mixed teams consisting of team members from both professions (Schulthess, 2015; zu Castell et al., 2022).
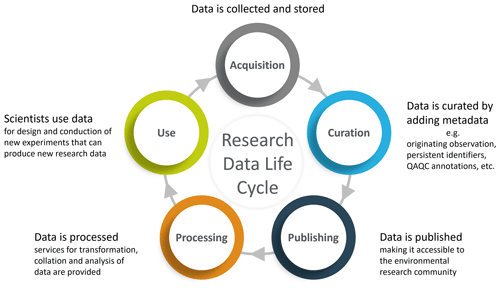
Figure 3 The research data life cycle of ENVRI; adapted from Hidalga et al. (2020). QAQC denotes quality assurance and quality control.
To illustrate with more detail the necessary steps towards interoperable and harmonised ECV data as essential input for research support environments, we concentrate on the most relevant ECVs for climate change, which are temperature and greenhouse gas atmospheric abundance. Numerous in situ stations and satellite-borne systems deliver observational data on these ECVs. These data need to be calibrated, processed (data reduction), interpolated, re-gridded, and subject to quality control in complex workflows to generate higher-level high-quality data sets. These data sets can then be used in model systems to derive other ECV data that answer the relevant science or policy questions. Traceability, transparency, and information on the data quality through the whole value chain are essential for using these data in relevant societal evaluations and decision support systems. The ENVRI RIs have started implementing the required technologies at their RI level and developing the first scientific demonstrators for ECV data interoperability of the ATMO-RIs. Finally, ECV data can be used by interdisciplinary research groups for data assimilation, data fusion, and model development.
Access to FAIR environmental data provided by the ATMO-RIs and to research support environments will be made available to the atmospheric and environmental research communities through the ENVRI-Hub described in the Appendix.
3.2 New technology approaches supporting scientific knowledge production
The availability of effective data and research support environments is complemented by the development of new approaches to scientific knowledge production. Currently, considerable knowledge is recorded in scientific articles. This narrative text-document-based expression of research work is designed for human expert consumption, whereas the knowledge recorded in scientific articles is difficult for machines to access. As a result, machine support in scientific knowledge processing is still inadequate and research communities routinely invest enormous human resources to conduct research synthesis, e.g. in systematic reviews, as information needs to be manually extracted from articles and manually organised to support synthesis. In the coming decade, scientific knowledge infrastructures (Stocker, 2017) must further advance their digital infrastructures so that scientific knowledge is produced to be FAIR by design and therefore more efficiently reusable with advanced machine support. Initiatives and services such as the Open Research Knowledge Graph (Stocker et al., 2023) are demonstrating advanced technologies like knowledge graphs, natural language processing, terminology services, and semantic resources. They also include approaches that integrate the production of FAIR scientific knowledge into data analysis and thus enable the reuse of FAIR scientific knowledge in data science. Recent developments in artificial intelligence are also enabling large language models to extract and synthesise significant information from published scientific narratives. Such initiatives can fundamentally transform digital scholarship and the way digital infrastructures support scientific knowledge production.
Knowledge graphs and, more generally, artificial intelligence technologies, virtual research environment (VRE) services, and FAIR scientific data management are key instruments supporting the production of new scientific knowledge. Data discovery services, such as the ENVRI Knowledge Base search engine (Farshidi et al., 2021), can considerably improve the search quality of research assets. This improvement is achieved by allowing search services to effectively expand search queries with a broader set of relevant and similar keywords and by improving the similarity inference of search results using the additional semantic information provided by the concepts and their relationships in knowledge graphs (Farshidi and Zhao, 2022). Semantic information provided by knowledge graphs can help annotate a scientific experiment or observation strategy with rich meta-information for describing its domain problem, data sets, software tools, and experiment steps. This annotation then allows for effective sharing and reuse of experimental workflows across user communities. As new knowledge is derived from scientific experiments or observations, the publication of scientific papers, research software and data sets will be further linked in knowledge graphs.
4.1 Rethinking the scientific approach
Performing atmospheric research through the scientific data and services provided by the ATMO-RIs and making use of the opportunities offered by open science require a fundamental change in the mindset of individual scientists that will open up new ways of tackling scientific questions of increasing complexity. Particularly, adaptation to the approaches and methods of data-intensive science applied to open data and services from long-term operating research infrastructures calls for rethinking at multiple levels.
At the level of data provision, the creation, curation, and processing of observational data are disconnected from data analysis and scientific exploration, and data provision is realised by the ATMO-RIs through the ENVRI-Hub or other open-access data sources, supported by rich metadata. At the level of scientific exploration, large data sets will remain at the source while data analysis is brought to the data by means of science-based composable workflows running in analytical frameworks such as Jupyter notebooks. Open-source programming environments like GitHub foster the collaborative development and application of data analytical tools and methods in science teams which are ideally composed of both domain scientists and software developers and are restricted neither institutionally nor regionally because of the technologies for remote work offered by the worldwide web.
On the conceptual side, the database accessible to scientists is significantly broadened by the implementation of the science-based framework of ECVs for the provision of ATMO-RI data, combined with the transparent reporting of provenance information in accordance with the FAIR principles and the discoverability of data via enhanced search capabilities though standardised keywords and vocabularies. Making use of open-source programming environments linked with harmonised standard operating procedures for instruments measuring ECVs in accordance with the GCOS-defined requirements (GCOS, 2022a) for data accuracy and precision promotes the collaborative development of data quality assurance and control procedures across the ATMO-RIs, culminating in an increasingly consistent data set of ECVs. In analytical frameworks made available by the ATMO-RIs as well as other ENVRI-RIs through the ENVRI-Hub, ECV-based scientific workflow templates will provide the best data handling, processing, analysis, and visualisation experience. Uses include data cleaning and transformation, numerical simulation, statistical modelling, data visualisation, and machine learning.
New ways of scientific knowledge creation are in reach when leaving the traditional path of working with self-generated data or data from a scientific collaboration and analysing the data within the involved research teams. Recalling that data-intensive science is characterised as collaborative, networked, and driven by immense quantities of data, the access to open data, open software, and resources of previously unknown computational power, combined with a positive attitude towards knowledge sharing, indeed offers a new scientific approach towards complex scientific questions which was not possible before. However, one of the most important bottlenecks in this context is the training of current and future scientists working in our field to familiarise them with the new concepts, tools, and methods.
4.2 Research infrastructures as key facilitators of the new scientific approach
The environmental research infrastructures organised in the ENVRI cluster have covered a considerable part of the way towards a harmonised global climate observation system complemented by open-access and interoperable reliable data, as requested by WMO (Weatherhead et al., 2018; Carmichael et al., 2023) or by the initiative taken by Kulmala (2018) and Kulmala et al. (2023a, b). Making data and scientific products available through free and open access further broadens the use and reuse of the scientific information provided by ACTRIS, IAGOS, and ICOS and finally of the scientific knowledge gained from the continuous operation of the ATMO-RIs. Already today, the atmosphere-centred environmental research infrastructures play a central role in the evolving global climate observing system through the provision of scientific data and knowledge to institutional users like the Copernicus Atmosphere Monitoring Service or the Global Atmosphere Watch programme of the World Meteorological Organization. Through the efforts undertaken by the ATMO-RIs jointly with the other RIs of the ENVRI cluster towards the provision of FAIR data with open access and supported by analytical frameworks, the benefits for the global scientific community in climate sciences and beyond will continuously increase and offer new research options, particularly to a new generation of scientists familiar with data-intensive tools and methods.
Continuing on the outlined path to FAIR and open data in the environmental sciences will not only open the door to broader use of data and scientific products by the global scientific community, especially across traditional scientific fields, but also foster the deeper integration of environmental, life, and social sciences, which is needed to respond to the grand societal challenges we face. The emerging facilities provided by EOSC and the ENVRI-Hub as an EOSC-hosted platform for the collaboration and co-design of the ENVRI RIs will play a central role in this integration of research infrastructures towards interoperability of data and services.
It must be noted, though, that during this integration process, the integrity and functionality of the independent research infrastructures need to be maintained. Their operational requirements are very different and call for tailored solutions for infrastructure design and implementation. Integration then takes place at the level of providing scientific data and services, as well as access to the individual infrastructures. The sustainable success model therefore consists of highly specialised, unique, and independent research infrastructures that integrate as much as possible in the provision and interoperability of data, services, and access to research infrastructure digital and physical assets of any kind.
A1 Environmental research infrastructures in atmospheric sciences
A1.1 the community of environmental research infrastructures.
By definition in Article 2 (6) of Regulation (EU) No 1291/2013 of 11 December 2013 (EC, 2013), “research infrastructures” mean facilities, resources, and services that are used by research communities to conduct research and foster innovation in their fields. Where relevant, they may be used beyond research, for example for education or public services. They include major scientific equipment or sets of instruments; knowledge-based resources such as collections, archives, or scientific data; e-infrastructures such as data and computing systems and communication networks; and any other infrastructure of a unique nature essential to achieving excellence in research and innovation.
The science cluster of ESFRI-listed environmental research infrastructures (ENVRI) in Europe was formed as a strong community of principal producers and providers of environmental research data and research services in Europe. For most of the ESFRI-listed RIs, access to their services is not simply limited to digital (data) services. Moreover, physical and remote access to the different types of research facilities (i.e. central laboratories, reference instruments, research platforms) is another integral part of their service portfolio and fulfilment of their research and innovation strategies. The science cluster of environmental RIs is one out of five science clusters of the ESFRI list of RIs. The other clusters focus on energy, health and food, physical sciences and engineering, and social and cultural innovation; see the latest ESFRI Roadmap report for more information (ESFRI, 2021b).
In the past decade, through the European Commission cluster projects ENVRI (2011–2014), ENVRIplus (2015–2019), and ENVRI-FAIR (2019–2023), the ENVRI RIs have created a robust conceptual and technical framework based on the FAIR principles that empowers the ENVRI cluster to provide discipline- or domain-oriented (e.g. atmosphere, marine, land system) or thematically/methodologically oriented (e.g. carbon cycle) services in support of climate change research in all its facets (Petzold et al., 2019). This framework includes common standards and policies for the data life cycle, including cataloguing, curation, provenance, and service provision within the ENVRI cluster, with specific consideration of the FAIR principles including interoperability solutions and open standards, e.g. on metadata description. Open access to data sets generated, hosted, and curated by the ENVRI RIs is another integrating element of this framework, which is currently realised at the RI level but will be lifted to cluster level once cross-RI authentication and authorisation infrastructures (AAIs) are in place. The numerous aspects tackled during these joint efforts are described in detail by Zhao and Hellström (2020).

A1.2 Atmosphere-centred research infrastructures
The ENVRI landscape of atmosphere-centred environmental research infrastructures (ATMO-RIs) is illustrated in Table A1, whereas Table A2 compiles brief descriptions of the ATMO-RIs, with the Aerosol, Clouds and Trace Gases Research Infrastructure (ACTRIS); the In-service Aircraft for a Global Observing System (IAGOS); and the Integrated Carbon Observation System (ICOS) Atmosphere Thematic Centre performing research on the chemical composition of the atmosphere in a changing climate. The Svalbard Integrated Arctic Earth Observing System (SIOS) organises multi-domain observation platforms on the Svalbard archipelago in the Arctic region, with atmosphere-related research data largely provided via ACTRIS and ICOS, while EISCAT_3D concentrates on the dynamics of the mesosphere. Thus, the ATMO-RI landscape consists of three RIs targeting solely atmospheric research topics (ACTRIS, EISCAT, IAGOS), and two multi-domain RIs with an atmospheric component (ICOS, SIOS).
Table A1 The landscape of environmental research infrastructures in Europe (ENVRI) in atmospheric sciences in a nutshell.
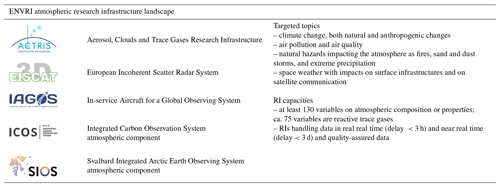
Download Print Version
Table A2 ESFRI environmental research infrastructures of the atmospheric sciences.
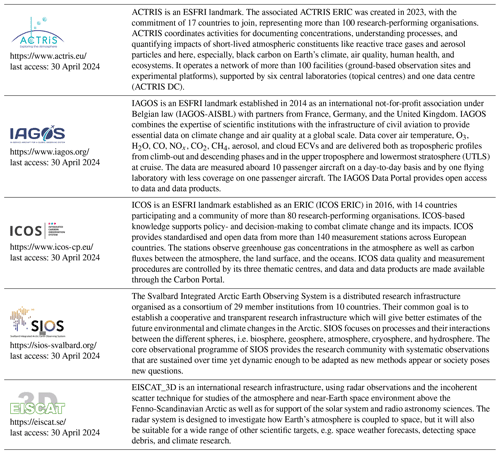
Harmonising and securing the landscape of atmospheric observations
Historically, the ATMO-RIs have developed from independent research projects which started their integration processes with the emerging ESFRI Roadmap process in the early 2000s. All predecessor projects focused on different research targets but in similar research fields. ACTRIS brought together ground-based cloud remote sensing measurements (CloudNET), the European Supersites for Atmospheric Aerosol Research (EUSAAR), the European Aerosol Research Lidar Network (EARLINET), the European atmospheric simulation chambers (EUROCHAMP), and the European component of a number of additional monitoring networks (Laj et al., 2024). IAGOS merged two projects on the measurements of ozone, water vapour, and other trace species by a fleet of in-service Airbus aircraft (MOZAIC; Marenco et al., 1998) or by means of one instrumented airfreight container (CARIBIC; Brenninkmeijer et al., 1999) into one infrastructure on atmospheric observation by civil passenger aircraft (Petzold et al., 2015; Thouret et al., 2022). ICOS was built around the scientific target of continuous, long-term observations of the carbon cycle, including the quantification of greenhouse gas emissions and sinks and their impacts on Earth systems (Heiskanen et al., 2022). Predecessor projects are numerous ( > 25), and Fig. 1 in Franz et al. (2018) illustrates the fragmentation of observation networks in Europe which then developed into ICOS. While ACTRIS, IAGOS, and ICOS infrastructures are characterised by strongly distributed observation networks, EISCAT and SIOS have emerged from single-sited activities.
Looking at the evolution of the ATMO-RIs, one important achievement of the research infrastructure implementation process becomes immediately apparent, namely the integration of a formerly scattered landscape of multiple observation networks into a few research infrastructures. The direct benefits of this consolidation process were joining resources of all kinds, reducing duplication of work, and offering data of previously unknown quality and complexity. Before the consolidation phase, data had been locked in silos and had been poorly standardised over decades, hampering scientific progress severely. The first harmonisation efforts were undertaken by the Global Atmosphere Watch programme of WMO, but during the consolidation process, the ATMO-RIs developed cutting-edge standardisation for their observations and implemented highly efficient data life cycle management systems, leading to timely, open, and FAIR data access by scientists all over the world. Tackling the next step, the ATMO-RIs joined forces in the European Union's Horizon 2020 project “Sustainable Access to Atmospheric Research Facilities” (ATMO-ACCESS, 2024) for establishing a comprehensive and sustainable framework for access to distributed atmospheric RIs, ensuring integrated access to and optimised use of the services they provide.
Another major achievement of the ATMO-RIs which deserves explicit mention is the conservation of long-term atmospheric observation stations and time series which were in danger during the transition process from research projects to research infrastructures. The detection of trends and seasonality in the presence of greenhouse gases and short-lived climate-active atmospheric constituents is an important aspect of climate science. An accurate description of trends relies heavily on the ability to place the measurements of all kinds of climate-active atmospheric constituents in a historical context, i.e. to compare measurements against measurements from the same location in preceding years and decades. From a scientific perspective, longer time series reduce the uncertainties in the interpretation of current measurements. The length of time series which are produced by the different ATMO-RIs is therefore a good indicator of how well these data meet the requirements of climate scientists. It is important to note that the use of long time series of observations for trend analysis requires a measurement infrastructure that guarantees inter-comparability in terms of observation precision and accuracy over the period of interest. Such inter-comparability generally requires constant vigilance and support, which operational RIs secure.
To give examples, we may first refer to the long-term in situ observations of ozone and water vapour in the upper troposphere and lowermost stratosphere by passenger aircraft equipped with MOZAIC instrumentation (Marenco et al., 1998), starting in 1994. These long-term data not only could be secured but also were subjected to the most modern data quality assurance standards, and the collection of data successfully continued after the transition of MOZAIC to IAGOS was completed. Today these data sets cover the climatologically relevant length of 30 years and are widely used, e.g. in the IPCC reports, in the Tropospheric Ozone Assessment Report (Schultz et al., 2017; Tarasick et al., 2019), and in climatological studies (Petetin et al., 2016; Gaudel et al., 2018, 2020; Petzold et al., 2020). The representativeness of the IAGOS airborne measurements in the lower troposphere was explicitly investigated to ensure the applicability of these airborne data sets in the context of air quality studies (Petetin et al., 2018). Without the implementation of IAGOS as a research infrastructure, the historical data would not be available and the continuation of observations would not have been possible.
The second example focuses on the measurements of greenhouse gases and the carbon cycle that comprise a core element in the scientific fields of ecology and evolution, environmental sciences, atmospheric sciences, forestry, and agronomy. ICOS provides long-term, high-quality observations that follow (and cooperatively set) the global standards for the best possible quality of data on the atmospheric composition of greenhouse gases, greenhouse gas exchange fluxes measured by eddy covariance, and CO 2 partial pressure at water surfaces. Besides these main data products, ICOS also produces observations of many ancillary variables, using the same highest-quality standards. The ICOS measurement infrastructure is in large part built from existing measurement stations which have been updated to provide measurements that meet ICOS standards. Data which were collected before ICOS measurement protocols were put in place have been secured physically and with respect to data quality. They now contribute to the ICOS record of long-term data sets and to the World Data Centre for Greenhouse Gases run by the Japan Meteorological Agency as an extremely important contribution to the global greenhouse gas community in the context of data archiving and dissemination.
Of ICOS' 134 measurement stations, 101 stations provided data on the length of time series held by them. Data from these 101 stations describe how long they have been operational or, in some cases, how long the station has been collecting measurements that are relevant to ICOS. The average length of time series across all domains is 11 years, and this is evenly spread between the ecosystem domain (average 11 years), atmosphere domain (12 years), and ocean domain (11 years); see Fig. A1. Time series from atmosphere stations tend to be the longest, which likely reflects the overlap between historical atmosphere measurements and current variables being measured by ICOS. Details are given in the latest ICOS Impact Assessment Report (Belle et al., 2018).
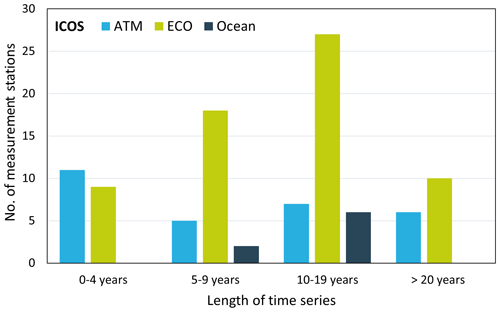
Figure A1 Number of measurement stations and length of time series held by ICOS measurement stations in 2018, for the atmosphere (ATM), ecosystem (ECO), and ocean domains (data source: Belle et al., 2018).
The third example is the aerosol ECV “aerosol single-scattering albedo” retrieved from ACTRIS measurements. ACTRIS covers observations of short-lived constituents of the atmosphere, using in situ and remote sensing methods located on the Earth surface. The series of ACTRIS projects, leading to the establishment of the ACTRIS ERIC, started in 2011, but predecessor projects date back to the year 2000, with single time series of observations having started already in 1995. Figure A2 shows an example of a long time series of aerosol particle scattering and the absorption coefficient (upper and middle panel respectively) observed at the Ispra station in northern Italy. From data with both high quality and high time resolution, the aerosol particle single-scattering albedo can be calculated, an ECV necessary for assessing the direct aerosol climate effect. The single-scattering albedo is shown in the lower panel over the period 2009–2023.
Overall, long-term observations of climate-relevant aerosol properties by ACTRIS became an indispensable ingredient of the global climate observation system and have trigged multiple scientific studies on the long-term aerosol impact on climate (e.g. Zanatta et al., 2016; Collaud Coen et al., 2020; Laj et al., 2020). Trends are detectable in the data, which is possible only due to the collection of data with consistent quality and operating procedures over long time periods.
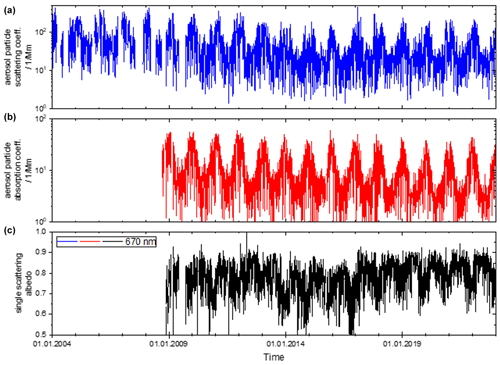
Figure A2 Time series of aerosol particle scattering ( a ; Putaud and Martins dos Santos, 2022b) and absorption coefficients ( b ; Putaud and Martins dos Santos, 2022a), observed at the Ispra station in northern Italy associated with ACTRIS and ACTRIS pre-projects, all at 670 nm wavelength. The data displayed in ( a ) and ( b ) form the basis for calculating the aerosol particle single-scattering albedo, which is declared an essential climate variable (ECV).
Technical achievements
Technically, the ATMO-RIs have definitely pushed the borders of what can be observed. The measurements are highly standardised, connected to unified calibration systems, partially available in near real time, and well distributed over the European continent or even globally. Measurement methods deployed by the ATMO-RIs follow published common specifications and protocols and use synergies among the RIs as efficiently as possible. The data are subject to quality control and processed at dedicated data centres, using open and published processing chains. The RI-specific calibration laboratories provide the stations with working standards and analyse flask samples for trace gas concentrations. All data from raw data up to the final quality-controlled (averaged) data are openly accessible from the RI data portals (ACTRIS and IAGOS data portals, ICOS Carbon Portal).
Today, ICOS provides the densest and most integrated observational network on greenhouse gases worldwide; IAGOS is a globally unique observation infrastructure for atmospheric trace gases, aerosol, and cloud particles which reaches parts of the atmosphere not accessible for in situ observations by other platforms; and ACTRIS constitutes a research infrastructure on short-lived atmospheric constituents like reactive trace gases, aerosols, and clouds, which investigates the processes leading to the variability in these constituents in the natural environment and in controlled atmospheres of unprecedented complexity. The strategic role of the European complementary RIs in the atmospheric sciences (the ATMO-RIs) has been highlighted in a recent IPCC report (IPCC, 2021a; see Sect. 1.5.1.1, “Major expansions of observational capacity”), noting the key contribution of ICOS, IAGOS, and ACTRIS. These three ATMO-RIs are also essential data providers to the Copernicus services in the areas of atmosphere monitoring (CAMS; Wagner et al., 2021; Peuch et al., 2022) and climate change (C3S; Thepaut et al., 2018) as well as to the WMO Global Atmosphere Watch Programme (GAW; Carmichael et al., 2023) and play leading roles in the evolving Global Greenhouse Gas Watch programme.
A2 The science-based framework of essential climate variables
The Earth atmosphere is a complex medium with thousands of chemical species interacting with their environment. Consequently, monitoring the atmospheric composition comprehensively in the long term is impossible. To transform the challenging task of integrated Earth observation into a concept closer to a global climate observation system, GCOS (co-sponsored by WMO) has introduced the concept of essential climate variables (ECVs). ECVs for atmospheric composition are defined as a physical or chemical variable or a group of linked variables that critically contributes to the characterisation of the atmospheric composition (Bojinski et al., 2014). This set of variables includes not only the long-living greenhouse gas CO 2 , but also many of the short-lived climate forcers such as ozone, methane, and aerosol particles and meteorological parameters like air temperature and humidity (water vapour volume mixing ratio, specific humidity, relative humidity). The observables to be recorded for climate monitoring are defined by WMO through the process of the Rolling Review of Requirements in the Observing Systems Capability Analysis and Review Tool (WMO-OSCAR, 2024). WMO has also defined a subset of the existing set of ECVs to provide the most essential parameters representing the state of the climate system. These indicators include global mean surface temperature, global ocean heat content, state of ocean acidification, glacier mass balance, Arctic and Antarctic sea ice extent, global CO 2 mole fraction, and global mean sea level (Trewin et al., 2021).
Among the nine atmospheric application areas in the process of the Rolling Review of Requirements, two are critically relevant to the work of atmospheric research infrastructures.
Atmospheric climate monitoring defines the requirements for climate observations that are essential to detect, model, and assess climate change and its impact; support adaptation to climate change; monitor the effectiveness of policies for mitigating climate change; and develop climate information services. ECVs for the atmosphere, the oceans, and terrestrial systems (Bojinski et al., 2014) are identified for this application area. ECV data sets provide the empirical evidence needed to understand and predict the evolution of climate, to guide mitigation and adaptation measures, to assess risks and enable attribution of climatic events to the underlying causes, and to underpin climate services. The current list of ECVs is specified by the Global Climate Observing System (GCOS, 2010). Updated requirements for the observation of ECVs were recently published (GCOS, 2022a).
The area of atmospheric composition forecasting and monitoring defines requirements related to evaluating and analysing changes (temporally and spatially) in atmospheric composition regionally and globally to support treaty monitoring, climatologies, and re-analyses, assessing trends in composition and emissions/fluxes, and better understanding of processes, using data of controlled quality.
The recommended essential climate variables as defined by GCOS in 2022 are listed in Table A3. A complete list of variables and requirements for atmospheric applications can be found through the OSCAR tool (WMO-OSCAR, 2024). Table A3 also lists the ATMO-RIs covering the respective parameters. ECVs based on total column densities of specific molecules are covered by a variety of satellite-borne instruments and by ground-based remote sensing observational networks such as the Total Carbon Column Observing Network (TCCON; Wunch et al., 2011), the Network for the Detection of Atmospheric Composition Change (NDACC; De Mazière et al., 2018), the federated network for aerosol characterisation AERONET (Holben et al., 1998), or the global ozonesonde network (Tarasick et al., 2021).
Concluding from the latest landscape analysis of the environment domain (ESFRI, 2021a), ENVRI RIs and ATMO-RIs in particular have been indispensable for monitoring and understanding changes in ECVs over several decades. Given the great variety and complexity of observational data provided by all ENVRI RIs, the science-based framework of ECVs offers a concept and guidelines for the implementation of tools and standards on the data and metadata level that concentrate on key variables covered by multiple ENVRI RIs and allow searchability, accessibility, and interoperability of these key ECV data, with the final goal of enabling cross-RI exploitation of data.
Table A3 Essential climate variables for the atmospheric climate monitoring application area as defined in the 2022 GCOS Implementation Plan (GCOS, 2022b) and the ATMO-RIs providing the observations.
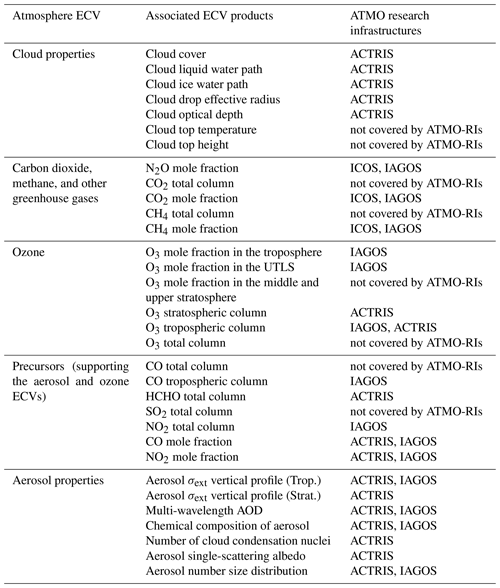
Abbreviations and symbols: Trop., troposphere; Strat., stratosphere; AOD, aerosol optical depth; σ ext , aerosol light extinction coefficient. Note: ACTRIS and ICOS measurements are in any case surface-based and originate either from in situ or from remote sensing techniques; IAGOS measurements originate from in situ profile observations from the boundary layer to the lowermost stratosphere.
Download Print Version | Download XLSX
A3 The ENVRI-Hub
The ENVRI cluster responded to the numerous challenges of data-intensive science by establishing a “Data for Science” theme in their collaborative project ENVRIplus and then by starting the development and implementation of the identified research support environments in the successor project ENVRI-FAIR. These efforts finally evolved into the ENVRI-Hub as a platform for environmental services, data, knowledge, and training to support and increase collaboration and the sharing of information beyond traditional scientific communities (Petzold et al., 2019; Zhao and Hellström, 2020). Since open science is at the core of the ENVRI RIs, the ENVRI-Hub also serves as a robust conceptual and technical framework that will facilitate the integration of the environmental science community into the European Open Science Cloud (EOSC). Details of the ENVRI-Hub architecture, its design criteria, and technical solutions implemented can be found in the ENVRI-Hub white paper (Petzold et al., 2023).
Currently, the ENVRI-Hub is accessible as a demonstrator version (ENVRI-Hub, 2024) with envisaged functionalities not yet fully developed. In its matured stage, the ENVRI-Hub will provide researchers, from, for example, the atmospheric science community, with the necessary services to practise open science through using data and services published in the ENVRI Catalogue of Services. It will provide research products and services to EOSC to enhance researcher, citizen, and industry involvement in open science. ECV workflows, data sets, services, training material, and publications will be made available to EOSC when assets and services reach the adequate maturity level. To ensure the reproducibility of results, e.g. the training and documentation of services, metadata or analytical workflows will be provided and made available through the training platform, assisted by training events. Science demonstrators will be enabled by analytical frameworks, where ECV-based workflow templates will provide the best data handling and visualisation experiences. Applications will include data cleaning and transformation, numerical simulation, statistical modelling, data visualisation, and machine learning. Already today, the ENVRI Community in Zenodo (ENVRI-Community, 2024) contains documentation, reports, and research papers with articles having open-access licences with minimal restrictions. The release of the ENVRI-Hub and the workflows developed on the ENVRI-Hub will be open-source and managed on the Git repository on EGI Infrastructure. The mature releases will also be published in Zenodo.
A4 Science demonstrators as examples for the interoperability of observation infrastructures
Atmospheric scientists entering the ENVRI-Hub though its portal on EOSC will reach graphical user interfaces for accessing data and services, such as map-based portals and lists of catalogued data sets, with semantic-driven search functionalities that enable them to search for appropriate data sets or services using controlled vocabularies of discipline-related keywords. They can use the research support environments in different ways, as illustrated in Fig. A3 for the discovery and use of data (top) or data products and services (bottom), and build their own analytical workflows from components developed beforehand by other scientists and published through the ENVRI-Hub.
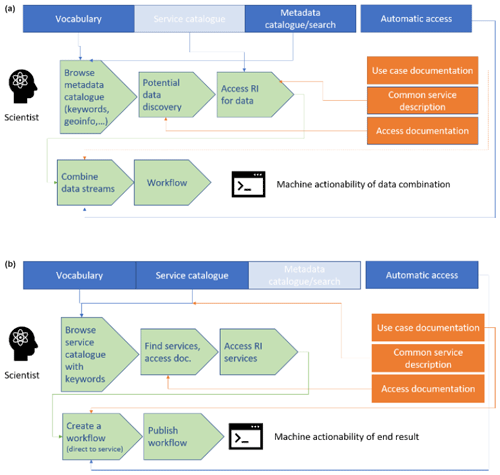
Figure A3 (a) Advanced data discovery and use; (b) advanced service discovery and use with the data in, for example, Jupyter Notebook across RIs (Petzold et al., 2023).
To give a brief description of data search and discovery (Fig. A3, top panel), the scientist browses the metadata catalogue with the help of the vocabulary, discovers data of potential interest, then browses the metadata catalogue for information about the access to the RI of interest with the help of the provided access documentation, and repeats this chain until all data of interest have been collected. Finally, the whole process can be put into a workflow which can be stored for reuse and combination with other workflows. A similar procedure is possible for the advanced service discovery and use (Fig. A3, bottom panel).
The ground for offering the advanced services illustrated in Fig. A3 for atmosphere-centred research questions was prepared through the considerable improvement of cross-RI atmospheric services and interactions achieved during ENVRI-FAIR. As a result, it is now possible to implement cross-RI services relying on the convergence between RIs on important topics like vocabularies, unique identifiers, and licences. The vocabulary within the ATMO-RIs was very diverse, RI specific, and developed over a long time from various user communities and applications. Presently, there is a controlled vocabulary extending beyond our RIs that has enabled better integration with other RIs and thematic domains.
The implementation of unique identifiers was crucial for cross-RI synergies and has led to the improved exchange of services, procedures, and data products. Also, an atmospheric community standard has evolved on the licence for data use. This has led to the reliable reuse of data. With a common use of licence now considered more a “community standard”, there is also possibility of producing new high-level cross-RI data products that utilise services and data across the atmosphere domain. The convergence of solutions across the atmosphere-centred RIs has led to new products that are useful for all users across the RIs and outside the atmosphere domain, e.g. satellite-borne instrument data extraction and colocation through machine-to-machine interoperable services relevant to the full atmosphere domain and beyond.
Science-based services are designed to facilitate data discoverability and accessibility for complex research questions and thus to support researchers focusing on scientific questions instead of searching for data. The following examples from the service portfolio of the ATMO-RIs are already accessible through the current demonstrator version of the ENVRI-Hub:
The atmospheric colocation service is a suite of tools to identify and retrieve space-borne observations that match ground-based measurements, given a list of ground-based stations and satellites together with user-specified colocation criteria. The service builds on the implementation of the ENVRI Python library for the dynamic retrieval of platform metadata for ACTRIS, ICOS, and SIOS stations and, in the case of IAGOS, for airports for which vertical profiles of atmospheric parameters are provided. A hierarchical catalogue structure for satellite-borne instrument data based on the AERIS/ICARE information system and enhanced with specific metadata is also implemented.
The ICOS Carbon Portal footprint tool provides atmospheric surface emission influence calculations (a.k.a., footprints) for any 3D location or specific station in the model domain and any period for which meteorological data are available. When ready, the results can be viewed in the tool and directly accessed through our Jupyter Notebook service and Python library, to be combined with emission data for concentration calculations at the receptor(s) and comparison with observations retrieved from the RI data repositories.
The FAIR ENVRI Atmospheric Data Demonstrator aims to shorten time responses in the case of extreme events like fires, emissions from volcanic eruption, and desert dust intrusion by providing scientific analyses as well as harmonised data sets and tools. It will offer a search tool for the ATMO-RIs' data availability for the period and area of interest with a focus on ECVs, fetch the selected data including automatic previews of the data sets, and provide statistical analysis on the downloaded time series. In addition, access to data from ATMO-RIs is offered as a bundle with automated compilation of provenance information, and a colocation service with satellite data is available.
The Dashboard for the State of the Environment is designed to be completely user configurable so that the users can select from a list of indicators to be shown and their order. Providers can add, remove, and edit indicators through a standard RESTful API that allows transferring all parameters, including the configuration of the indicators and how to provide data values and thumbnail interaction. The dashboard builds on EOSC services like AAI, cloud services, and data storage, and the workflows that provide the indicators will also build on the EOSC and research infrastructure computing integration. As a proof of concept, a limited list of indicators is currently available, and we foresee that the participating RIs will provide many more indicator options in the near future. The dashboard service is completely open-source, and, like the whole concept, it is designed to be flexible and expansible.
The science demonstrators are practical examples of the provision of interoperable services from the ATMO-RIs to the scientific community, enabling cross-RI data discovery and use. The software behind the science demonstrators can be accessed in GitHub repositories for further use and composition with users' own workflows. In that respect, the science demonstrators are showcases for new research opportunities evolving from a combination of RI data infrastructures with tools and methods of data-driven science.
Access to the data used in Fig. A2 is provided through Putaud and Martins dos Santos (2022a, b).
AP conceived and wrote the manuscript, and all co-authors contributed to their respective areas of expertise.
At least one of the (co-)authors is a member of the editorial board of Atmospheric Chemistry and Physics . The peer-review process was guided by an independent editor, and the authors also have no other competing interests to declare.
Publisher’s note: Copernicus Publications remains neutral with regard to jurisdictional claims made in the text, published maps, institutional affiliations, or any other geographical representation in this paper. While Copernicus Publications makes every effort to include appropriate place names, the final responsibility lies with the authors.
This article is part of the special issue “20 years of Atmospheric Chemistry and Physics”. It is not associated with a conference.
This paper is dedicated to the memory of our dear colleague Astrid Kiendler-Scharr, who passed away much too early in 2023. She was a big supporter of the concept of research infrastructures and recognised at a very early stage the important role that research infrastructures will play in today's complex research landscape. As a consequence, she served on the RI boards of ACTRIS and IAGOS and strongly promoted the use of RI data and services, particularly in work of the IPCC.
The European research infrastructures ACTRIS, IAGOS, and ICOS gratefully acknowledge continuous support by the European Strategy Forum on Research Infrastructures, ESFRI, and long-term funding by the European Union's Research and Innovation programmes through the cluster projects ENVRI (2011–2014, grant agreement 283465), ENVRIplus (2015–2019, grant agreement 654182), ENVRI-FAIR (2019–2023, grant agreement 824068), ATMO-ACCESS (started 2021, grant agreement 101008004), RI-URBANS (started 2021, grant agreement 101036245), ICOS Cities (started 2021, grant agreement 101037319), and EOSC Future (started 2021, grant agreement 101017536). Furthermore, all RIs gratefully acknowledge the support of the European Union's Research and Innovation programmes during the design and implementation phases of the individual RIs and the support of the implementation and operation by all national research ministries and funding agencies involved.
Finally, the authors would like to thank Oksana Tarasova and the anonymous reviewer for their constructive and supportive criticism, which helped to significantly improve the manuscript. They also would like to thank the executive and senior editors of Atmospheric Chemistry and Physics for the opportunity to contribute this opinion article to the special issue, “20 years of Atmospheric Chemistry and Physics”.
This research has been supported by the European Commission, EU Horizon 2020 Framework Programme (grant no. 824068). The article processing charges for this open-access publication were covered by the Forschungszentrum Jülich.
This paper was edited by Gabriele Stiller and James Allan and reviewed by Oksana Tarasova and one anonymous referee.
Atkinson, M., Gesing, S., Montagnat, J., and Taylor, I.: Scientific workflows: Past, present and future, Future Gener. Comput. Syst., 75, 216–227, https://doi.org/10.1016/j.future.2017.05.041 , 2017.
ATMO-ACCESS: Sustainable Access to Atmospheric Research Facilities, https://www.atmo-access.eu/ (last access: 21 March 2024), 2024.
Bailo, D.: Four-stages FAIR Roadmap – FAIR Pyramid, Zenodo, https://doi.org/10.5281/zenodo.3299353 , 2019.
Bauer, P., Dueben, P. D., Hoefler, T., Quintino, T., Schulthess, T. C., and Wedi, N. P.: The digital revolution of Earth-system science, Nat. Comput. Sci., 1, 104–113, https://doi.org/10.1038/s43588-021-00023-0 , 2021.
Bell, G., Hey, T., and Szalay, A.: Beyond the Data Deluge, Science, 323, 1297-1298, https://doi.org/10.1126/science.1170411 , 2009.
Belle, J. v., Barneveld-Biesma, J. v., Bastiaanssen, V., Buitenhuis, A., Saes, L., and Veen, G. v.: ICOS Impact Assessment Report, technopolis group, Amsterdam, 73 pp., https://www.icos-cp.eu/sites/default/files/2018-10/ICOS_Impact_Assessment_2018.pdf (last access: 30 April 2024), 2018.
Bojinski, S., Verstraete, M., Peterson, T. C., Richter, C., Simmons, A., and Zemp, M.: The concept of essential climate variables in support of climate research, applications and policy, B. Am. Meteorol. Soc., 95, 1431–1443, https://doi.org/10.1175/bams-d-13-00047.1 , 2014.
Bouwer, L. M., Dransch, D., Ruhnke, R., Rechid, D., Frickenhaus, S., and Greinert, J.: Integrating Data Science and Earth Science, 1 Ed., SpringerBriefs in Earth System Sciences, Springer Cham, eBook, XIV, 148 pp., https://doi.org/10.1007/978-3-030-99546-1 , 2022.
Brenninkmeijer, C. A. M., Crutzen, P. J., Fischer, H., Gusten, H., Hans, W., Heinrich, G., Heintzenberg, J., Hermann, M., Immelmann, T., Kersting, D., Maiss, M., Nolle, M., Pitscheider, A., Pohlkamp, H., Scharffe, D., Specht, K., and Wiedensohler, A.: CARIBIC – Civil aircraft for global measurement of trace gases and aerosols in the tropopause region, J. Ocean. Atmos. Technol., 16, 1373–1383, https://doi.org/10.1175/1520-0426(1999)016<1373:ccafgm>2.0.co;2 , 1999.
Carmichael, G. R., Tarasova, O., Hov, Ø., Barrie, L., and Butler, J. H.: Global Atmospheric Composition Observations: The Heart of Vital Climate and Environmental Action, B. Am. Meteorol. Soc., 104, E666–E672, https://doi.org/10.1175/BAMS-D-22-0016.1 , 2023.
Cerezo, N., Montagnat, J., and Blay-Fornarino, M.: Computer-Assisted Scientific Workflow Design, J. Comput., 11, 585–612, https://doi.org/10.1007/s10723-013-9264-5 , 2013.
Clark, H., Bennouna, Y., Tsivlidou, M., Wolff, P., Sauvage, B., Barret, B., Le Flochmoën, E., Blot, R., Boulanger, D., Cousin, J.-M., Nédélec, P., Petzold, A., and Thouret, V.: The effects of the COVID-19 lockdowns on the composition of the troposphere as seen by In-service Aircraft for a Global Observing System (IAGOS) at Frankfurt, Atmos. Chem. Phys., 21, 16237–16256, https://doi.org/10.5194/acp-21-16237-2021 , 2021.
Collaud Coen, M., Andrews, E., Alastuey, A., Arsov, T. P., Backman, J., Brem, B. T., Bukowiecki, N., Couret, C., Eleftheriadis, K., Flentje, H., Fiebig, M., Gysel-Beer, M., Hand, J. L., Hoffer, A., Hooda, R., Hueglin, C., Joubert, W., Keywood, M. , Kim, J. E., Kim, S.-W., Labuschagne, C., Lin, N.-H., Lin, Y., Lund Myhre, C., Luoma, K., Lyamani, H., Marinoni, A., Mayol-Bracero, O. L., Mihalopoulos, N., Pandolfi, M., Prats, N., Prenni, A. J., Putaud, J.-P., Ries, L., Reisen, F., Sellegri, K., Sharma, S., Sheridan, P., Sherman, J. P., Sun, J., Titos, G., Torres, E., Tuch, T., Weller, R., Wiedensohler, A., Zieger, P., and Laj, P.: Multidecadal trend analysis of in situ aerosol radiative properties around the world, Atmos. Chem. Phys., 20, 8867–8908, https://doi.org/10.5194/acp-20-8867-2020 , 2020.
Dai, Q., Shin, E., and Smith, C.: Open and inclusive collaboration in science, OECD Science, Technology and Industry Working Papers No 2018/04, OECD, Paris, France, 29 pp., https://doi.org/10.1787/2dbff737-en , 2018.
De Mazière, M., Thompson, A. M., Kurylo, M. J., Wild, J. D., Bernhard, G., Blumenstock, T., Braathen, G. O., Hannigan, J. W., Lambert, J.-C., Leblanc, T., McGee, T. J., Nedoluha, G., Petropavlovskikh, I., Seckmeyer, G., Simon, P. C., Steinbrecht, W., and Strahan, S. E.: The Network for the Detection of Atmospheric Composition Change (NDACC): history, status and perspectives, Atmos. Chem. Phys., 18, 4935–4964, https://doi.org/10.5194/acp-18-4935-2018 , 2018.
EC: REGULATION (EU) No 1291/2013 OF THE EUROPEAN PARLIAMENT AND OF THE COUNCIL establishing Horizon 2020 – the Framework Programme for Research and Innovation (2014–2020) and repealing Decision No 1982/2006/EC, European Commission, Brussels, Belgium, 11 December 2013, 173 pp., https://eur-lex.europa.eu/LexUriServ/LexUriServ.do?uri=OJ:L:2013:347:0104:0173:EN:PDF (last access: 30 April 2024), 2013.
EC: EOSC Declaration, European Commission, Brussels, Ref. Ares(2017)3488418 – 11/07/2017, 9 pp., https://eosc-portal.eu/sites/default/files/eosc_declaration.pdf (last access: 30 April 2024), 2017.
ENVRI: Environmental Research Infrastructures, https://envri.eu/ (last access: 21 March 2024), 2024.
ENVRI-Community: ENVRI-Community at Zenodo, https://zenodo.org/communities/envri/ (last access: 21 March 2024), 2024.
ENVRI-Hub: ENVRI-Hub Demonstrator, https://envri-hub.envri.eu/ (last access: 21 March 2024), 2024.
ESFRI: Landscape Analysis – Environment, in: Strategy Report on Research Infrasructures – Roadmap 2021, edited by: Euopean Strategy Forum for Research Infrastructures, Brussels, Belgium, 62–75, https://roadmap2021.esfri.eu/landscape-analysis/section-1/environment/ (last access: 30 April 2024), 2021a.
ESFRI: Strategy Report on Research Infrasructures – Roadmap 2021, Euopean Strategy Forum for Research Infrastructures, Brussels, Belgium, 243 pp., https://roadmap2021.esfri.eu/ (last access: 30 April 2024), 2021b.
Evangeliou, N., Platt, S. M., Eckhardt, S., Lund Myhre, C., Laj, P., Alados-Arboledas, L., Backman, J., Brem, B. T., Fiebig, M., Flentje, H., Marinoni, A., Pandolfi, M., Yus-Dìez, J., Prats, N., Putaud, J. P., Sellegri, K., Sorribas, M., Eleftheriadis, K., Vratolis, S., Wiedensohler, A., and Stohl, A.: Changes in black carbon emissions over Europe due to COVID-19 lockdowns, Atmos. Chem. Phys., 21, 2675–2692, https://doi.org/10.5194/acp-21-2675-2021 , 2021.
Farshidi, S., Liao, X., Li, N., Goldfarb, D., Magagna, B., Stocker, M., Jeffery, K., Thijsse, P., Pichot, C., Petzold, A., and Zhao, Z.: Knowledge sharing and discovery across heterogeneous research infrastructures, Open Res. Europe, 1, 68, https://doi.org/10.12688/openreseurope.13677.2 , 2021.
Farshidi, S. and Zhao, Z.: An Adaptable Indexing Pipeline for Enriching Meta Information of Datasets from Heterogeneous Repositories, Advances in Knowledge Discovery and Data Mining, Cham, 2022, Springer International Publishing, 472–484, https://link.springer.com/chapter/10.1007/978-3-031-05936-0_37 (last access: 30 April 2024), 2022.
Franz, D., Acosta, M., Altimir, N., Arriga, N., Arrouays, D., Aubinet, M., Aurela, M., Ayres, E., Lopez-Ballesteros, A., Barbaste, M., Berveiller, D., Biraud, S., Boukir, H., Brown, T., Brummer, C., Buchmann, N., Burba, G., Carrara, A., Cescatti, A., Ceschia, E., Clement, R., Cremonese, E., Crill, P., Darenova, E., Dengel, S., D'Odorico, P., Filippa, G., Fleck, S., Fratini, G., Fuss, R., Gielen, B., Gogo, S., Grace, J., Graf, A., Grelle, A., Gross, P., Grunwald, T., Haapanala, S., Hehn, M., Heinesch, B., Heiskanen, J., Herbst, M., Herschlein, C., Hortnagl, L., Hufkens, K., Ibrom, A., Jolivet, C., Joly, L., Jones, M., Kiese, R., Klemedtsson, L., Kljun, N., Klumpp, K., Kolari, P., Kolle, O., Kowalski, A., Kutsch, W., Laurila, T., de Ligne, A., Linder, S., Lindroth, A., Lohila, A., Longdoz, B., Mammarella, I., Manise, T., Jimenez, S. M., Matteucci, G., Mauder, M., Meier, P., Merbold, L., Mereu, S., Metzger, S., Migliavacca, M., Molder, M., Montagnani, L., Moureaux, C., Nelson, D., Nemitz, E., Nicolini, G., Nilsson, M. B., Op de Beeck, M., Osborne, B., Lofvenius, M. O., Pavelka, M., Peichl, M., Peltola, O., Pihlatie, M., Pitacco, A., Pokorny, R., Pumpanen, J., Ratie, C., Rebmann, C., Roland, M., Sabbatini, S., Saby, N. P. A., Saunders, M., Schmid, H. P., Schrumpf, M., Sedlak, P., Ortiz, P. S., Siebicke, L., Sigut, L., Silvennoinen, H., Simioni, G., Skiba, U., Sonnentag, O., Soudani, K., Soule, P., Steinbrecher, R., Tallec, T., Thimonier, A., Tuittila, E. S., Tuovinen, J. P., Vestin, P., Vincent, G., Vincke, C., Vitale, D., Waldner, P., Weslien, P., Wingate, L., Wohlfahrt, G., Zahniser, M., and Vesala, T.: Towards long-term standardised carbon and greenhouse gas observations for monitoring Europe's terrestrial ecosystems: a review, Int. Agrophys., 32, 439–455, https://doi.org/10.1515/intag-2017-0039 , 2018.
Gaudel, A., Cooper, O. R., Ancellet, G., Barret, B., Boynard, A., Burrows, J. P., Clerbaux, C., Coheur, P. F., Cuesta, J., Cuevas, E., Doniki, S., Dufour, G., Ebojie, F., Foret, G., Garcia, O., Granados-Munoz, M. J., Hannigan, J. W., Hase, F., Hassler, B., Huang, G., Hurtmans, D., Jaffe, D., Jones, N., Kalabokas, P., Kerridge, B., Kulawik, S., Latter, B., Leblanc, T., Le Flochmoen, E., Lin, W., Liu, J., Liu, X., Mahieu, E., McClure-Begley, A., Neu, J. L., Osman, M., Palm, M., Petetin, H., Petropavlovskikh, I., Querel, R., Rahpoe, N., Rozanov, A., Schultz, M. G., Schwab, J., Siddans, R., Smale, D., Steinbacher, M., Tanimoto, H., Tarasick, D. W., Thouret, V., Thompson, A. M., Trickl, T., Weatherhead, E., Wespes, C., Worden, H. M., Vigouroux, C., Xu, X., Zeng, G., and Ziemke, J.: Tropospheric Ozone Assessment Report: Present-day distribution and trends of tropospheric ozone relevant to climate and global atmospheric chemistry model evaluation, Elementa-Sci. Anthrop., 6, 58, https://doi.org/10.1525/elementa.291 , 2018.
Gaudel, A., Cooper, O. R., Chang, K. L., Bourgeois, I., Ziemke, J. R., Strode, S. A., Oman, L. D., Sellitto, P., Nedelec, P., Blot, R., Thouret, V., and Granier, C.: Aircraft observations since the 1990s reveal increases of tropospheric ozone at multipl e locations across the Northern Hemisphere, Sci. Adv., 6, 11, https://doi.org/10.1126/sciadv.aba8272 , 2020.
GCOS: Implementation Plan for the Global Observing System for Climate in Support of the UNFCCC, World Meteorological Organization, Geneva, Switzerland, Report GCOS-138, 186 pp., https://library.wmo.int/viewer/58703/?offset=#page=1&viewer=picture&o=bookmark&n=0&q= (last access: 30 April 2024), 2010.
GCOS: The Global Observing System for Climate: Implementation Needs, World Meteorological Organization, Geneva, Switzerland, Report GCOS-200, 315 pp., https://library.wmo.int/viewer/55469?medianame=GCOS-200_OnlineVersion_#page=1&viewer=picture&o=bookmark&n=0&q= (last access: 30 April 2024), 2016.
GCOS: The 2022 GCOS ECVs Requirements, World Meteorological Organization, Geneva, Switzerland, Report GCOS-245, 244 pp., https://library.wmo.int/records/item/58111-the-2022-gcos-ecvs-requirements-gcos-245 (last access: 30 April 2024), 2022a.
GCOS: The 2022 GCOS Implementation Plan, World Meteorological Organization, Geneva, Switzerland, Report GCOS-244, 85 pp., https://gcos.wmo.int/en/publications/gcos-implementation-plan2022 (last access: 30 April 2024), 2022b.
Gkatzelis, G. I., Gilman, J. B., Brown, S. S., Eskes, H., Gomes, A. R., Lange, A. C., McDonald, B. C., Peischl, J., Petzold, A., Thompson, C. R., and Kiendler-Scharr, A.: The global impacts of COVID-19 lockdowns on urban air pollution: A critical review and recommendations, Elementa-Sci. Anthrop., 9, 00176, https://doi.org/10.1525/elementa.2021.00176 , 2021.
Gray, J.: Jim Gray on eScience: A Transformed Scientific Method, in: The Fourth Paradigm: Data-Intensive Scientific Discovery, edited by: Hey, T., Tansley, S., and Tolle, K., Microsoft Research, Redmond, Washington, USA, xvii–xxxi, https://www.microsoft.com/en-us/research/wp-content/uploads/2009/10/Fourth_Paradigm.pdf (last access: 30 April 2024), 2009.
Heiskanen, J., Brümmer, C., Buchmann, N., Calfapietra, C., Chen, H., Gielen, B., Gkritzalis, T., Hammer, S., Hartman, S., Herbst, M., Janssens, I. A., Jordan, A., Juurola, E., Karstens, U., Kasurinen, V., Kruijt, B., Lankreijer, H., Levin, I., Linderson, M.-L., Loustau, D., Merbold, L., Myhre, C. L., Papale, D., Pavelka, M., Pilegaard, K., Ramonet, M., Rebmann, C., Rinne, J., Rivier, L., Saltikoff, E., Sanders, R., Steinbacher, M., Steinhoff, T., Watson, A., Vermeulen, A. T., Vesala, T., Vítková, G., and Kutsch, W.: The Integrated Carbon Observation System in Europe, B. Am. Meteorol. Soc., 103, E855–E872, https://doi.org/10.1175/BAMS-D-19-0364.1 , 2022.
Hidalga, A. N. d. l., Hardisty, A., Martin, P., Magagna, B., and Zhao, Z.: The ENVRI Reference Model, in: Towards Interoperable Research Infrastructures for Environmental and Earth Sciences: A Reference Model Guided Approach for Common Challenges, edited by: Zhao, Z., and Hellström, M., Springer International Publishing, Cham, 61–81, https://doi.org/10.1007/978-3-030-52829-4_1 , 2020.
Holben, B. N., Eck, T. F., Slutsker, I., Tanre, D., Buis, J. P., Setzer, A., Vermote, E., Reagan, J. A., Kaufman, Y. J., Nakajima, T., Lavenu, F., Jankowiak, I., and Smirnov, A.: AERONET – A federated instrument network and data archive for aerosol characterization, Remote Sens. Environ., 66, 1–16, doi: https://doi.org/10.1016/s0034-4257(98)00031-5 , 1998.
ICOS-Cities: ICOS-Cities, https://www.icos-cp.eu/projects/icos-cities (last access: 21 March 2024), 2024.
IPCC: Climate Change 2021: The Physical Science Basis. Contribution of Working Group I to the Sixth Assessment Report of the Intergovernmental Panel on Climate Change, edited by: Masson-Delmotte, V., Zhai, P., Pirani, A., Connors, S. L., Péan, C., Berger, S., Caud, N., Chen, Y., Goldfarb, L., Gomis, M. I., Huang, M., Leitzell, K., Lonnoy, E., Matthews, J. B. R., Maycock, T. K., Waterfield, T., Yelekçi, O., Yu, R., and Zhou, B., Cambridge University Press, Cambridge, United Kingdom and New York, NY, USA, https://doi.org/10.1017/9781009157896 , 2021a.
IPCC: Summary for Policymakers, in: Climate Change 2021 – The Physical Science Basis: Working Group I Contribution to the Sixth Assessment Report of the Intergovernmental Panel on Climate Change, edited by: Masson-Delmotte, V., Zhai, P., Pirani, A., Connors, S. L., Péan, C., Berger, S., Caud, N., Chen, Y., Goldfarb, L., Gomis, M. I., Huang, M., Leitzell, K., Lonnoy, E., Matthews, J. B. R., Maycock, T. K., Waterfield, T., Yelekçi, O., Yu, R., and Zhou, B., Cambridge University Press, Cambridge, 3-32, https://doi.org/10.1017/9781009157896.001 , 2021b.
IPCC: Climate Change 2023: Synthesis Report. Contribution of Working Groups I, II and III to the Sixth Assessment Report of the Intergovernmental Panel on Climate Change edited by: Core Writing Team, Lee, H., and Romero, J., IPCC, Geneva, 184 pp., https://doi.org/10.59327/IPCC/AR6-9789291691647 , 2023.
Kulmala, M.: Build a global Earth observatory, Nature, 553, 21–23, https://doi.org/10.1038/d41586-017-08967-y , 2018.
Kulmala, M., Kokkonen, T., Ezhova, E., Baklanov, A., Mahura, A., Mammarella, I., Back, J., Lappalainen, H. K., Tyuryakov, S., Kerminen, V. M., Zilitinkevich, S., and Petaja, T.: Aerosols, Clusters, Greenhouse Gases, Trace Gases and Boundary-Layer Dynamics: on Feedbacks and Interactions, Bound.-Layer Meteor., 186, 475–503, https://doi.org/10.1007/s10546-022-00769-8 , 2023a.
Kulmala, M., Lintunen, A., Lappalainen, H., Virtanen, A., Yan, C., Ezhova, E., Nieminen, T., Riipinen, I., Makkonen, R., Tamminen, J., Sundström, A.-M., Arola, A., Hansel, A., Lehtinen, K., Vesala, T., Petäjä, T., Bäck, J., Kokkonen, T., and Kerminen, V.-M.: Opinion: The strength of long-term comprehensive observations to meet multiple grand challenges in different environments and in the atmosphere, Atmos. Chem. Phys., 23, 14949–14971, https://doi.org/10.5194/acp-23-14949-2023 , 2023b.
Laj, P., Bigi, A., Rose, C., Andrews, E., Lund Myhre, C., Collaud Coen, M., Lin, Y., Wiedensohler, A., Schulz, M., Ogren, J. A., Fiebig, M., Gliß, J., Mortier, A., Pandolfi, M., Petäja, T., Kim, S.-W., Aas, W., Putaud, J.-P., Mayol-Bracero, O., Keywood, M., Labrador, L., Aalto, P., Ahlberg, E., Alados Arboledas, L., Alastuey, A., Andrade, M., Artíñano, B., Ausmeel, S., Arsov, T., Asmi, E., Backman, J., Baltensperger, U., Bastian, S., Bath, O., Beukes, J. P., Brem, B. T., Bukowiecki, N., Conil, S., Couret, C., Day, D., Dayantolis, W., Degorska, A., Eleftheriadis, K., Fetfatzis, P., Favez, O., Flentje, H., Gini, M. I., Gregorič, A., Gysel-Beer, M., Hallar, A. G., Hand, J., Hoffer, A., Hueglin, C., Hooda, R. K., Hyvärinen, A., Kalapov, I., Kalivitis, N., Kasper-Giebl, A., Kim, J. E., Kouvarakis, G., Kranjc, I., Krejci, R., Kulmala , M., Labuschagne, C., Lee, H.-J., Lihavainen, H., Lin, N.-H., Löschau, G., Luoma, K., Marinoni, A., Martins Dos Santos, S., Meinhardt, F., Merkel, M., Metzger, J.-M., Mihalopoulos, N., Nguyen, N. A., Ondracek, J., Pérez, N., Perrone, M. R., Petit, J.-E., Picard, D., Pichon, J.-M., Pont, V., Prats, N., Prenni, A., Reisen, F., Romano, S., Sellegri, K., Sharma, S., Schauer, G., Sheridan, P., Sherman, J. P., Schütze, M., Schwerin, A., Sohmer, R., Sorribas, M., Steinbacher, M., Sun, J., Titos, G., Toczko, B., Tuch, T., Tulet, P., Tunved, P., Vakkari, V., Velarde, F., Velasquez, P., Villani, P., Vratolis, S., Wang, S.-H., Weinhold, K., Weller, R., Yela, M., Yus-Diez, J., Zdimal, V., Zieger, P., and Zikova, N.: A global analysis of climate-relevant aerosol properties retrieved from the network of Global Atmosphere Watch (GAW) near-surface observatories, Atmos. Meas. Tech., 13, 4353–4392, https://doi.org/10.5194/amt-13-4353-2020 , 2020.
Laj, P., Lund Myhre, C., Riffault, V., Amiridis, V., Fuchs, H., Eleftheriadis, E., Petäjä, T., Kivekäs, N., Juurola, E., Saponaro, G., Philippin, S., Cornacchia, C., Alados Arboledas, L., Baars, H., Claude, A., De Mazière, M., Dils, B., Murberg, L.-E., Fiebig, M., Haeffelin, M., Herrmann, H., Höhler, K., Illmann, N., Kreuter, A., Ludewig, E., Marinou, E., Möhler, O., Mona, L., Nicolae, D., O'Connor, E., Petracca Altieri, R.-M., Picquet-Varrault, B., Pospichal, B., Putaud, J.-P., Reimann, S., Salameh, T., Siomos, N., Stachlewska, I., van Pinxteren, D., Voudouri, K.-A., Wandinger, U., Wiedensohler, A., Apituley, A., Comerón, A., Gysel-Beer, M., Mihalopoulos, N., Nikolova, N., Pietruczuk, A., Sauvage, S., Sciare, J., Skov, S., Svendby, T., Swietlicki, E., Tonev, D., Vaughan, G., Zdimal, V., Baltensperger, U., Doussin, J.-F., Kulmala, M., Pappalardo, G., Sorvari Sundet, S., and Vana, M.: Aerosol, Clouds and Trace Gases Research Infrastructure – ACTRIS, the European research infrastructure supporting atmospheric science, B. Am. Meteor. Soc., in review, 2024.
Lynch, C. A.: Jim Gray's Fourth Paradigm and the Construction of the Scientific Record, in: The Fourth Paradigm: Data-Intensive Scientific Discovery, edited by: Hey, T., Tansley, S., and Tolle, K., Microsoft Research, Redmond, Washington, USA, 177–183, https://www.microsoft.com/en-us/research/wp-content/uploads/2009/10/Fourth_Paradigm.pdf (last access: 30 April 2024), 2009.
Marenco, A., Thouret, V., Nédélec, P., Smit, H., Helten, M., Kley, D., Karcher, F., Simon, P., Law, K., Pyle, J., Poschmann, G., Von Wrede, R., Hume, C., and Cook, T.: Measurement of ozone and water vapor by Airbus in-service aircraft: The MOZAIC airborne program, An overview, J. Geophys. Res., 103, 25631–25642, https://doi.org/10.1029/98jd00977 , 1998.
Petetin, H., Thouret, V., Fontaine, A., Sauvage, B., Athier, G., Blot, R., Boulanger, D., Cousin, J.-M., and Nédélec, P.: Characterising tropospheric O3 and CO around Frankfurt over the period 1994–2012 based on MOZAIC–IAGOS aircraft measurements, Atmos. Chem. Phys., 16, 15147–15163, https://doi.org/10.5194/acp-16-15147-2016 , 2016.
Petetin, H., Jeoffrion, M., Sauvage, B., Athier, G., Blot, R., Boulanger, D., Clark, H., Cousin, J.-M., Gheusi, F., Nedelec, P., Steinbacher, M., and Thouret, V.: Representativeness of the IAGOS airborne measurements in the lower troposphere, Elementa-Sci. Anthrop., 6, 23, https://doi.org/10.1525/elementa.280 , 2018.
Petzold, A., Thouret, V., Gerbig, C., Zahn, A., Brenninkmeijer, C. A. M., Gallagher, M., Hermann, M., Pontaud, M., Ziereis, H., Boulanger, D., Marshall, J., Nédélec, P., Smit, H. G. J., Frieß, U., Flaud, J.-M., Wahner, A., Cammas, J.-P., Volz-Thomas, A., and IAGOS-Team: Global-Scale Atmosphere Monitoring by In-Service Aircraft – Current Achievements and Future Prospects of the European Research Infrastructure IAGOS, Tellus B, 67, 28452, https://doi.org/10.3402/tellusb.v67.28452 , 2015.
Petzold, A., Asmi, A., Vermeulen, A., Pappalardo, G., Bailo, D., Schaap, D., Glaves, H. M., Bundke, U., and Zhao, Z.: ENVRI-FAIR - Interoperable environmental FAIR data and services for society, innovation and research, 15th IEEE International Conference on eScience 2019, 277–280, https://doi.org/10.1109/eScience.2019.00038 , 2019.
Petzold, A., Neis, P., Rütimann, M., Rohs, S., Berkes, F., Smit, H. G. J., Krämer, M., Spelten, N., Spichtinger, P., Nédélec, P., and Wahner, A.: Ice-supersaturated air masses in the northern mid-latitudes from regular in situ observations by passenger aircraft: vertical distribution, seasonality and tropospheric fingerprint, Atmos. Chem. Phys., 20, 8157–8179, https://doi.org/10.5194/acp-20-8157-2020 , 2020.
Petzold, A., Gomes, A. R., Bundke, U., Schleiermacher, C., Adamaki, A., Vermeulen, A., Zhao, Z., Stocker, M., Lund Myhre, C., Boulanger, D., Hienola, A., and Bailo, D.: ENVRI-Hub Design and Architecture White Paper (Version 1), Zenodo, 21 pp., https://zenodo.org/record/8046894 (last access: 30 April 2024), 2023.
Peuch, V. H., Engelen, R., Rixen, M., Dee, D., Flemming, J., Suttie, M., Ades, M., Agusti-Panareda, A., Ananasso, C., Andersson, E., Armstrong, D., Barre, J., Bousserez, N., Dominguez, J. J., Garrigues, S., Inness, A., Jones, L., Kipling, Z., Letertre-Danczak, J., Parrington, M., Razinger, M., Ribas, R., Vermoote, S., Yang, X. B., Simmons, A., de Marcilla, J. G., and Thepaut, J. N.: The Copernicus Atmosphere Monitoring Service: From Research to Operations, B. Am. Meteorol. Soc., 103, E2650–E2668, https://doi.org/10.1175/bams-d-21-0314.1 , 2022.
Putaud, J. P. and Martins dos Santos, S.: ACTRIS, EMEP, GAW-WDCA, 2008–2022: Aerosol particle absorption coefficient observed by filter absorption photometer at Ispra, data hosted by EBAS at NILU, https://ebas-data.nilu.no/DataSets.aspx?stations=IT0004R&projects=ACTRIS&InstrumentTypes=filter_absorption_photometer&fromDate=1970-01-01&toDate=2023-12-31 (last access: 30 April 2024), 2022a.
Putaud, J. P. and Martins dos Santos, S.: ACTRIS, EMEP, GAW-WDCA, 2004–2022: Aerosol particle scattering coefficient observed by nephelometer at Ispra, data hosted by EBAS at NILU, https://ebas-data.nilu.no/DataSets.aspx?stations=IT0004R&projects=ACTRIS&InstrumentTypes=nephelometer&fromDate=1970-01-01&toDate=2023-12-31 (last access: 30 April 2024), 2022b.
RI-URBANS: Research Infrastructures Services Reinforcing Air Quality Monitoring Capacities in European Urban & Industrial AreaS, https://riurbans.eu/ (last access: 21 March 2024), 2024.
Romanello, M., Napoli, C. D., Drummond, P., Green, C., Kennard, H., Lampard, P., Scamman, D., Arnell, N., Ayeb-Karlsson, S., Ford, L. B., Belesova, K., Bowen, K., Cai, W., Callaghan, M., Campbell-Lendrum, D., Chambers, J., Daalen, K. R. v., Dalin, C., Dasandi, N., Dasgupta, S., Davies, M., Dominguez-Salas, P., Dubrow, R., Ebi, K. L., Eckelman, M., Ekins, P., Escobar, L. E., Georgeson, L., Graham, H., Gunther, S. H., Hamilton, I., Hang, Y., Hänninen, R., Hartinger, S., He, K., Hess, J. J., Hsu, S.-C. , Jankin, S., Jamart, L., Jay, O., Kelman, I., Kiesewetter, G., Kinney, P., Kjellstrom, T., Kniveton, D., Lee, J. K. W., Lemke, B., Liu, Y., Liu, Z., Lott, M., Batista, M. L., Lowe, R., MacGuire, F., Sewe, M. O., Martinez-Urtaza, J., Maslin, M., McAllister, L., McGushin, A., McMichael, C., Mi, Z., Milner, J., Minor, K., Minx, J. C., Mohajeri, N., Moradi-Lakeh, M., Morrissey, K., Munzert, S., Murray, K. A., Neville, T., Nilsson, M., Obradovich, N., O'Hare, M. B., Oreszczyn, T., Otto, M., Owfi, F., Pearman, O., Rabbaniha, M., Robinson, E. J. Z., Rocklöv, J., Salas, R. N., Semenza, J. C., Sherman, J. D., Shi, L., Shumake-Guillemot, J., Silbert, G., Sofiev, M., Springmann, M., Stowell, J., Tabatabaei, M., Taylor, J., Triñanes, J., Wagner, F., Wilkinson, P., Winning, M., Yglesias-González, M., Zhang, S., Gong, P., Montgomery, H., and Costello, A.: The 2022 report of the Lancet Countdown on health and climate change: health at the mercy of fossil fuels, Lancet, 400, 1619–1654, https://doi.org/10.1016/S0140-6736(22)01540-9 , 2022.
Schulthess, T. C.: Programming revisited, Nat. Phys., 11, 369–373, https://doi.org/10.1038/nphys3294 , 2015.
Schultz, M. G., Schroder, S., Lyapina, O., Cooper, O. R., Galbally, I., Petropavlovskikh, I., von Schneidemesser, E., Tanimoto, H., Elshorbany, Y., Naja, M., Seguel, R. J., Dauert, U., Eckhardt, P., Feigenspan, S., Fiebig, M., Hjellbrekke, A. G., Hong, Y. D., Kjeld, P. C., Koide, H., Lear, G., Tarasick, D., Ueno, M., Wallasch, M., Baumgardner, D., Chuang, M. T., Gillett, R., Lee, M., Molloy, S., Moolla, R., Wang, T., Sharps, K., Adame, J. A., Ancellet, G., Apadula, F., Artaxo, P., Barlasina, M. E., Bogucka, M., Bonasoni, P., Chang, L., Colomb, A., Cuevas-Agullo, E., Cupeiro, M., Degorska, A., Ding, A. J., FrHlich, M., Frolova, M., Gadhavi, H., Gheusi, F., Gilge, S., Gonzalez, M. Y., Gros, V., Hamad, S. H., Helmig, D., Henriques, D., Hermansen, O., Holla, R., Hueber, J., Im, U., Jaffe, D. A., Komala, N., Kubistin, D., Lam, K. S., Laurila, T., Lee, H., Levy, I., Mazzoleni, C., Mazzoleni, L. R., McClure-Begley, A., Mohamad, M., Murovec, M., Navarro-Comas, M., Nicodim, F., Parrish, D., Read, K. A., Reid, N., Ries, N. R. L., Saxena, P., Schwab, J. J., Scorgie, Y., Senik, I., Simmonds, P., Sinha, V., Skorokhod, A. I., Spain, G., Spangl, W., Spoor, R., Springston, S. R., Steer, K., Steinbacher, M., Suharguniyawan, E., Torre, P., Trickl, T., Lin, W. L., Weller, R., Xu, X. B., Xue, L. K., and Ma, Z. Q.: Tropospheric Ozone Assessment Report: Database and metrics data of global surface ozone observations, Elementa-Sci. Anthrop., 5, 26, https://doi.org/10.1525/elementa.244 , 2017.
SDG: United Nations Sustainable Development Goals, https://sdgs.un.org/goals (last access: 21 March 2024), 2024.
Sokhi, R. S., Singh, V., Querol, X., Finardi, S., Targino, A. C., Andrade, M. d. F., Pavlovic, R., Garland, R. M., Massagué, J., Kong, S., Baklanov, A., Ren, L., Tarasova, O., Carmichael, G., Peuch, V.-H., Anand, V., Arbilla, G., Badali, K., Beig, G., Belalcazar, L. C., Bolignano, A., Brimblecombe, P., Camacho, P., Casallas, A., Charland, J.-P., Choi, J., Chourdakis, E., Coll, I., Collins, M., Cyrys, J., da Silva, C. M., Di Giosa, A. D., Di Leo, A., Ferro, C., Gavidia-Calderon, M., Gayen, A., Ginzburg, A., Godefroy, F., Gonzalez, Y. A., Guevara-Luna, M., Haque, S. M., Havenga, H., Herod, D., Hõrrak, U., Hussein, T., Ibarra, S., Jaimes, M., Kaasik, M., Khaiwal, R., Kim, J., Kousa, A., Kukkonen, J., Kulmala, M., Kuula, J., La Violette, N., Lanzani, G., Liu, X., MacDougall, S., Manseau, P. M., Marchegiani, G., McDonald, B., Mishra, S. V., Molina, L. T., Mooibroek, D., Mor, S., Moussiopoulos, N., Murena, F., Niemi, J. V., Noe, S., Nogueira, T., Norman, M., Pérez-Camaño, J. L., Petäjä, T., Piketh, S., Rathod, A., Reid, K., Retama, A., Rivera, O., Rojas, N. Y., Rojas-Quincho, J. P., San José, R., Sánchez, O., Seguel, R. J., Sillanpää, S., Su, Y., Tapper, N., Terrazas, A., Timonen, H., Toscano, D., Tsegas, G., Velders, G. J. M., Vlachokostas, C., von Schneidemesser, E., Vpm, R., Yadav, R., Zalakeviciute, R., and Zavala, M.: A global observational analysis to understand changes in air quality during exceptionally low anthropogenic emission conditions, Env. Int., 157, 106818, https://doi.org/10.1016/j.envint.2021.106818 , 2021.
Steffen, W., Grinevald, J., Crutzen, P., and McNeill, J.: The Anthropocene: conceptual and historical perspectives, Philos. Trans. R. Soc. A, 369, 842–867, https://doi.org/10.1098/rsta.2010.0327 , 2011.
Stocker, M.: Advancing the Software Systems of Environmental Knowledge Infrastructures, in: Terrestrial Ecosystem Research Infrastructures: Challenges and Opportunities, edited by: Chabbi, A. and Loescher, H. W., CRC Press, Taylor & Francis Group, 399–423, https://doi.org/10.1201/9781315368252-19 , 2017.
Stocker, M., Oelen, A., Jaradeh, M. Y., Haris, M., Oghli, O. A., Heidari, G., Hussein, H., Lorenz, A.-L., Kabenamualu, S., Farfar, K. E., Prinz, M., Karras, O., D'Souza, J., Vogt, L., and Auer, S.: FAIR scientific information with the Open-Research Knowledge Graph, FAIR Connect, 1, 19–21, https://doi.org/10.3233/FC-221513 , 2023.
Tarasick, D., Galbally, I. E., Cooper, O. R., Schultz, M. G., Ancellet, G., Leblanc, T., Wallington, T. J., Ziemke, J., Liu, X., Steinbacher, M., Staehelin, J., Vigouroux, C., Hannigan, J. W., Garcia, O., Foret, G., Zanis, P., Weatherhead, E., Petropavlovskikh, I., Worden, H., Osman, M., Liu, J., Chang, K. L., Gaudel, A., Lin, M. Y., Granados-Munoz, M., Thompson, A. M., Oltmans, S. J., Cuesta, J., Dufour, G., Thouret, V., Hassler, B., Trickl, T., and Neu, J. L.: Tropospheric Ozone Assessment Report: Tropospheric ozone from 1877 to 2016, observed levels, trends and uncertainties, Elementa-Sci. Anthrop., 7, 72 pp., https://doi.org/10.1525/elementa.376 , 2019.
Tarasick, D. W., Smit, H. G. J., Thompson, A. M., Morris, G. A., Witte, J. C., Davies, J., Nakano, T., Van Malderen, R., Stauffer, R. M., Johnson, B. J., Stübi, R., Oltmans, S. J., and Vömel, H.: Improving ECC Ozonesonde Data Quality: Assessment of Current Methods and Outstanding Issues, Earth Space Sci., 8, e2019EA000914, https://doi.org/10.1029/2019EA000914 , 2021.
The Lancet Planetary, H.: A tale of two emergencies, Lancet, 4, e86, https://doi.org/10.1016/S2542-5196(20)30062-0 , 2020.
Thepaut, J. N., Pinty, B., Dee, D., and Engelen, R.: The Copernicus Programme and its Climate Change Service, in: IEEE International Symposium on Geoscience and Remote Sensing IGARSS, 38th IEEE International Geoscience and Remote Sensing Symposium (IGARSS), Valencia, SPAIN, 2018, IEEE, WOS:000451039801193, 1591–1593, 2018.
Thouret, V., Clark, H., Petzold, A., Nédélec, P., and Zahn, A.: IAGOS: Monitoring Atmospheric Composition for Air Quality and Climate by Passenger Aircraft, in: Handbook of Air Quality and Climate Change, edited by: Akimoto, H., and Tanimoto, H., Springer Nature Singapore, Singapore, 1–14, https://doi.org/10.1007/978-981-15-2527-8_57-1 , 2022.
Trewin, B., Cazenave, A., Howell, S., Huss, M., Isensee, K., Palmer, M. D., Tarasova, O., and Vermeulen, A.: Headline Indicators fo r Global Climate Monitoring, B. Am. Meteorol. Soc., 102, E20–E37, https://doi.org/10.1175/bams-d-19-0196.1 , 2021.
Vermeulen, A., Glaves, H., Pouliquen, S., and Kokkinaki, A.: Supporting Cross-Domain System-Level Environmental and Earth Science, in: Towards Interoperable Research Infrastructures for Environmental and Earth Sciences: A Reference Model Guided Approach for Common Challenges, edited by: Zhao, Z. and Hellström, M., Springer International Publishing, Cham, 3–16, https://doi.org/10.1007/978-3-030-52829-4_1 , 2020.
von Schneidemesser, E., Monks, P. S., Allan, J. D., Bruhwiler, L., Forster, P., Fowler, D., Lauer, A., Morgan, W. T., Paasonen, P., Righi, M., Sindelarova, K., and Sutton, M. A.: Chemistry and the Linkages between Air Quality and Climate Change, Chem. Rev., 115, 3856–3897, https://doi.org/10.1021/acs.chemrev.5b00089 , 2015.
Wagner, A., Bennouna, Y., Blechschmidt, A.-M., Brasseur, G., Chabrillat, S., Christophe, Y., Errera, Q., Eskes, H., Flemming, J., Hansen, K. M., Inness, A., Kapsomenakis, J., Langerock, B., Richter, A., Sudarchikova, N., Thouret, V., and Zerefos, C.: Comprehensive evaluation of the Copernicus Atmosphere Monitoring Service (CAMS) reanalysis against independent observations: Reactive gases, Elementa-Sci. Anthrop., 9, 00171, https://doi.org/10.1525/elementa.2020.00171 , 2021.
WCRP: World Climate Research Programme, https://www.wcrp-climate.org/ (last access: 21 March 2024), 2024.
Weatherhead, E. C., Wielicki, B. A., Ramaswamy, V., Abbott, M., Ackerman, T. P., Atlas, R., Brasseur, G., Bruhwiler, L., Busalacchi, A. J., Butler, J. H., Clack, C. T. M., Cooke, R., Cucurull, L., Davis, S. M., English, J. M., Fahey, D. W., Fine, S. S., Lazo, J. K., Liang, S. L., Loeb, N. G., Rignot, E., Soden, B., Stanitski, D., Stephens, G., Tapley, B. D., Thompson, A. M., Trenberth, K. E., and Wuebbles, D.: Designing the Climate Observing System of the Future, Earth Future, 6, 80–102, https://doi.org/10.1002/2017ef000627 , 2018.
Wilkinson, M. D., Dumontier, M., Aalbersberg, I. J., Appleton, G., Axton, M., Baak, A., Blomberg, N., Boiten, J.-W., da Silva Santos, L. B., Bourne, P. E., Bouwman, J., Brookes, A. J., Clark, T., Crosas, M., Dillo, I., Dumon, O., Edmunds, S., Evelo, C. T., Finkers, R., Gonzalez-Beltran, A., Gray, A. J. G., Groth, P., Goble, C., Grethe, J. S., Heringa, J., 't Hoen, P. A. C., Hooft, R., Kuhn, T., Kok, R., Kok, J., Lusher, S. J., Martone, M. E., Mons, A., Packer, A. L., Persson, B., Rocca-Serra, P., Roos, M., van Schaik, R., Sansone, S.-A., Schultes, E., Sengstag, T., Slater, T., Strawn, G., Swertz, M. A., Thompson, M., van der Lei, J., van Mulligen, E., Velterop, J., Waagmeester, A., Wittenburg, P., Wolstencroft, K., Zhao, J., and Mons, B.: The FAIR Guiding Principles for scientific data management and stewardship, Sci. Data, 3, 160018, https://doi.org/10.1038/sdata.2016.18 , 2016.
WMO-OSCAR: Observing Systems Capability Analysis and Review Tool, https://space.oscar.wmo.int/ (last access: 21 March 2024), 2024.
Wunch, D., Toon, G. C., Blavier, J. F. L., Washenfelder, R. A., Notholt, J., Connor, B. J., Griffith, D. W. T., Sherlock, V., and Wennberg, P. O.: The Total Carbon Column Observing Network, Philos. Trans. R. Soc. A, 369, 2087–2112, https://doi.org/10.1098/rsta.2010.0240 , 2011.
Zanatta, M., Gysel, M., Bukowiecki, N., Muller, T., Weingartner, E., Areskoug, H., Fiebig, M., Yttri, K. E., Mihalopoulos, N., Kouvarakis, G., Beddows, D., Harrison, R. M., Cavalli, F., Putaud, J. P., Spindler, G., Wiedensohler, A., Alastuey, A., Pandolfi, M., Sellegri, K., Swietlicki, E., Jaffrezo, J. L., Baltensperger, U., and Laj, P.: A European aerosol phenomenology-5: Climatology of black carbon optical properties at 9 regional background sites across Europe, Atmos. Environ., 145, 346–364, https://doi.org/10.1016/j.atmosenv.2016.09.035 , 2016.
Zhao, Z. and Hellström, M.: Towards Interoperable Research Infrastructures for Environmental and Earth Sciences, Lecture Notes in Computer Science, Springer Cham, Open Access, 373 pp., https://doi.org/10.1007/978-3-030-52829-4 , 2020.
zu Castell, W., Ruhnke, R., Bouwer, L. M., Brix, H., Dietrich, P., Dransch, D., Frickenhaus, S., Greinert, J., and Petzold, A.: Data Science and Earth System Science, in: Integrating Data Science and Earth Science: Challenges and Solutions, edited by: Bouwer, L. M., Dransch, D., Ruhnke, R., Rechid, D., Frickenhaus, S., and Greinert, J., Springer International Publishing, Cham, 1–6, https://doi.org/10.1007/978-3-030-99546-1_1 , 2022.
- Setting the stage
- Entering the era of data-intensive science by integrating atmospheric sciences, data science, and open science
- Opening up new research opportunities
- Appendix A: Sustainable infrastructures for monitoring atmospheric composition
- Data availability
- Author contributions
- Competing interests
- Special issue statement
- Acknowledgements
- Financial support
- Review statement

- Current Students
- Faculty / Staff
- Paying for College
- Alumni Services
- Partnerships
- Program Finder
- Affordable, Flexible, Accessible
- Distance Education
- All Online Courses & Degrees
- Baccalaureate Online
- Graduate Online
- Start Dates
- Admissions, Costs & Aid
- Faculty and Contacts
- Academic and Career Support
- Student Testimonials
- Distance Education Advantage
- About Hybrid Learning
- Hybrid Learning Degrees
- Student Life
- Academic Support
- Academic Calendar
- Faculty & Contacts
- Technical Institute for Environmental Professions
- Term Calendar
- Sustainable Ventures
- Careers & Outcomes
- About Unity
- Office of the President
- Announcing Our Evolution
- Sustainable Achievements & Initiatives
- Reinventing College
- Extended Reality (XR)
- Commencement
- Give to Unity Environmental University
- Institutional Communications
- Unity Environmental University News
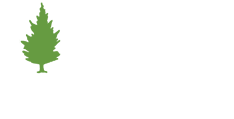
The Importance of Quantitative Methods in Environmental Science and Sustainability Measurement
March 23, 2017
Home / News / The Importance of Quantitative Methods in Environmental Science and Sustainability Measurement
Environmental science is a continuously evolving academic field that seeks to help us gain a progressively better understanding of our natural world and develop effective solutions for important sustainability issues. Challenging the status quo to address environmental problems requires solid evidence to persuade decision makers of the necessity of change. This makes quantitative literacy essential for sustainability professionals to interpret scientific data and implement management procedures.
With our world facing increasingly complex environmental issues, quantitative techniques reduce the numerous uncertainties by providing a reliable representation of reality, enabling us to proceed toward potential solutions with greater confidence. A wide range of statistical tools and approaches are now available for sustainability scientists to measure environmental indicators and inform responsible policy-making.
How Quantitative Methods Provide Context for Environmental Science and Sustainability
Environmental science brings a transdisciplinary systems approach to analyzing sustainability concerns. As the intrinsic concept of sustainability can be interpreted according to diverse values and definitions, quantitative methods based on rigorous scientific research are crucial for establishing an evidence-based consensus on pertinent issues that provide a foundation for meaningful policy implementation.
Statistical evidence is often necessary to defend conservation conclusions
Descriptive and inferential statistical evidence provides a strong foundation for defending conclusions to various audiences. Applying an appropriate range of data sources and quantitative models can produce logical inferences to estimate the probability of future results while quantifying the extent of uncertainty, limits, and future research needs. Given the urgency of environmental issues like climate change and the prevalence of skeptics, effectively summarizing and communicating irrefutable results of complex statistical analyses can make the difference in developing successful courses of action.
How an M.S. in Sustainability Integrates Quantitative Methods
In an M.S. in Sustainability , such as natural resource management, students acquire the fundamental quantitative literacy to correctly evaluate and interpret ecological literature. They learn how to design effective studies, integrate quantitative models, and apply advanced statistical approaches.
For example, Bayesian methods are used to enable scientists to systematically factor in various forms of prior evidence while observing how conclusions change with the new information. This allows a quicker reaction to emerging conditions. Bayesian statistical inference has successfully been applied in conservation biology, addressing many of the problems inherent in standard hypothesis testing while including important factors causing uncertainty. It provides an alternate framework for decision-making that permits more options and better conclusions.
Statistical Models Mitigate Environmental Science and Sustainability Uncertainty
The principles of statistics and probability, multivariate analysis, and spatial analysis methods provide a common ground for scientists, engineers, and other environmental professionals to communicate with each other. Despite the sophistication of the latest mathematical models, the enormous complexity of interactions between environmental systems introduces some level of uncertainty into all predictions.
The quantitative methods acquired in a Sustainability Master’s online combine information from various sources to create more informed predictions, while importantly providing the scientific reasoning to accurately describe what is known and what is not. This quantification of uncertainty makes it impossible to dismiss climate and conservation models, therefore providing a clearer impetus for change.
Interested in pursuing a rewarding career in environmental science and sustainability ?
Contact Unity College to learn about our online Master’s Professional Science programs.
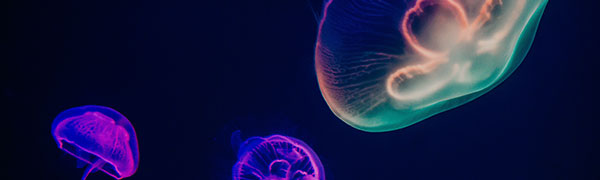
Start Your Journey
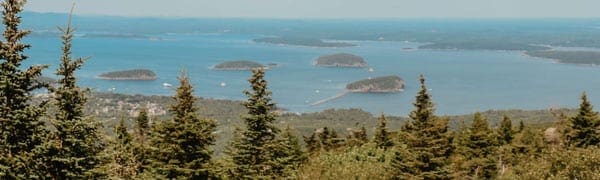
Looking for Answers
Get More Info
© Unity Environmental University 2024. “America’s Environmental University.™”
Privacy Overview

Saturated soils could impact survival of young trees planted to address climate change
The saturated soil conditions predicted to result from increased rainfall in the UK’s upland regions could have a knock-on effect on the ambition to create more woodland in the fight against climate change, a new study has found.
Researchers from the University of Plymouth have spent a number of years exploring how temperate rainforests could be an effective nature-based solution to some of the planet’s greatest challenges.
They have also shown that the UK’s uplands could in future see significantly more annual rainfall than is currently being predicted in national climate models.
In new research, they found that higher soil water levels within areas such as Dartmoor, the Lake District and the Scottish Highlands could have a significant impact on the survival rates of both acorns and juvenile oak saplings.
Published in the journal Forest Ecology and Management , it is the first study to highlight the importance of factoring in soil conditions when looking at where and how to create the temperate rainforests of the future.
Dr Thomas Murphy, Lecturer in Environmental Sciences at the University of Plymouth, is the study’s lead author. He said: “In recent years, there have been increasing calls to plant more trees as part of the global effort to combat climate change. Restoration and expansion of temperate rainforests, which are a globally rare ecosystem, is seen as one of the potential solutions. But with our previous work also predicting an increase in future rainfall we wanted to know if the woodlands we create will support naturally colonising trees in future. Our results show that higher water levels within soils directly contribute to reduced survival of both acorns and young oak trees. We believe it provides landowners, land managers and policy makers with important information as to which species might work in particular locations to support more resilient future rainforests.”
For the study, researchers planted acorns from English oaks (Quercus robur) in containers with four soil states, from completely flooded to low saturation where the water level was 220mm below the acorn.
The acorns did not survive in the flooded soils, but survival rates improved gradually – 43% at high saturation, 77% at medium saturation, and 83% at low saturation – as the water level dropped.
The surviving seedlings also exhibited reduced root:shoot ratio, leaf photosynthesis, and a lower likelihood of late season shoot growth in soils of higher saturation.
In a concurrent field experiment, juvenile English oak and Sessile oak (Quercus petraea) saplings were planted in a region of Dartmoor that is seasonally waterlogged and frequented by grazing livestock.
In these tests, the English oaks exhibited greater shoot growth and leaf photosynthesis than its close relation in areas where the soil was more saturated.
The researchers, including environmental scientists and ecologists, say the results highlight the need for better understanding of soil influence on tree development.
Dr Murphy added: “There has been extensive talk about how larger trees respond to the effects of climate change. But these results show we need to factor in the response of young trees as well, especially if they are being envisioned as an integral part of the solution. By examining their response to conditions now, while also thinking about what these locations are going to be like in 50 years’ time, we can better understand the right trees for the right locations, and hopefully make these woodlands more resilient in the long-term.”
- Environmental Issues
- Global Warming
- Environmental Awareness
- Global warming
- Global warming controversy
- Climate engineering
- Consensus of scientists regarding global warming
- Effects of global warming
- Common Eider
Story Source:
Materials provided by University of Plymouth . Original written by Alan Williams. Note: Content may be edited for style and length.
Journal Reference :
- Thomas R. Murphy, Mick E. Hanley, Jon S. Ellis, Paul H. Lunt. Soil saturation limits early oak establishment in upland pastures for restoration of Atlantic oak woodlands . Forest Ecology and Management , 2024; 561: 121895 DOI: 10.1016/j.foreco.2024.121895
Cite This Page :
Explore More
- Controlling Shape-Shifting Soft Robots
- Brain Flexibility for a Complex World
- ONe Nova to Rule Them All
- AI Systems Are Skilled at Manipulating Humans
- Planet Glows With Molten Lava
- A Fragment of Human Brain, Mapped
- Symbiosis Solves Long-Standing Marine Mystery
- Surprising Common Ideas in Environmental ...
- 2D All-Organic Perovskites: 2D Electronics
- Generative AI That Imitates Human Motion
Trending Topics
Strange & offbeat.
Evaluation of commercial importance of endophytes isolated from Argemone mexicana and Papaver rhoeas
- Innovations and Advances in Environmental Sciences & Sustainable Development
- Published: 07 May 2024
Cite this article
- Pooja Singh 1 ,
- Angkita Sharma 1 ,
- Sahana Mukherjee 1 ,
- Manobjyoti Bordoloi 2 &
- Shoma Paul Nandi ORCID: orcid.org/0000-0003-1416-2425 1
32 Accesses
Explore all metrics
The paper industry is a composite one constituting different types of mills, processes, and products. The paper industries consume large amounts of resources, like wood and water. These industries also create huge amounts of waste that have to be treated. In our study, 23 endophytic bacteria were isolated from Argemone mexicana , and 16 endophytic bacteria were isolated from Papaver rhoeas . Seventeen and 15 bacterial endophytes from A. mexicana and P. rhoeas , respectively, showed cellulose-degrading activity. The biochemical and molecular characterization were done for endophytic bacteria with cellulolytic activity. The consortium of cellulose-degrading endophytic bacteria from A. mexicana showed endoglucanase activity (0.462 IU/ml) and FPCase enzyme activity (0.269 IU/ml) and from P. rhoeas gave endoglucanase activity (0.439 IU/ml) and FPCase enzyme activity (0.253 IU/ml). Degraded carboxy methylcellulose and filter paper were further treated by Saccharomyces cerevisiae and bioethanol was produced. Cellulose-degrading endophytic bacteria were also tested for auxin, siderophore production, and phosphate solubilization activities. Individual cellulose-degrading endophytic bacteria with plant growth-promoting activities were used as biofertilizers, tested for plant growth-promoting activities using Basmati Pusa 1121 rice, and plant growth parameters were recorded. The degraded paper enhances the growth of rice plants. Selected bacterial endophytes and their consortia from A. mexicana and P. rhoeas were powerful cellulose degraders, which can be further employed for ethanol production and as significant biofertilizers in agriculture.
This is a preview of subscription content, log in via an institution to check access.
Access this article
Price includes VAT (Russian Federation)
Instant access to the full article PDF.
Rent this article via DeepDyve
Institutional subscriptions
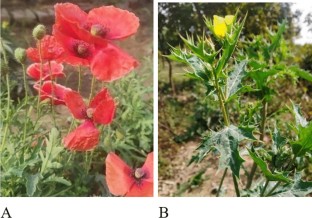
Similar content being viewed by others
Microbial enzymes: industrial progress in 21st century.
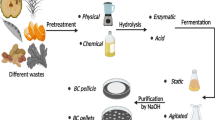
Recent advances in bacterial cellulose: a low-cost effective production media, optimization strategies and applications
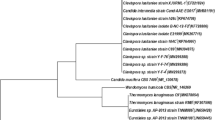
Agricultural Waste Management by Production of Second-Generation Bioethanol from Sugarcane Bagasse Using Indigenous Yeast Strain
Data availability.
The datasets used and/or analyzed during the current study are available from the corresponding author upon reasonable request.
Alam A, Khan AA (2020) Argemone mexicana L.: a weed with versatile medicinal and pharmacological applications. Ann Phytomed Int J 9: 218–223. https://doi.org/10.21276/ap.2020.9.1.29
Alori ET, Glick BR, Babalola OO (2017) Microbial phosphorus solubilization and its potential for use in sustainable agriculture. Front Microbiol 8:971. https://doi.org/10.3389/fmicb.2017.00971
Article Google Scholar
Bashir A, Nigar S, Yousaf S (2013) Isolation and identification of cellulose degrading bacteria from municipal waste and their screening for potential antimicrobial activity. World Appl Sci J 27:1420–1426. https://doi.org/10.5829/idosi.wasj.2013.27.11.81162
Article CAS Google Scholar
Behera BC, Parida S, Dutta SK, Thatoi HN (2014) Isolation and Identification of cellulose degrading bacteria from mangrove soil of Mahanadi river delta and their cellulase production ability. Am J Microbiol Res 2(1): 41–46. https://doi.org/10.12691/ajmr-2-1-6
Brahmachari G, Gorai D, Roy R (2013) Argemone mexicana : chemical and pharmacological aspects. Rev Bras Farmacogn 23:559–567. https://doi.org/10.1590/S0102-695X2013005000021
Brown DM, Pawlak J, Grunden AM (2021) Bacterial valorization of pulp and paper industry process streams and waste. Appl Microbiol Biotechnol 105(4):1345–1363. https://doi.org/10.1007/s00253-021-11107-2
Chatli AS, Beri V, Sidhu BS (2008) Isolation and characterisation of phosphate solubilising microorganisms from the cold desert habitat of Salix alba Linn. in trans Himalayan region of Himachal Pradesh. Indian J Microbiol 48(2): 267–273. https://doi.org/10.1007/s12088-008-0037-y
Christian M, Steffens B, Schenck D, Burmester S, Böttger M, Lüthen H (2006) How does auxin enhance cell elongation? Roles of auxin-binding proteins and potassium channels in growth control. Plant Biol (stuttg) 8(3):346–352. https://doi.org/10.1055/s-2006-923965
Compant S, Mitter B, Colli-Mull JG, Gangl H, Sessitsch A (2011) Endophytes of grapevine flowers, berries, and seeds: identification of cultivable bacteria, comparison with other plant parts, and visualization of niches of colonization. Microb Ecol 62(1):188–197. https://doi.org/10.1007/s00248-011-9883-y
De Almeida MN, Guimarães VM, Bischoff KM et al (2011) Cellulases and hemicellulases from endophytic Acremonium species and its application on sugarcane bagasse hydrolysis. Appl Biochem Biotechnol 165:594–610. https://doi.org/10.1007/s12010-011-9278-z
De Marco ÉG, Heck K, Martos ET, Van der Sand ST (2017) Purification and characterization of a thermostable alkaline cellulase produced by Bacillus licheniformis 380 isolated from compost. Anais Da Acad Brasil De Ciências 89(3):2359–2370. https://doi.org/10.1590/0001-3765201720170408
Dillon J (2004) Dillon VM (2004) The gut bacteria of insects non pathogenic interaction. Annu Rev Entomol 49:71–92. https://doi.org/10.1146/annurev.ento.49.061802.123416
Eida MF, Nagaoka T, Wasaki J, Kouno K (2012) Isolation and characterization of cellulose-decomposing bacteria inhabiting sawdust and coffee residue composts. Microbes Environ 27(3):226–233. https://doi.org/10.1264/jsme2.ME11299
Fadiji AE, Babalola OO (2020) Elucidating mechanisms of endophytes used in plant protection and other bioactivities with multifunctional prospects. Front Bioeng Biotechnol 8:467. https://doi.org/10.3389/fbioe.2020.00467
Fahim S, Nisar N, Ahmad Z, Asghar Z, Said A, Atif S et al (2019) Managing paper and pulp industry by-product waste utilizing sludge as a bio-fertilizer. Pol J Environ Stud 28(1). https://doi.org/10.15244/pjoes/83614
Felsenstein J (1985) Confidence limits on phylogenies: an approach using the bootstrap. Evolution 39:783–791. https://doi.org/10.1111/j.1558-5646.1985.tb00420.x
Ferbiyanto A, Rusmana I, Rafudin R (2015) Characterization and identifcation of cellulolytic bacteria from gut of worker Macrotermes gilvus . HAYATI J Biosci 22:197–200. https://doi.org/10.1016/j.hjb.2015.07.001
Flimban S, Oh SE, Joo JH et al (2019) Characterization and identification of cellulose-degrading bacteria isolated from a microbial fuel cell reactor. Biotechnol Bioproc E 24:622–631. https://doi.org/10.1007/s12257-019-0089-3
Gaur VK, Sharma P, Sirohi R, Awasthi MK, Dussap CG, Pandey A (2020) Assessing the impact of industrial waste on environment and mitigation strategies: a comprehensive review. J Hazard Mater 398:123019. https://doi.org/10.1016/j.jhazmat.2020.123019
Gopal PM, Sivaram NM, Barik D (2019) Paper industry wastes and energy generation from wastes. In Energy from toxic organic waste for heat and power generation. Woodhead Publishing,pp 83–97. https://doi.org/10.1016/B978-0-08-102528-4.00007-9
Gupta P, Samant K, Sahu A (2012) Isolation of cellulose degrading bacteria and determination of their cellulolytic potential. Int J Appl Microbiol Sci 1(2):13–23. https://doi.org/10.1155/2012/578925
Haile A, Gelebo GG, Tesfaye T, Mengie W, Mebrate MA, Abuhay A, Limeneh DY (2021) Pulp and paper mill wastes: utilizations and prospects for high value-added biomaterials. Bioresour Bioprocess 8(1):1–22. https://doi.org/10.1186/s40643-021-00385-3
Hossain MA, Ahammed MA, Sobuj SI, Shifat SK, Somadder PD (2021) Cellulase producing bacteria isolation, screening and media optimization from local soil sample. Am J Microbiol Res 9(3): 62–74 https://doi.org/10.12691/ajmr-9-3-1
Islam F, Roy N (2018) Screening, purification and characterization of cellulase from cellulase producing bacteria in molasses. BMC Res Notes 11:445. https://doi.org/10.1186/s13104-018-3558-4
Jain D, Sanadhya S, Saheewala H, Maheshwari D, Shukwal A, Singh PB, Meena RH, Choudhary R, Mohanti SR, Singh A (2020) Molecular diversity analysis of plant growth promoting rhizobium isolated from groundnut and evaluation of their field efficacy. Curr Microbiol 77:1550–2155. https://doi.org/10.1007/s00284-020-01963-y
Jain D, Ravina BAA, Chauhan S, Rajpurohit D, Mohanty SR (2021) Polyphasic characterization of plant growth promoting cellulose degrading bacteria isolated from organic manures. Curr Microbiol 78(2):739–748. https://doi.org/10.1007/s00284-020-02342-3
Kaur C, Gupta M, Garai S, Mishra SK, Chauhan PS, Sopory S, Singla-Pareek SL, Adlakha N, Pareek A (2022) Microbial methylglyoxal metabolism contributes towards growth promotion and stress tolerance in plants. Environ Microbiol 24(6):2817–2836. https://doi.org/10.1111/1462-2920.15743
Kour R, Jain D, Bhojiya AA, Sukhwal A, Sanadhya S, Saheewala H, Jat G, Singh A, Mohanty SR (2019) Zinc biosorption, biochemical and molecular characterization of plant growth-promoting zinc-tolerant bacteria. 3 Biotech 9(11): 421 https://doi.org/10.1007/s13205-019-1959-2
Krishnan S, Ahmad MF, Zainuddin NA, Din MFM, Rezania S, Chelliapan S et al (2020) Chapter 9: bioethanol production from lignocellulosic biomass (water hyacinth): a biofuel alternative. Bioreactors-Sustainable Design and Industrial Applications in Mitigation of GHG Emissions. Elsevier, pp 123–143. https://doi.org/10.1016/B978-0-12-821264-6.00009-7
Kumar GS, Chandra MS, Sumanth M, Vishnupriya A, Reddy RB, Choi YL (2009) Cellulolytic enzymes from submerged fermentation of different substrates by newly isolated Bacillus spp. FME J Korean Soc Appl Bi 52:17–21. https://doi.org/10.3839/jksabc.2009.003
Kumar S, Stecher G, Li M, Knyaz C, Tamura K (2018) MEGA X: molecular evolutionary genetics analysis across computing platforms. Mol BiolEvol 35:1547–1549. https://doi.org/10.1093/molbev/msy096
Lo YC, Saratale GD, Chen WM, Bai MD, Chang JS (2009) Isolation of cellulose-hydrolytic bacteria and applications of the cellulolytic enzymes for cellulosic biohydrogen production. Enzyme Microb Technol 44:417–425. https://doi.org/10.1016/j.enzmictec.2009.03.002
Maki ML, Broere M, Leung KT, Qin W (2011) Characterization of some efficient cellulase producing bacteria isolated from paper mill sludges and organic fertilizers. Int J Biochem Mol Biol 2:146–154
CAS Google Scholar
Maniatis T, Fritsch EF, Sambrook J (1982) Molecular cloning: a laboratory manual, cold spring harbor (N Y: cold spring harbor laboratory), pp 545. https://doi.org/10.1016/0307-4412(83)90068-7
Miller GL (1959) Use of dinitrosalicylic acid reagent for determination of reducing sugar. Anal Chem 31:426–428. https://doi.org/10.1021/ac60147a030
N’dayegamiye A, Nyiraneza J, Giroux M, Grenier M, Drapeau A (2013) Manure and paper mill sludge application effects on potato yield, nitrogen efficiency and disease incidence. Agronomy 3(1):43–58. https://doi.org/10.3390/agronomy3010043
Naik SB, Abrar S, Krishnappa M (2019) Industrially important enzymes from fungal endophytes. Rec Adv White Biotechnol Through Fungi 263–280. https://doi.org/10.1007/978-3-030-10480-1_7
Nei M, Kumar S (2000) Molecular evolution and phylogenetics. Oxford University Press, New YorkF
Book Google Scholar
Ng LC, Sariah M, Sariam O, Radziah O, Abidin MA (2012) Rice seed bacterization for promoting germination and seedling growth under aerobic cultivation system. Aust J Crop Sci 6:170–175
Nunes JR, Cabral F, Lopez-Pineiro A (2008) Short-term effects on soil properties and wheat production from secondary paper sludge application on two Mediterranean agricultural soils. Bioresour Technol 99(11):4935–4942. https://doi.org/10.1016/j.biortech.2007.09.016
Pahari A, Pradhan A, Nayak SK, Mishra BB (2017) Bacterial siderophore as a plant growth promoter. In: Patra J, Vishnuprasad C, Das G (eds) Microbial biotechnology. Springer, Singapore. https://doi.org/10.1007/978-981-10-6847-8_7
Patten CL, Glick BR (2002) Regulation of indoleacetic acid production in Pseudomonas putida GR12-2 by tryptophan and the stationary-phase sigma factor RpoS. Can J Microbiol 48(7):635–642. https://doi.org/10.1139/w02-053
Quaye AK, Volk TA, Hafner S, Leopold DJ, Schirmer C (2011) Impacts of paper sludge and manure on soil and biomass production of willow. Biomass bioenerg 35(7):2796–2806. https://doi.org/10.1016/j.biombioe.2011.03.008
Rastogi G, Muppidi GL, Gurram RN, Adhikari A, Bischoff KM, Hughes SR, Apel WA, Bang SS, Dixon DJ, Sani RK (2009) Isolation and characterization of cellulose-degrading bacteria from the deep subsurface of the Homestake gold mine, Lead, South Dakota, USA. J Ind Microbiol Biotechnol 36(4):585–598. https://doi.org/10.1007/s10295-009-0528-9
Ratnaweera PB, de Silva ED, Williams DE, Andersen RJ (2015) Antimicrobial activities of endophytic fungi obtained from the arid zone invasive plant Opuntia dillenii and the isolation of equisetin, from endophytic Fusarium sp. BMC Complem Altern M 15: 220 https://doi.org/10.14288/1.0074648
Rawway M, Ali SG, Badawy AS (2017) Isolation and identification of cellulose degrading bacteria from different sources at Assiut governorate Upper Egypt. J Ecol Health Environ 6(1):15–24. https://doi.org/10.18576/jehe/060103
Sadhu S, Maiti TK (2013) Cellulase production by bacteria: a review. Br Microbiol Res J 3(3):235–258. https://doi.org/10.9734/BMRJ/2013/2367
Saitou N, Nei M (1987) The neighbor-joining method: a new method for reconstructing phylogenetic trees. Mol Biol Evol 4:406–425. https://doi.org/10.1093/oxfordjournals.molbev.a040454
Saxena S, Bahadur J, Varma A (1993) Cellulose and hemicellulose degrading bacteria from termite gut and mould soils of India. Indian J Microbiol 33:55–60
Google Scholar
Schulz B, Guske S, Dammann U, Boyle C (1998) Endophyte-host interactions II. Defining symbiosis of the endophyte-host interactions. Symbiosis, Philadelphia. Pa (USA) 25:213–227
Sharma S, Prasad RK, Chatterjee S, Sharma A, Vairale MG, Yadav KK (2019) Characterization of Bacillus species with keratinase and cellulase properties isolated from feather dumping soil and cockroach gut. Proc Natl Acad Sci India Sect B Biol Sci 89(3):1079–1086. https://doi.org/10.1007/s40011-018-1026-5
Sharma A, Singh P, Sarmah BM, Nandi SP (2020) Isolation of cellulose-degrading endophyte from Capsicum chinense and determination of its cellulolytic potential. Biointerface Res Appl Chem 10(6):6964–6973. https://doi.org/10.33263/BRIAC106.69646973
Sim CSF, Chen SH, Ting ASY (2019) Endophytes: emerging tools for the bioremediation of pollutants. In Emerging and eco-friendly approaches for waste management. Springer, Singapore, pp 189–217. https://doi.org/10.1007/978-981-10-8669-4_10
Singh S, Singh TD, Singh VP, Pandey VB (2010) Quaternary alkaloids of Argemone mexicana . Pharm Biol 48(2):158–160. https://doi.org/10.3109/13880200903062622
Singh P, Sharma A, Bordoloi M, Nandi SP (2020) Molecular identification of endophytic fungi isolated from medicinal plant. Biointerface Res Appl Chem 10:6436–6443. https://doi.org/10.33263/BRIAC105.64366443
Teather RM, Wood PJ (1982) Use of Congo red-polysaccharide interactions in enumeration and characterization of cellulolytic bacteria from the bovine rumen. Appl Environ Microbiol 43(4):777–780. https://doi.org/10.1128/aem.43.4.777-780.1982
Tran TTA, Le TKP, Mai TP, Nguyen DQ (2019) Bioethanol production from lignocellulosic biomass. In: alcohol fuels-current technologies and future prospect. IntechOpen https://doi.org/10.5772/intechopen.86437
Vimal J, Venu A, Joseph J (2016) Isolation and identification of cellulose degrading bacteria and optimization of the cellulase production. Int J Res Biosciences 5(3):58–67. https://doi.org/10.15680/IJIRSET.2015.0408012
Yousef N, Mawad A, Abeed A (2019) Enhancement the cellulase activity induced by endophytic bacteria using calcium nanoparticles. Curr Microbiol 76(3):346–354. https://doi.org/10.1007/s00284-018-1614-x
Download references
Acknowledgements
The authors acknowledge the intellectual support from Prof. Amithabh Bandopadhyay. PS acknowledges a fellowship from UGC.
The work is financially supported by NE-DBT (Grant No: AGRI/2015/48) of SPN.
Author information
Authors and affiliations.
Amity Institute of Biotechnology, Amity University, Noida, Uttar Pradesh, India
Pooja Singh, Angkita Sharma, Sahana Mukherjee & Shoma Paul Nandi
Department of Chemistry, Cotton University, Guwahati, Assam, India
Manobjyoti Bordoloi
You can also search for this author in PubMed Google Scholar
Contributions
SPN, PS, AS, and MJB conceptualized the problem and designed some of the experiments. PS, AS, and SM designed and performed the experiments, and analyzed the data. The manuscript was written by PS which was further edited and modified by SPN. All authors read and approved the final manuscript.
Corresponding author
Correspondence to Shoma Paul Nandi .
Ethics declarations
Ethics approval and consent to participate.
Not applicable.
Consent for publication
Competing interests.
The authors declare no competing interests.
Additional information
Responsible Editor: Ta Yeong Wu
Publisher's Note
Springer Nature remains neutral with regard to jurisdictional claims in published maps and institutional affiliations.
Rights and permissions
Springer Nature or its licensor (e.g. a society or other partner) holds exclusive rights to this article under a publishing agreement with the author(s) or other rightsholder(s); author self-archiving of the accepted manuscript version of this article is solely governed by the terms of such publishing agreement and applicable law.
Reprints and permissions
About this article
Singh, P., Sharma, A., Mukherjee, S. et al. Evaluation of commercial importance of endophytes isolated from Argemone mexicana and Papaver rhoeas . Environ Sci Pollut Res (2024). https://doi.org/10.1007/s11356-024-33527-z
Download citation
Received : 22 April 2023
Accepted : 27 April 2024
Published : 07 May 2024
DOI : https://doi.org/10.1007/s11356-024-33527-z
Share this article
Anyone you share the following link with will be able to read this content:
Sorry, a shareable link is not currently available for this article.
Provided by the Springer Nature SharedIt content-sharing initiative
- Papaveraceae
- Cellulose-degrading bacteria
- Endoglucanase
- Plant growth-promoting bacteria
- Agriculture
- Phytohormones
- Find a journal
- Publish with us
- Track your research
Democrats say Big Oil misled public for decades about climate change
Major oil companies have misled Americans for decades about the threat of human-caused climate change, according to a new report released Tuesday by Democrats in Congress.
The 65-page report was the result of a three-year investigation and was made public hours before a Senate Budget Committee hearing about the role that oil and gas companies have played in global warming.
“They could’ve been the environmental Paul Revere but, instead, they were more like Rip Van Winkle, wanting everyone to go to sleep,” Rep. Jamie Raskin, D-Md., said about fossil fuel companies’ efforts to mislead and distract the American public for more than 60 years. “The thing that gets me the most is thinking back to the decades when ‘Big Oil and Gas’ understood the problem in a way almost no one in the country or the world did.”
Democrats’ investigation revealed research, transcripts and even video recordings that show the fossil fuel industry knew the consequences of its emissions since at least the 1960s. Their report also showed how oil and gas companies initially tried to hide that information but employed new tactics to downplay the urgency of eliminating emissions.
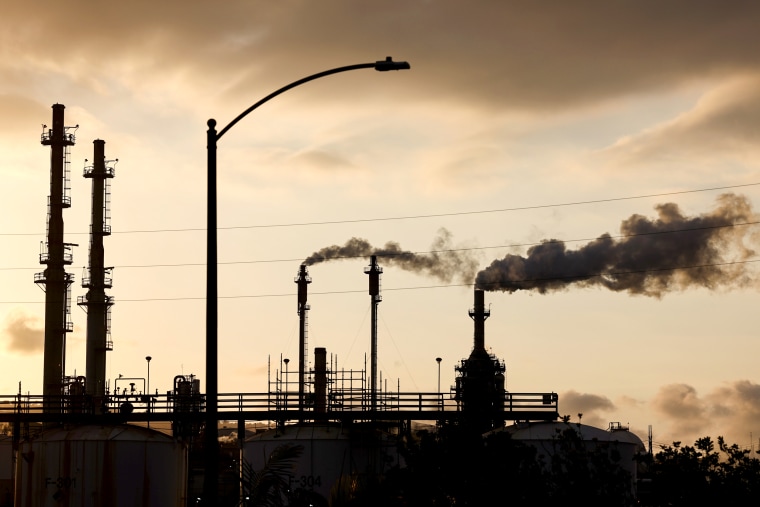
Geoffrey Supran, an associate professor and director of the Climate Accountability Lab at the University of Miami, researches climate disinformation and propaganda from the fossil fuel industry. He said that oil and gas companies’ claims of decarbonizing are just their latest strategy to delay climate action.
“Putting spin before science continues at oil companies to this day,” he said of his research into the tactics of the industry.
“This is greenwashing 101,” Supran added. “Talk green, act dirty.”
Senate Republicans called the hearing purely partisan and attempted to refocus the discussion on the financial cost of rapidly transitioning away from oil and gas.
“We spend all this money, and we don’t lower global temperatures one scintilla of a degree,” said Republican Sen. John Kennedy of Louisiana, one of the top oil and gas producing states in the U.S.
The American Petroleum Institute (API), a major lobbying group for the industry, was repeatedly blamed in the report and Senate hearing for helping oil and gas companies hide the truth about climate change. According to Senate Democrats, API both advised fossil fuel companies on public relations strategies, while also acting as a scapegoat for congressional scrutiny.
In a statement to NBC News, an API spokesperson said, “At a time of persistent inflation and geopolitical instability, our nation needs more American energy — including more oil and natural gas — and less unfounded election year rhetoric. America’s energy workers are focused on delivering the reliable, affordable oil and natural gas Americans demand while scaling the next generation of low-carbon technologies like hydrogen and carbon capture, and any suggestion to the contrary is inaccurate.”
Democrats reiterated that oil and gas companies have damaged the planet for decades without having to pay for the consequences. That led Sen. Bernie Sanders, I-Vt., to ask what happens next.
“If we have an industry that knowingly, and that’s the point … knowingly understood that climate change would bring devastating destruction to the lives of billions of people, what are the legal grounds we can hold them accountable for?” he said.
Chase Cain is a national climate reporter for NBC News.
Thank you for visiting nature.com. You are using a browser version with limited support for CSS. To obtain the best experience, we recommend you use a more up to date browser (or turn off compatibility mode in Internet Explorer). In the meantime, to ensure continued support, we are displaying the site without styles and JavaScript.
- View all journals
- Explore content
- About the journal
- Publish with us
- Sign up for alerts
- 01 May 2024
Why it’s essential to study sex and gender, even as tensions rise
You have full access to this article via your institution.
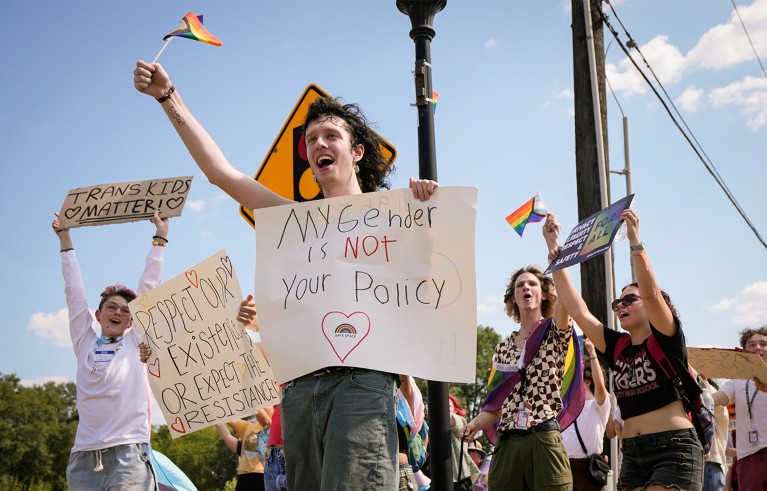
In 2023, students protested against a new policy in Texas, where parents would be notified if their child asks to be identified as transgender. Credit: Brett Coomer/Houston Chronicle/Getty
This week, Nature is launching a collection of opinion articles on sex and gender in research. Further articles will be published in the coming months. The series will highlight the necessity and challenges of studying a topic that is both hugely under-researched and, increasingly, the focus of arguments worldwide — many of which are neither healthy nor constructive.
Some scientists have been warned off studying sex differences by colleagues. Others, who are already working on sex or gender-related topics, are hesitant to publish their views. Such a climate of fear and reticence serves no one. To find a way forward we need more knowledge, not less.
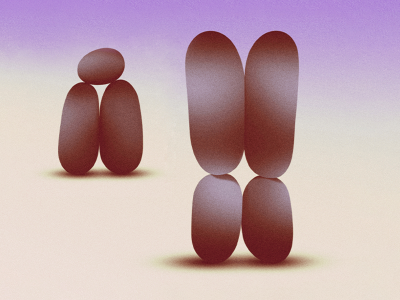
Collection: Sex and gender in science
Nearly 20 researchers from diverse fields, including neuroscience, psychology, immunology and cancer, have contributed to the series, which provides a snapshot of where scholars studying sex and gender are aligned — and where they are not. In time, we hope this collection will help to shape research, and provide a reference point for moderating often-intemperate debates.
In practice, people use sex and gender to mean different things. But researchers studying animals typically use sex to refer to male and female individuals , as defined by various anatomical and other biological features. In studies involving humans, participants are generally asked to identify their own sex and/or gender category. Here, gender usually encompasses social and environmental factors , including gender roles, expectations and identity.
For as long as scientific inquiry has existed, people have mainly studied men or male animals. Even as recently as 2009, only 26% of studies using animals included both female and male individuals, according to a review of 10 fields in the biological sciences 1 . This bias has had serious consequences. Between 1997 and 2000, for instance, eight prescription drugs were removed from the US market, because clinical testing had not revealed women’s greater risk of developing health problems after taking the drugs.
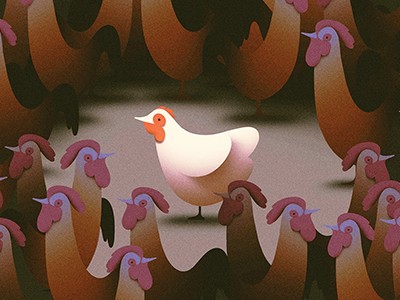
Male–female comparisons are powerful in biomedical research — don’t abandon them
The tide, however, is turning. Many journals, including those in the Nature Portfolio , and funders, such as the US National Institutes of Health, have developed guidelines and mandates to encourage scientists to consider sex and, where appropriate, gender in their work.
These efforts are reaping benefits 2 . Studies, for example, are showing that a person’s sex and/or gender can influence their risk of disease and chances of survival when it comes to many common causes of death — including cardiovascular conditions and cancer.
Despite this, many researchers remain unconvinced that the inclusion of sex and gender information is important in their field. Others, who are already doing so, have told Nature that they’re afraid of how their work is perceived and of how it could be misunderstood, or misused.
Podcast: Sex and gender discussions don't need to be toxic
Because researchers who are exploring the effects of sex and gender come from many disciplines, there will be disagreements. An often-raised and valid concern, for example, is that when researchers compare responses between female and male animals, or between men and women, they exclude those whose sex and/or gender doesn’t fall into a binary categorization scheme. Another is that variability between individuals of the same sex could be more important than that between sexes.
Sometimes sense does seem to get lost in the debates. That the term sex refers to a lot of interacting factors, which are not fully understood, does not invalidate its usefulness as a concept 3 . That some people misinterpret and misuse findings concerning differences between sexes, particularly in relation to the human brain, should not mean denying that any differences exist.
Tempering the debate
Many of the questions being raised, however, are important to ask, especially given concerns about how best to investigate biological differences between groups of humans , and the continued — and, in some regions, worsening — marginalization of people whose sex and/or gender identity doesn’t fall into narrowly defined norms. Often, such questions and concerns can be addressed through research. For example, studies might find that variability between individuals of the same sex in diet, or body weight, say, are more important predictors of how likely they are to develop anaemia than whether they are male or female.
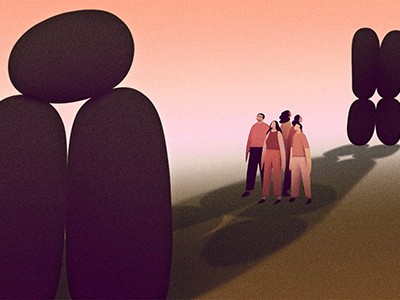
We need more-nuanced approaches to exploring sex and gender in research
The problem, then is not the discussions alone: science exists to examine and interrogate disagreements. Rather, the problem is that debates — and work on sex and gender, in general — are being used to polarize opinions about gender identity. As Arthur Arnold, a biologist at the University of California, Los Angeles, and his colleagues describe in their Comment article , last September, legislation banning gender-affirming medical care for people under 18 years old was introduced in Texas on the basis of claims that everyone belongs to one of two gender groups, and that this reality is settled by science. It isn’t. Scientists are reluctant to study sex and gender, not just because of concerns about the complexity and costs of the research, but also because of current tensions.
But it is crucial that scholars do not refrain from considering the effects of sex and gender if such analyses are relevant to their field. Improved knowledge will help to resolve concerns and allow a scholarly consensus to be reached, where possible. Where disagreements persist, our hope is that Nature ’s collection of opinion articles will equip researchers with the tools needed to help them persuade others that going back to assuming that male individuals represent everyone is no longer an option.
Nature 629 , 7-8 (2024)
doi: https://doi.org/10.1038/d41586-024-01207-0
Beery, A. K. & Zucker, I. Neurosci. Biobehav. Rev. 35 , 565–572 (2011).
Article PubMed Google Scholar
Tannenbaum, C., Ellis, R. P., Eyssel, F., Zou, J. & Schiebinger, L. Nature 575 , 137–146 (2019).
Velocci, B. Cell 187 , 1343–1346 (2024).
Download references
Reprints and permissions
Related Articles
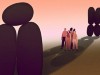
- Research data
- Research management
Japan can embrace open science — but flexible approaches are key
Correspondence 07 MAY 24
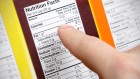
Researchers want a ‘nutrition label’ for academic-paper facts
Nature Index 17 APR 24
Adopt universal standards for study adaptation to boost health, education and social-science research
Correspondence 02 APR 24
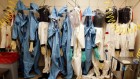
US funders to tighten oversight of controversial ‘gain of function’ research
News 07 MAY 24
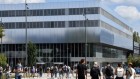
France’s research mega-campus faces leadership crisis
News 03 MAY 24
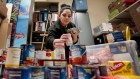
Hunger on campus: why US PhD students are fighting over food
Career Feature 03 MAY 24
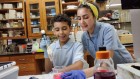
US National Academies report outlines barriers and solutions for scientist carers
Career News 02 MAY 24
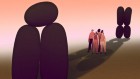
Comment 01 MAY 24
Southeast University Future Technology Institute Recruitment Notice
Professor openings in mechanical engineering, control science and engineering, and integrating emerging interdisciplinary majors
Nanjing, Jiangsu (CN)
Southeast University
Staff Scientist
A Staff Scientist position is available in the laboratory of Drs. Elliot and Glassberg to study translational aspects of lung injury, repair and fibro
Maywood, Illinois
Loyola University Chicago - Department of Medicine
W3-Professorship (with tenure) in Inorganic Chemistry
The Institute of Inorganic Chemistry in the Faculty of Mathematics and Natural Sciences at the University of Bonn invites applications for a W3-Pro...
53113, Zentrum (DE)
Rheinische Friedrich-Wilhelms-Universität
Principal Investigator Positions at the Chinese Institutes for Medical Research, Beijing
Studies of mechanisms of human diseases, drug discovery, biomedical engineering, public health and relevant interdisciplinary fields.
Beijing, China
The Chinese Institutes for Medical Research (CIMR), Beijing
Research Associate - Neural Development Disorders
Houston, Texas (US)
Baylor College of Medicine (BCM)
Sign up for the Nature Briefing newsletter — what matters in science, free to your inbox daily.
Quick links
- Explore articles by subject
- Guide to authors
- Editorial policies

IMAGES
VIDEO
COMMENTS
The critical scientific research areas in the future are as follows: (1) mass and energy transportation in the soil and its stabilization mechanisms; (2) processes and impacts on the soil ecosystem; (3) response and feedback of soil to global changes; and (4) sustainable management of soil and land resources. 4.2.5.
Environmental science is defined as an interdisciplinary academic field that integrates various academic fields (particularly sciences) to study the structure and function of our life-supporting ...
The importance of environmental science has never been greater as the entire natural world is experiencing the negative effects of ... Not only has scientific research led to the creation of laws and regulations aimed at protecting the environment, but environmental scientists are often responsible for conducting field site surveys and other ...
Irreproducible research has negative consequences beyond the obvious impact on achieving new scientific discoveries that can advance healthcare and enable new technologies. The conduct of science is resource intensive, resulting in a large environmental impact from even the smallest research programs.
The UN's recent announcement of a Decade of Ocean Science provides a glimmer of hope, but scientists will need to work closely with decision-makers and society at large to get the ocean back on ...
Environmental science is the multidisciplinary study of all aspects of the Earth's physical and biological environments. It encompasses environmental chemistry, soil science, ecology ...
In a recent case specifically related to amphibians, Calhoun et al. demonstrate the importance of "interdisciplinary and engaged" (p. 11005) research, and promote interdisciplinary research as ...
The EU Research Agenda developed by the HERA project covers six major research goals on environment, climate and health. Within each of them, research areas were identified and research needs specified resulting in altogether 30 specific research goals (Table 1).Several of the research goals are interlinked e.g. air-pollution is identified as a priority in the global environment (Research Goal ...
98. 99. 100. Qualitative research methods examine a wide range of topics in the study of environment and resource management. This first review on the topic highlights innovative and impactful research over the past few decades, drawing from social science disciplines that include sociology, geography, anthropology, political science, public ...
Abstract. Research plays a crucial central role between physicians and regulatory agencies in assessing the potential risks posed by an ever-increasing variety of environmental pollutants. The explosion in our understanding of biology and the development of the powerful tools of molecular biology during the last 50 years have provided us with a ...
Thus, it is clear that environmental studies are vital for spreading awareness and finding ways to protect it. It refers to a systematic study of the environment and its physical, biological, social, cultural, and natural factors. You can say environmental studies are all about learning how we should protect our environment with sustainable ...
Ten years after the first Earth Day in 1970, environmental activist Senator Gaylord Nelson wrote a letter about the importance of the environment to the American people and the world. ... Research - Environmental science researchers examine environmental issues using the scientific method, monitor these issues over time and collaborate to ...
What could the future of environmental health sciences hold, and what steps might be taken now to guide the field's trajectory? To envision a future research enterprise that integrates environmental health sciences, biomedical science, prevention research, and disease-specific research across the continuum from fundamental discovery research through the application of this research to ...
Introduction. Research environments matter. Environmental considerations such as robust cultures of research quality and support for researchers are thought to be the most influential predictors of research productivity.1, 2 Over 25 years ago, Bland and Ruffin1 identified 12 characteristics of research‐favourable environments in the international academic medicine literature spanning the ...
Building a global culture of sustainability in science will be crucial to reducing the carbon footprint of laboratories. Laboratories are typically highly resource-intensive spaces with large ...
Education in research ethics is can help people get a better understanding of ethical standards, policies, and issues and improve ethical judgment and decision making. Many of the deviations that occur in research may occur because researchers simply do not know or have never thought seriously about some of the ethical norms of research.
2.1 Atmosphere-centred research infrastructures in Europe. Recognising that the in-depth investigation of the effects of climate change and their impacts on human health, food security, and other aspects of the United Nations Sustainable Development Goals (SDG, 2024) requires a critical mass in terms of scientific capacities and resources, the European Commission has initiated the development ...
Environmental science brings a transdisciplinary systems approach to analyzing sustainability concerns. As the intrinsic concept of sustainability can be interpreted according to diverse values and definitions, quantitative methods based on rigorous scientific research are crucial for establishing an evidence-based consensus on pertinent issues ...
The researchers, including environmental scientists and ecologists, say the results highlight the need for better understanding of soil influence on tree development.
Focus on Environmental Development. Environmental science aims to avoid stream pollution and re-educate the general population about the importance of recycling to preserve our available resources. Based on data-driven planning models, environmentalists seek to direct development towards sustainability.We must use every available resource in the most efficient way possible so that it can be ...
The environmental impact of research increasingly needs to be taken into account in design and execution. This makes good financial sense. However, it is especially in the research world as one of the key reasons for doing health research is to improve our knowledge to improve health. Specifically, doing research in a more sustainable way ...
Developing countries can adapt to climate change effectively using nature-based solutions. Nature-based solutions can reduce the immediate risks and impacts of climate change in coastal areas and ...
Environmental Science and Pollution Research - The paper industry is a composite one constituting different types of mills, processes, and products. ... growth promotion, nutrient assimilation, nitrogen fixation, and enhanced defense response. Endophytes are commercially important due to their ability to produce industrially relevant enzymes ...
Major oil companies have misled Americans for decades about the threat of human-caused climate change, according to a new report released Tuesday by Democrats in Congress. The 65-page report was ...
Movement Ecology (2023) Citizen science is an increasingly acknowledged approach applied in many scientific domains, and particularly within the environmental and ecological sciences, in which non ...
Computer Science A. Thursday, May 9, 2024. Chinese Language and Culture. Environmental Science. Psychology. Friday, May 10, 2024. European History. United States History. ... AP Seminar and AP Research students to submit performance tasks as final and their presentations to be scored by their AP Seminar or AP Research teachers.
A neuropsychologist, a biochemist, a cell biologist, and an ecological engineer from the University of the Free State (UFS) have all received their first nomination in this year's NSTF-South32 Awards. The four researchers have been nominated in the TW Kambule-NSTF Award: Emerging Researcher category.These emerging researchers are part of a group of nine UFS researchers nominated for the ...
Scientists are reluctant to study sex and gender, not just because of concerns about the complexity and costs of the research, but also because of current tensions. But it is crucial that scholars ...