Academia.edu no longer supports Internet Explorer.
To browse Academia.edu and the wider internet faster and more securely, please take a few seconds to upgrade your browser .
Enter the email address you signed up with and we'll email you a reset link.
- We're Hiring!
- Help Center
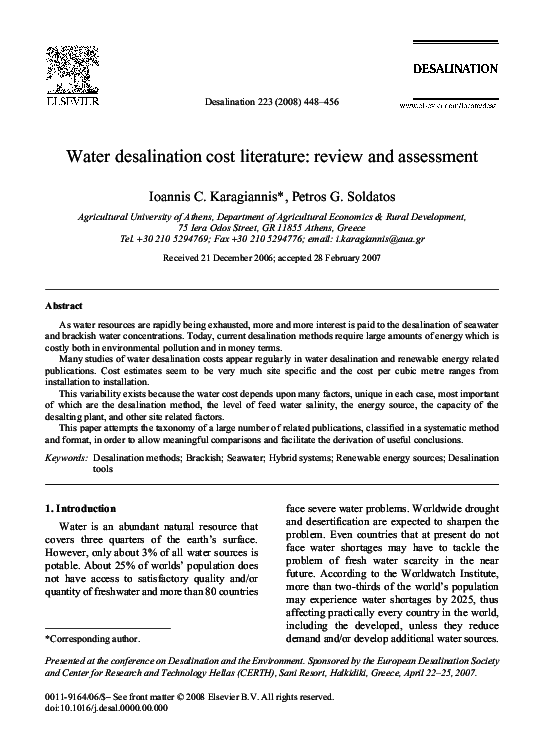

Water desalination cost literature: review and assessment

2008, Desalination
Related Papers
Desalination
Thomas Missimer
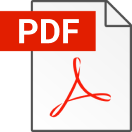
In the last decade desalination has been considered as a solution for potable water needs only for specific water scarcity countries having cheap fuel. Now, desalination is extensively used, even where it was unthinkable twenty years back, due to reduction in desalination cost. The cost reduction is due to new developments and improvements in desalination technologies, particularly in RO technology. The RO is a well accepted technology due to recent increase in energy prices and takes up a major share in worldwide market. But, it is not able to achieve its proper share in the Arabian Gulf market due to difficult seawater composition and extensive historical use of thermal desalination. But RO still has potential in hybrid systems in the Arabian Gulf to account for seasonal and night to day fluctuations in the demand for power and water. There is a need for an accurate methodology for evaluation of desalination costs to help in selection of appropriate technology suitable for a specific location, for process design and other requirements. However, existing methodologies and software packages do not account for all the parameters that contribute for desalting cost and their accuracy is limited to specific conditions. This paper presents an overview of the trends in desalination costs for major desalination technologies like Multi Stage Flash, Multi Effect Distillation and Reverse Osmosis and review of costing methodologies.
Desalination and Water Treatment
Massimo Moser , Jürgen Kern
المجلة الأكاديمية للأبحاث والنشر العلمي
ahmed hamdan
Water Resources Research
Mona Amin , Ghada Al Bazedi
Fresh water scarcity in many countries limits sustainable development; in many cases sea water desalination represents an ideal solution. Reverse osmosis membrane technology has developed over the past 50 years and it has 80% share in the total number of desalination plants installed worldwide. In this study, the approaches adopted for the economic evaluation of SWRO desalination processes, cost estimation programs and published equations that evaluate the capital and the total cost of desalinated water have been reviewed, analyzed and evaluated for further implementation of desalination plants. Four methods have been selected to simulate different desalination capacities. All costs have been updated using ENR cost index for 2015. The results show that for large capacities, the capital and unit costs are in accordance with a percent deviation when comparing different cost estimation procedure. WTCOST and DEEP software show comparable unit cost $0.96/m 3 and $0.99/m 3 , respectively; while for capacities less than 1000 m 3 /d there is a significant percent deviation between methods. 1. Introduction Today, reverse osmosis membranes are the one of leading technologies for the desalination process, as they are applied to a diversity of salt water resources using membrane system design and tailored pretreatment. A wide variety of research and general information on RO desalination is available; however, a direct comparison of seawater and brackish water RO systems is necessary to highlight similarities and differences in process development [1]. One of the most critical features of any water treatment project is the cost. For membrane desalination, decreasing costs and producing higher water quality are among a number of important reasons why this technology continues. The cost of RO desalination plant is divided into direct capital cost, indirect capital cost and annual operating cost. Direct capital costs include the cost of land, major and auxiliary process equipment, and construction costs. Freight and insurance, construction overheads, and contingency costs are part of the indirect capital costs. While, annual operating costs are after plant commissioning and during plant operation and include chemicals, energy, wages, plant maintenance, expenditures etc. A wide distribution of these cost items are reported [2-3]. An exploratory study has been conducted to review the approaches adopted for the economic evaluation of SWRO desalination processes. Cost estimation programs that evaluate the capital and the total cost of desalinated water have been developed by several research and commercial entities. These water desalting cost programs enable estimation of desalting costs for sea water or brackish water using various technologies. Equations representing cost models have been developed by several authors. In addition, conceptual cost estimates for desalting process have been developed in graphical form [4].
Mohamed Eltawil
Water Conditioning & Purification
Nikolay Voutchkov
Mark Bennet
The paper reviews the cost of different water supply and water treatment options around the world. The cost of supplying ground water is found to be proportional to the lift. The relationship between cost and volume of water treated by specific treatment options is assessed. Vehicular transportation of water is found to be very costly compared to wastewa-ter treatment and compared to conventional water treatment and supply. Efforts have been made to differentiate the cost of water with respect to its application in various sectors. The capital cost of infrastructure required to extract, treat, supply, and reclaim water is also studied. Finally, the effects of precipitation, geographic aspects, population, financial, regulatory laws, and social attributes of a specific region are considered as they affect the cost of water. Global use of water in agriculture and the costs of agricultural irrigation are studied. Pressurized irrigation systems are costlier compared to flood or surface irrigation systems.
Journal of Membrane and Separation Technology
Lifescience Global Canada
Desalination applications based on reverse osmosis (RO) technology today comprise over 50% of the capacity of all desalination systems worldwide and represent 75-85% of new desalination projects being implemented. The major reason for the shift in desalination projects to RO technology is the high energy efficiency of the RO process. There are three major application categories of large capacity, RO-based desalination projects: brackish RO; advanced municipal wastewater reclamation; and seawater RO. In the two first categories (brackish RO and wastewater reclamation), the systems' configuration and equipment components are well defined. Therefore, project costs and operating expenses are fairly predictable. In seawater RO desalination systems, the RO process configuration is also very similar; however, some variability exists regarding the configuration of seawater water delivery and feed water pretreatment. The rest of the system's components and system operation methods are very uniform. However, an evaluation of published cost data of medium-to large-scale water RO desalination projects illustrates significant variability in costs of desalination systems. This paper will analyze current economic conditions of seawater desalination, and highlight the limitations and possibilities of additional improvements of the economics of the SWRO desalination process.
RELATED PAPERS
Marina Pankova
Journal of biomolecular techniques : JBT
Satyahari Dey
Fausto Amaro
The Journal of Clinical Endocrinology & Metabolism
Fernando Civeira
Ririen Prihandarini Dr.Ir
Can Tho University Journal of Science
marcus M holanda
Journal of Materials Chemistry B
María Dolores RUBIANES
ECMS 2010 Proceedings edited by A Bargiela S A Ali D Crowley E J H Kerckhoffs
Tribology International
Salete Martins Alves
Revista do Instituto de Medicina Tropical de São Paulo
Mirian Sotto
Electoral Studies
James Tilley
Maria Virgínia A Martins
Anuário do Instituto de Geociências
Marìa Isabel Haag
Monika Foltyn-Zarychta
Victor Manuel Valderrama Ramirez
American Journal of Medical Genetics Part B: Neuropsychiatric Genetics
Josep Antoni Ramos-Quiroga
Jefferson Mortatti
Elizabeth Resendiz Sanchez
CSUN毕业证成绩单 加州州立大学北岭分校学位证
RELATED TOPICS
- We're Hiring!
- Help Center
- Find new research papers in:
- Health Sciences
- Earth Sciences
- Cognitive Science
- Mathematics
- Computer Science
- Academia ©2024

Brackish (4) As water resources are rapidly being exhausted, more and more interest is paid to the desalination of seawater and brackish water concentrations. Today, current desalination methods require large amounts of energy which is costly both in environmental pollution and i ...
Seawater (54) As water resources are rapidly being exhausted, more and more interest is paid to the desalination of seawater and brackish water concentrations. Today, current desalination methods require large amounts of energy which is costly both in environmenta ...
Hybrid systems (4) ... mainly due to much lower energy consumption and the recent technological advances that have been achieved in membranes. Under special conditions hybrid systems can offer increased and more stable production of fresh water. Desalination 223 (2008) 448–456 Water desalination cost literature: revie ...
Desalination methods () As water resources are rapidly being exhausted, more and more interest is paid to the desalination of seawater and brackish water concentrations. Today, current desalination methods require large amounts of energy which is costly both i ...
Desalination (272) As water resources are rapidly being exhausted, more and more interest is paid to the desalination of seawater and brackish water concentrations. Today, current desalination methods require large amounts of energy which is costly both i ...
Renewable energy sources (3) ... is costly both in environmental pollution and in money terms. Many studies of water desalination costs appear regularly in water desalination and renewable energy related publications. Cost estimates seem to be very much site specific and the cost per cubic metre ranges from installation to... the cost for seawater ranges from 0.4h/m3 to more than 3h/m3, while for brackish water desalination the cost is almost half. When renewable energy sources are used the cost is much higher, and in some cases can reach even 15h/m3, due to most expensive energy supply systems. However, this cost i ...
Key issues in Seawater Desalination
Seawater Intake
Reverse Osmosis Desalination Process
Desalination Pretreatment Chlorination Dechlorination Chlorinator system
Desalination Post-treatment Boron Removal Remineralization
Desalination Polishing steps
Desalination and Energy Costs
Brine Disposal
Membranes cleaning and troubleshooting
Instrumentation and Control
Membrane Technology Plate & Frame membranes
Desalination
Desalination with Reverse Osmosis modules
Watermakers
Ultra High Recovery Seawater Desalination
About Lenntech
Lenntech (european head office).
Distributieweg 3 2645 EG Delfgauw The Netherlands Phone: +31 152 610 900 fax: +31 152 616 289 e-mail: [email protected]
Lenntech USA LLC (Americas)
5975 Sunset Drive South Miami, FL 33143 USA Phone: +1 877 453 8095 (speaks English & Spanish) e-mail: [email protected]
Lenntech DMCC (Middle East)

Copyright © 1998-2020 Lenntech B.V. All rights reserved
Thank you for visiting nature.com. You are using a browser version with limited support for CSS. To obtain the best experience, we recommend you use a more up to date browser (or turn off compatibility mode in Internet Explorer). In the meantime, to ensure continued support, we are displaying the site without styles and JavaScript.
- View all journals
- My Account Login
- Explore content
- About the journal
- Publish with us
- Sign up for alerts
- Open access
- Published: 15 May 2024
Assessing the suitability of desalination techniques for hydraulic barriers
- Hanna Rosentreter ORCID: orcid.org/0000-0003-0608-0104 1 ,
- Marc Walther 2 &
- André Lerch ORCID: orcid.org/0000-0002-6355-9122 1
npj Clean Water volume 7 , Article number: 40 ( 2024 ) Cite this article
95 Accesses
1 Altmetric
Metrics details
- Environmental sciences
Seawater intrusion is a worldwide increasing challenge, which lowers the freshwater availability by salination of fresh groundwater resources in coastal areas. The abstraction-desalination-recharge (ADR) methodology can combat seawater intrusion, whereby desalination is hereby the key factor for the overall efficiency of aquifer remediation. Which desalination technique is suitable within ADR depends on several factors and was not discussed before. We use a multi-criteria decision analysis and cost analysis to compare nanofiltration, reverse osmosis, electrodialysis, and (membrane) capacitive deionization and show for three case scenarios which desalination technique is most suitable within ADR. Overall, electrodialysis, nanofiltration, and reverse osmosis have shown the best utility value for saline groundwater salinity of 1–10 g L −1 , whereby electrodialysis is more suitable for lower salinities. The lowest desalination costs are calculated for nanofiltration and reverse osmosis with 0.3–0.6 € m −3 depending on specific energy costs. Even capacitive deionisation can be a suitable alternative for low, slightly saline groundwater (1 g L −1 ) if the technology readiness level and a lifetime of electrodes increase and material costs decrease. These new insights provide a data analysis, costs, and decision support for desalination which are needed for the holistic approach to counteract seawater intrusion.
Similar content being viewed by others
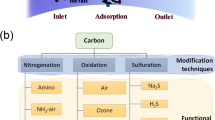
Removal of heavy metal ions from wastewater: a comprehensive and critical review
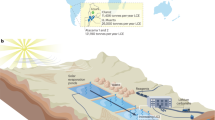
Environmental impact of direct lithium extraction from brines
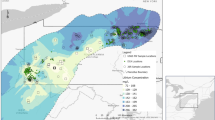
Estimates of lithium mass yields from produced water sourced from the Devonian-aged Marcellus Shale
Introduction.
Groundwater salination describes the process of increasing the salinity of fresh groundwater resources. This process is driven by inland geogenic salt deposits, saline (waste) waters, or the inland drift of seawater into fresh groundwater aquifers 1 . The latter—often published with the term seawater intrusion—occurs due to increasing groundwater abstraction at coastal areas and rising sea levels decreasing freshwater availability 2 , 3 . In order to satisfy the need for water for drinking water, irrigation, and industrial purposes, sustainable management of the groundwater aquifer is needed. Reducing fresh groundwater abstraction and increasing natural groundwater recharge would remediate the aquifer but is often impossible to implement due to the growth of population and water needs in coastal sites. Abrupt reduction of pumping rates would rather lead to an increased seawater intrusion and long recovery periods 4 , 5 . Managed aquifer recharge is recommended to overcome the seawater intrusion problematic, whereby water-scarce regions show a lack of adequate water resources for recharge 6 , 7 . Therefore, desalination would be a key process to reduce the saline water amount in the aquifer and to ensure simultaneously water in adequate quality and quantity for recharge. A so called mixed hydraulic barrier system provides a negative barrier for the abstraction of saline groundwater (SGW) and a positive barrier as recharge to drive the saltwater interface towards the sea and remediate the aquifer 8 . The abstraction-desalination-recharge (ADR) methodology can be extended by using the desalinated water for domestic use and recharging treated wastewater 7 (Fig. 1 ).
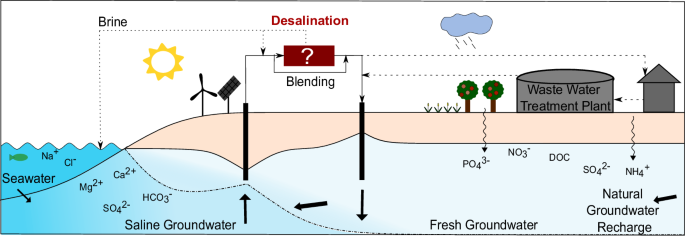
Abstraction-desalination-recharge by treated wastewater (ADRTWW) is shown in dashed lines for remediation of saline aquifers affected by saltwater intrusion. Dashed-dotted line symbolises the interface between freshwater and saline groundwater, which is pushed back seaward by ADR.
A practical application of ADR is not known to the authors. However, publications by Abd-Elhamid & Javadi 8 , Ebeling, Händel & Walther 9 , and Saad et al. 10 proved the effective remediation of ADR by groundwater flow models. Remediation costs will thereby depend on site-specific costs for the abstraction, desalination, and recharge processes. The quantitative and qualitative requirements for desalination within ADR depend on regional conditions and are summarised according to the literature as follows.
Results of simulated ADR scenarios of Ebeling, Händel & Walther 9 , Sadjad Mehdizadeh, Badaruddin & Khatibi 11 and Abd-Elaty, Abd-Elhamid & Qahman 12 show that the remediation duration of a saline aquifer can be reduced by increasing the recharge rate. According to these studies, depending on the specific hydraulic characteristics of the aquifer, >100 m 3 desalinated water per day and coast kilometer are needed for recharge, achieving a remediation duration of 3–100 years.
Saline groundwater effected by seawater intrusion usually show a sodium chloride concentration exceeding the drinking water and irrigation limit values 13 , 14 . SGW is classified in slightly, moderately, highly and very highly SGW due to their electrical conductivity or chloride concentration 15 , 16 (Table 1 ).
The time and location-dependent specific composition of SGW depends thereby on the chemical, physical, and biological processes in the aquifer 14 . Seawater intrusion indicated processes, as cation exchange, calcite dissolution and sulphate reduction lead to mineralisation of the groundwater with calcium, magnesium, bicarbonate, dissolved nutrients, inorganic carbon, organic carbon, sulphide and metals 3 , 17 which trigger scaling and fouling within desalination 18 . However, high nitrate concentrations in SGW often result from agriculture 19 , 20 .
Referring to Escalante et al. 21 , so far there exists no general guidelines or fixed limit concentrations for sodium and chloride concentrations for managed aquifer recharge. According to this publication and van der Bruggen 22 , the target concentration produced by desalination should meet regional conditions for optimal recharge and final use as the limit quality for drinking water 23 and irrigation 13 , 24 (Table 2 ). According to Martin 25 , the concentration for recharge water should equal the natural composition of the initial present freshwater concentration to reduce swelling, dissolution, precipitation, and clogging processes, which results in reduction of hydraulic conductivity of the aquifer. No complete desalination of the SGW is needed for managed aquifer recharge. The sodium chloride proportion of the total suspended solids (TDS) in SGW is usually 25% up to 90% 14 , therefore the rejection of sodium chloride (R NaCl ) needs usually to be lower than 90 % . The salt rejection by desalination is defined by the SGW concentration ( c SGW ) and the target recharge water concentration ( c recharge ).
If SGW contains organic carbon 26 , retention of organic matter is recommended to circumvent clogging problems during recharge 25 . Since the aquifer is prone to dissolved oxygen and pH changes as well 27 , 28 , aeration and intensive pH drifts during desalination should be avoided. Further, chemical use and temperature changes should be kept at a minimum since the brine, which consists of concentrated salts, is usually disposed into the sea 29 , 30 .
Since desalination is an energy consuming process, the specific energy consumption (SEC) for desalination will define the economic and ecologic feasibility of ADR. In anthropogenic-caused seawater intrusion-affected areas electric energy is usually available whereby these areas correlate with high solar radiation or wind power 31 , 32 , 33 , which makes desalination techniques powered by renewable energy more attractive for ADR. Since the theoretical minimum desalination energy needed for brackish water is lower than for seawater 34 , SEC for SGW desalination within ADR should be lower than the minimum SEC of seawater with 2.5 kWh m −3 35 , 36 in order to compete with managed aquifer recharge by seawater desalination.
Since several factors, as water quality and water quantity as well as the energy supply by renewable energy, are variable in time, flexible desalination technologies are favoured within ADR methodology. Further, adequate pre-treatment and post-treatment needs to be maintained as for other desalination applications according to the used desalination techniques 37 to maintain an energy efficient treatment process.
Classic pressure-driven desalination techniques, as nanofiltration (NF) and reverse osmosis (RO), and electrochemical desalination techniques, as electrodialysis (ED), comply with the above-described requirements and show according to Wang et al. 34 and Patel, Biesheuvel & Elimelech 38 the lowest SEC for brackish water desalination. Contrary, thermal large-scale-desalination techniques as multiple effect distillation, multistage flash distillation, and mechanical vapour compression show a higher SEC and are more applicable for brines 30 . Desalination techniques, such as membrane distillation, solar distillation, humidification-dehumidification, and microbiological desalination, show a high energy consumption, are up to now not suitable for commercial large-scale applications or are less investigated for brackish water desalination 39 , 40 , 41 .
RO is the most often used desalination technique regarding seawater and brackish water desalination 30 . Within this technology, hydrostatic pressure greater than the osmotic pressure of the saline solution is used to drive the liquid through a membrane against the natural direction of osmosis, producing a permeate stream on the effluent site and a concentrate stream (brine) on the influent site of the membrane. RO membranes are usually constructed as spiral-wound modules 42 . The solution diffusion model was mostly used to describe the transport mechanisms within RO before Wang et al. 43 emphasised that the water transport is driven by a pressure gradient and should be described by pore flow. Steric exclusion, Donnan effect, and dielectric exclusion of semipermeable membranes affect the specific salt rejection. RO is classified by the rising rejection rate of the membrane and rising feed pressure in: brackish water reverse osmosis (BWRO) and seawater reverse osmosis (SWRO). In addition, NF membranes, which are generally characterized by an even higher water and salt flux than BWRO, are often applied for low brackish water concentrations 44 .
In contrast to NF and RO, where the solvent as liquid is separated from the feed, electrochemical desalination technologies separate the ions of the feed, making these technologies, especially for lower salt rejections more advantageous 45 . Electrodialysis (ED) uses an electric potential difference to transport charged anions and cations through an ion exchange membrane (IEM) to produce a low-saline solution in diluate chambers and a high-saline solution in concentrate chambers, which are stacked in parallel. The salt rejection is described by electromigration, convection and diffusion 46 . Most of the ED processes are operated as ED reversal, whereby the concentration polarisation on the membranes can be reduced by reversing the electric potential. ED is usually used for concentrate recovery or for brackish water desalination if no total desalinated product is needed 47 .
Similar to ED, capacitive deioniation (CDI) is an electrochemical membrane technology, whereby charged ions can adsorb in the electric double layer of a positively and a negatively charged electrode producing a low-saline solution 48 . If static electrodes are used, alternating desorption processes by short-circuiting or reversing the electrical current produce a concentrate solution 49 . Electrode coatings or the implementation of ions exchange membranes in CDI, called membrane capacitive deionisation (MCDI), improves the charge efficiency 50 , 51 . Further, flow electrode CDI can ensure continuous desalination even at higher concentrated brackish water concentrations and resource recovery but needs higher investments caused by the higher complexity of the system 52 , 53 , 54 .
Which desalination technique is suitable and how high the costs would be within ADR is rarely discussed in the literature. Therefore, the main research questions of this paper are: which desalination techniques should be used, and what are the costs within ADR? We use a multi-criteria decision analysis based on a literature review and a cost calculation in order to evaluate which pressure-driven desalination technique (NF/RO) or electrochemical desalination techniques (ED, CDI, and MCDI) are most suitable for three different case studies showing a TDS concentration in SGW of 1 g L −1 (A), 5 g L −1 (B) & 10 g L −1 (C) and site-specific parameters. Here, we include environmental, economical, technical, and social criteria. We distinguish between two different scenarios: a present scenario according to the current parameters and a future scenario according to predicted material improvements, including cost declines and lifespan. In this paper, we focus on the traditional ADR methodology without wastewater treatment, whereby desalination requirements of saline water are similar for both approaches.
Results and discussion
Desalination costs and energy consumption.
Costs and SEC for desalination are calculated for NF/RO, ED, CDI, and MCDI for SGW concentration of our case studies in Table 3 : A - East Frisian Islands in Germany (1 g L −1 ) 55 , 56 , 57 ; B—Kimje in South Korea (5 g L −1 ) 58 and C - Nile Delta in Egypt (10 g L −1 ) 59 , 60 . Results for pressure-driven desalination costs are shown either for NF regarding case A, or for RO regarding case B and C considering which membranes are more efficient (Supplementary Table 1 , Supplementary Fig. 1 , Supplementary Methods ). Figure 2 shows the specific capital expenditures per m 3 (CAPEX), operational expenditures per m 3 (OPEX), SEC and total costs per m 3 in the present and future scenario. Costs and SEC for desalination increase with rising SGW concentrations regardless the desalination technology (Fig. 2a–e ). The rise of costs and SEC with increasing SGW concentration is, however, steeper for the electrochemical technologies than for pressure-driven technologies, showing especially the advantage of RO, and disadvantage of ED, CDI and MCDI for higher SGW concentration and vice versa. The different case study-specific energy costs of Table 3 affect the costs but not these trends, since the influence of energy cost is superimposed by the SGW concentration in our study. The influence of the specific energy costs can be realised by differences between SEC (independent of energy price) in Fig. 2c and OPEX only for the SEC (OPEX energy ) in Fig. 2d . OPEX energy for NF/RO and ED regarding case study A, B, and C does not variate much with 0.04–0.10 € m −3 compared to the SEC with 0.18–1.62 kWh m −3 due to the highest energy costs for case study A and the lowest energy costs for case study C. Detailed data can be found in Supplementary Table 2 and Supplementary Table 3 . The calculated costs and SEC are generally in range with data given by literature (Supplementary Table 4 ). It should be noted that SEC and costs for CDI and MCDI for case C are only theoretically calculated and that these techniques are usually not suitable for a salinity of 10 g L −1 61 .
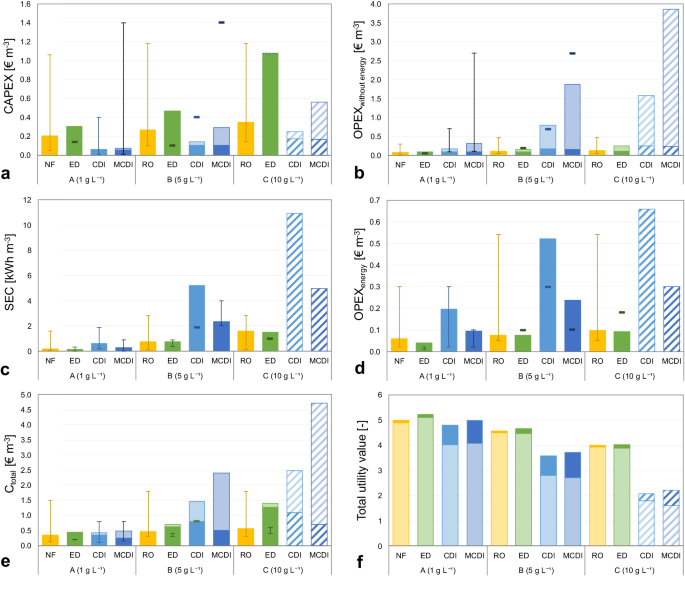
a Capital expenditures (CAPEX), b operational expenditures without energy (OPEX without energy ), c specific energy consumption (SEC), d energy costs (OPEX energy ), e total costs (C total ), and f total utility value for NF/RO, ED, CDI, and MCDI regarding case study A, B, and C. Costs of the future scenario are shown in a more glaring colour. Bars show comparative maximum and minimum values from other publications, which are listed in Supplementary Table 4 . Pattern filling for CDI and MCDI show theoretical data since these technologies usually not used for SGW concentration of ≥10 g L −1 .
The increase of CAPEX with the SGW concentration for electrochemical desalination techniques is higher than for pressure-driven desalination techniques (Fig. 2a ). According to our calculations, CAPEX is the lowest for CDI with 0.05–0.25 € m −3 by assuming a high capacitance and a high module packing density of Liu et al. 52 . MCDI shows higher CAPEX with 0.07–0.56 € m −3 due to the IEM costs. Hand et al. 62 and Bales et al. 63 predict similar CAPEX, whereby in their publications, CAPEX can be up to 1.4 € m −3 depending on the assumed material characteristics. ED shows with 0.30–1.08 € m −3 higher CAPEX than CDI and MCDI and higher CAPEX than NF/RO with 0.20–0.35 € m −3 . CAPEX of NF/RO and ED comply with real data by Wittholz et al. 64 and Sajtar & Bagley 65 . Especially for higher SGW concentration, less or no data are published to validate the results for CDI and MCDI, compared to ED and NF/RO. CAPEX reduction in the future case scenario (Supplementary Table 3 ) by lower electrode costs and IEM costs (Supplementary Table 7 ) would further reduce CAPEX for CDI and MCDI, whereby CAPEX reduction for ED by lower IEM costs and for pressure-driven desalination techniques by a higher permeability is neglectable ( Supplementary Methods , Supplementary Table 1 ). Increasing or decreasing the desalination capacity by factor 3 lowers and rises CAPEX for the specific desalination techniques, respectively, but does not impact the ratio between the specific techniques (Supplementary Fig. 2 ). Since CAPEX has the highest cost component in total costs of ED (details in Supplementary Table 2 , Supplementary Fig. 1 ) the scale of the desalination plant influences this technique the most.
Regarding the SGW concentration of 1–10 g L −1 , NF/RO shows the lowest OPEX without energy costs (OPEX without energy ) due to low membrane costs, followed by ED (Fig. 2b ). Note that NF membranes are considered for 1 g L −1 and RO membranes for 5–10 g L −1 SGW concentration (Supplementary Table 1 , Supplementary Methods ). OPEX without energy for CDI and MCDI is higher due to replacement of total module every half year up to 3 years for CDI and every 1 to 5 years for MCDI. According to the present scenario, CDI and MCDI are not cost-efficient for SGW concentration >1 g L −1 due to the needed membrane and electrode area, the specific material costs, and a low material lifetime. In the future scenario, the replacement costs for ED, CDI and MCDI can be reduced by the assumed reduced IEM costs and electrode costs as well as by an expanded electrode lifetime. Increasing the lifetime of NF/RO membranes does not lower OPEX due to the low membrane costs.
The lowest SEC and OPEX energy is calculated for ED, whereby the difference to NF/RO decreases with higher SGW concentration (Fig. 2c, d ). The SEC for CDI and MCDI is higher even though we considered energy recovery. In contrast to OPEX without energy , OPEX energy is lower for MCDI than for CDI due to a higher charge efficiency and higher energy recovery of MCDI.
Summarising OPEX and CAPEX of the present scenario, NF and RO show with 0.33 € m −3 and 0.45–0.55 € m −3 the lowest total costs ( C total ) in Fig. 2e for case study A, B, and C, respectively, whereby material improvements will not significantly change the costs as earlier discussed by Werber, Deshmukh & Elimelech 66 . However, future material improvements can lower total costs for ED, CDI, and MCDI for case study C from 1.41 to 1.26 € m −3 , from 2.49 to 1.08 € m −3 and from 4.71 to 0.70 € m −3 , respectively (Supplementary Table 2 in comparison to Supplementary Table 3 ). According to Supplementary Fig. 3a , the general result would be similar assuming for all case studies the same energy consumption, showing total costs for NF/RO between 0.30 and 0.60 € m − 3 . If the theoretical cost assumptions for CDI and MCDI can be verified in big-scale long-term desalination, especially MCDI could be a cost efficient alternative. In total, the SGW salinity, material costs of membranes and electrodes have a high impact on the desalination costs. It needs to be noted, that a target concentration for recharge >0.45 g L −1 would result in a lower needed salt retention, resulting in lower OPEX and CAPEX, especially for electrochemical desalination technologies. Since all results for costs and SEC depend on the rejection of sodium chloride, experimental investigations would bring higher accuracy for specific feedwater compositions, especially for monovalent desalination 45 .
Multi-criteria decision analysis
The resulting total utility values for NF/RO, ED, CDI, and MCDI for case study A, B, and C of the multi-criteria decision analysis are shown in Fig. 2f . The utility value and weight for each criterion, desalination alternative and case study are shown inclusive references in Supplementary Table 5 and Supplementary Table 6 . Criteria as water recovery, retention of sodium chloride, CAPEX, OPEX without energy , technology readiness level, and occupational safety for the desalination techniques are rated with a higher significance regarding the application within the ADR methodology, whereby criteria as the space requirement and flexibility & tolerance are rated with a lower significance (Fig. 3 ).
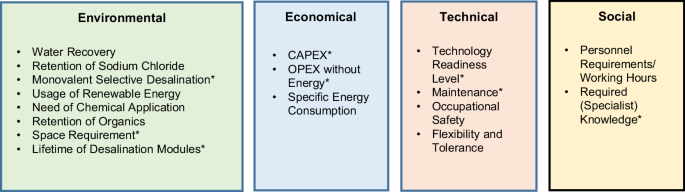
*utility value for criteria is modified for future scenario.
According to our multi-criteria decision analysis, ED and NF/RO show for all case studies in the present scenario, the highest total utility value due to a high water recovery, a high salt retention, a high material lifetime, a high occupational safety level, a high suitability to be coupled with renewable energy, low social requirements and the before mentioned results for CAPEX, OPEX and SEC. For a low SGW concentration as for case study A, especially the electrochemical technologies show a higher total utility value compared to case study B and C cause of their lower need of chemical application, a low SEC, the option of using monovalent desalination and a more flexible process. ED shows therefore the highest utility value for case A in the present and future scenarios. ED benefits in the future scenario by lower costs, improved material properties and higher monovalent ion selectivity of IEM as recently discussed by Patel et al. 67 , giving ED the highest utility value regarding case study B.
NF/RO has a higher total utility value regarding the criteria maintenance and lifetime of materials and shows lower total desalination costs as discussed before. Improvements of the permeability and durability of the membranes does here effectively increase the total utility value for NF/RO in the future scenario in comparison to the before discussed cost and energy results.
CDI and MCDI show in the present scenario a low technology readiness level and a low lifetime of the electrode. Without increasing the durability of the electrode or similar materials, these techniques cannot compete with ED and NF/RO. Due to the finite specific salt adsorption, high costs for IEM, and lower water recovery at higher SGW concentration, these desalination technologies are less suitable for higher SGW concentrations. The drawback of finite salt adsorption might be solved in the future by flow electrode CDI. For low SGW concentrations, CDI and MCDI can be an alternative for NF/RO and ED.
Weighting the criteria of case study A and C similar to case study B as in Supplementary Table 6 —assuming that the case studies would only differ from their SGW concentration—surprisingly do not change the total utility value. Supplementary Fig. 3b shows approximately the same total utility value as in Fig. 2f , showing that in our study the SGW salinity mostly impacts the total utility value of the desalination techniques. The high energy price of Case A has according to our weighting not shown the full effect cause of the low SGW concentration. The energy price would have a higher impact in higher saline case studies because of their higher SEC. Including specific (monovalent) ion rejection, specific case-dependent material costs, and more specific details of a survey with different stakeholders can, however, result in more differentiated utility values.
ADR is a highly important methodology to remediate saline aquifers due to increasing seawater intrusion by climate change and sea level rise within the future. So far, the suitability and costs of different desalination techniques have not been evaluated before. We evaluate NF/RO, ED, CDI, and MCDI according to the specific requirements within ADR by a multi-criteria decision analysis. Based on the current literature, NF/RO and ED are the most suitable technologies within the application of ADR to remediate saline aquifers with a concentration of 1–10 g/L. Especially for slightly SGW, ED shows the highest total utility value according to the low chemical application, high flexibility, low SEC, moderate costs, and monovalent ions selective rejection. In the future scenario, ED would profit verifiably by reduced IEM costs and improved material properties. Regarding the total costs, NF/RO are for the chosen case studies with 0.3–0.6 € m −3 the most cost-effective desalination technology. Even though CDI and MCDI are not able to compete with NF/RO and ED yet, lots of research is currently focused on improving their material drawbacks. With the three site-specific case studies, we want to highlight that the multi-criteria decision analysis and cost analysis depend on the site-specific conditions, parameters, and targets. However, the SGW and product water quality have the highest influence on the evaluation. This analysis could be further extended to an abstraction-desalination-recharge by treated wastewater (ADRTWW) methodology (Fig. 1 ) in order to identify additional costs and possible synergies in water use. The cost and multi-criteria decision analysis of this paper can be coupled with a groundwater transport model to analyze the nexus of salinization, remediation duration, and desalination costs. This coupled model could be further used as decision help in a holistic comparison with alternative methodologies (e.g., the building of water retention and infiltration ponds) to counteract seawater intrusion.
Comparative desalination techniques and case studies
NF, RO, and ED are standard brackish water desalination technologies and are therefore included in our comparative analysis. Even though, CDI is examined mostly in laboratory scale and is controversy discussed in the literature 61 , 68 , 69 , 70 , we included CDI and MCDI as potentially suitable technology in our study due to a high retention of sodium chloride 13 , 71 , 72 , recent economic analyses 52 , 62 , 63 and increasing interest in this technology 73 . A literature study for costs and SEC for brackish water desalination demonstrate the suitability of the preselected desalination technologies according to the before described requirements according quantity (>100 L d −1 ), quality (25% < R NaCl < 90%) and SEC (generally <2.5 kWh m −3 ). CDI and MCDI are usually not applied for feed concentrations of 5–10 g L −1 61 . However, CDI is included for these concentrations to demonstrate the trend with increasing concentrations. Flow electrode CDI, which shows a higher desalination capacity is not included in our study due to less experiences and data at treatment capacities >100 L d −1 53 , 54 .
The suitability of the desalination techniques and the costs depends on the site-specific conditions, therefore a multi-criteria decision analysis and costs analysis for three different SWI affected cases (Table 3 ) is conducted. The East Frisian Islands in Germany, Kimje in South Korea, and the Nile Delta in Egypt were chosen as examples with different SGW concentrations and different economic, social, and environmental characteristics.
Cost and energy calculation
CAPEX, OPEX, and SEC variate according to the SGW concentration and process parameters. In order give a fair comparison in this paper, costs and SEC are calculated for 600 m³/d recharge per kilometres of coastline and a remediation duration of 4 and 50 years according to the simulation of Ebeling, Händel & Walther 9 . The assumed water recovery was 75% with a water abstraction rate of 800 m 3 d −1 . Since CAPEX per produced flow rate are lower at higher capacities up to a limit capacity of 200,000 m 3 d −1 36 , we set the production rate per day to remediate 100 km coastline to 60,000 m 3 d −1 , which corresponds with the recharge rate of 600 m 3 d −1 km −1 . This production capacity is classified as a medium size desalination plant 36 . The capacity is increased and decreased by a factor of 3 in order to check, if this would have a major influence on the costs.
Total desalination costs ( \({C}_{{\rm{total}}}\) ) are divided into capital expenditures and operational expenditures. CAPEX include expenditures as conception, design, construction, while OPEX include regularly operation and maintenance costs associated with the plant as membrane replacement, energy costs, labour and maintenance costs. Since the energy price differs for the case studies (Table 3 ), we differentiate between OPEX without energy (OPEX without energy ), energy costs (OPEX energy ) and SEC.
CAPEX and OPEX for NF, RO and ED are well known due to their large-scale desalination application over decades. The costs for these techniques are calculated similar to Wittholz et al. 64 , Sajtar & Bagley 65 , Abdulbaki et al. 74 and Wreyford et al. 75 based on real large-scale data. Data of large-scale CDI costs are not published even though CDI plants up to 60,000 m 3 d −1 are already in use 76 . The cost calculation for CDI bases therefore on economic analysis of Liu et al. 52 for CDI and MCDI. Suitable NF and RO membranes are chosen regarding the feed concentration and needed retention rates in case A (NF membrane), B (BWRO membrane) and C (SWRO membrane) as pressure and flux and therefore costs changes. This gives a fairer comparison to electrochemical technologies which can be easily adjusted by the applied electric current and voltage.
The total costs per m 3 partial desalinated water are calculated for each desalination technology depending on the specific SGW concentration of case study A, B, and C (1–10 g L −1 , Table 3 ) and a target concentration of 0.45 g/L for the present and future scenario. The target concentration is chosen according to the recommended concentration in Table 2 , which fulfils the recommendation of Escalante et al. 21 and van der Bruggen et al. 22 . Detailed characteristics as feedwater-specific chemical cleaning, specific labour costs and monovalent selective rejection was not included in the cost and energy calculation. However, these characteristics are included in the multi-criteria decision analysis. A detailed description about the cost calculation is shown in Supplementary Methods .
In this paper, the multi-criteria decision analysis is used as decision help method to choose site-specific desalination techniques according to their weights of various criteria. This method gives a flexible transparently evaluation, the possibility to consider even seemingly non-related factors due to the broad criteria composition 77 and is adaptable to before-mentioned requirements. Further, a multi-criteria decision analysis was recently used to evaluate water treatment processes as in AbdulBaki et al. 78 and Wencki et al. 79 . We analysed NF/RO, ED and CDI for 17 criteria, which were divided into four categories: environmental, economical, technical and social (Fig. 3 ).
The desalination alternatives were evaluated according to a fast sustainable remediation of saline aquifers within ADR. A specific utility value ( v ) is given for each criteria ( r ) depending on the specific case ( x ) and desalination technique ( a ).
Here, m is the number of criteria and \(w\) the weight which define how important the criteria is according to the remediation of the aquifer (Table 4 ). Thereby, the sum of all weights has to be 1. The variable t represents the time scenario. We analysed the suitability of the technologies according to current knowledge in the literature for a present scenario (2023) and a future scenario (2043) due to predicted technical and material improvements ( Supplementary Methods ). As shown in the Supplementary References , 160 references were used in order to represent a transparent and non-subjective evaluation. The values and weights are given according to the evaluation standard for three case studies to rate the suitability of the technologies according to their specific conditions.
Data availability
The datasets used and/or analysed during the current study are available in the Supplementary information . Further data are available from the corresponding author on reasonable request.
UN Water. The United Nations World Water Development Report 2022 - groundwater making the invisible visible. (2022).
Bear, J. & Cheng, A. H.-D. Introduction. In: Seawater Intrusion in Coastal Aquifers — concepts, methods and practices (eds. Bear, J., Cheng, A. H.-D., Sorek, S., Ouazar, D. & Herrera, I.) 1–8 (Springer Netherlands, 1999).
Werner, A. D. et al. Seawater intrusion processes, investigation and management: recent advances and future challenges. Adv. Water Resour. 51 , 3–26 (2013).
Article Google Scholar
Walther, M. et al. Assessing the saltwater remediation potential of a three-dimensional, heterogeneous, coastal aquifer system: Model verification, application and visualization for transient density-driven seawater intrusion. Environ. Earth Sci. 72 , 3827–3837 (2014).
Article CAS Google Scholar
Walther, M., Stoeckl, L. & Morgan, L. K. Post-pumping seawater intrusion at the field scale: Implications for coastal aquifer management. Adv. Water Resour. 138 , 103561 (2020).
Casanova, J., Devau, N. & Pettenati, M. Managed aquifer recharge: an overview of issues and options. In: Integrated groundwater management: concepts, approaches and challenges (eds. Jakeman, A. J., Barreteau, O., Hunt, R. J., Rinaudo, J.-D. & Ross, A.) 413–434 (Springer International Publishing, 2016).
Hussain, M. S., Abd-Elhamid, H. F., Javadi, A. A. & Sherif, M. M. Management of seawater intrusion in Ccoastal aquifers: a review. Water 11 , 2467 (2019).
Abd-Elhamid, H. F. & Javadi, A. A. A cost-effective method to control seawater intrusion in coastal aquifers. Water Resour. Manag. 25 , 2755–2780 (2011).
Ebeling, P., Händel, F. & Walther, M. Potential of mixed hydraulic barriers to remediate seawater intrusion. Sci. Total Environ. 693 , 133478 (2019).
Saad, S., Javadi, A. A., Chugh, T. & Farmani, R. Optimal management of mixed hydraulic barriers in coastal aquifers using multi-objective Bayesian optimization. J. Hydrol. 612 , 128021 (2022).
Sadjad Mehdizadeh, S., Badaruddin, S. & Khatibi, S. Abstraction, desalination and recharge method to control seawater intrusion into unconfined coastal aquifers. GJESM 5 , 107–118 (2019).
Abd-Elaty, I., Abd-Elhamid, H. F. & Qahman, K. Coastal aquifer protection from saltwater intrusion using abstraction of brackish water and recharge of treated wastewater: case study of the gaza aquifer. J. Hydrol. Eng. 25 , 05020012 (2020).
Rosentreter, H., Walther, M. & Lerch, A. Partial desalination of saline groundwater: comparison of nanofiltration, reverse osmosis and membrane capacitive deionisation. Membranes 11 , 126 (2021).
Tomaszkiewicz, M., Abou Najm, M. & El-Fadel, M. Development of a groundwater quality index for seawater intrusion in coastal aquifers. Environ. Model. Softw. 57 , 13–26 (2014).
Rhoades, J. D., Kandiah, A. & Mashali, A. M. The use of saline waters for crop production. (Food and Agriculture Organization of the United Nations, Rome, 1992).
Konikow, L. F. & Reilly, T. E. Seawater Intrusion in the United States. In: Seawater intrusion in coastal aquifers — concepts, methods and practices (eds. Bear, J., Cheng, A. H.-D., Sorek, S., Ouazar, D. & Herrera, I.) 463–506 (Springer Netherlands, 1999).
Moore, W. S. & Joye, S. B. Saltwater intrusion and submarine groundwater discharge: acceleration of biogeochemical reactions in changing coastal aquifers. Front. Earth Sci . 9 , 600710 (2021).
Honarparvar, S. et al. Frontiers of membrane desalination processes for brackish water treatment: a review. Membranes 11 , 246 (2021).
Libutti, A. & Monteleone, M. Soil vs. groundwater: the quality dilemma. Managing nitrogen leaching and salinity control under irrigated agriculture in Mediterranean conditions. Agric. Water Manag. 186 , 40–50 (2017).
Weissman, G. et al. Increased irrigation water salinity enhances nitrate transport to deep unsaturated soil. VZJ 19 , e20041 (2020).
Google Scholar
Escalante, E. F., Casas, J. D. H., Medeiros, A. M. V. & Sauto, J. S. S. Regulations and guidelines on water quality requirements for Managed Aquifer Recharge. International comparison. AS/IT JGW 9 , 2 (2020).
Van der Bruggen, B. et al. Electrodialysis and nanofiltration of surface water for subsequent use as infiltration water. Water Res, 37 , 3867–3874 (2003).
WHO Press. Guidelines for drinking-water quality. (Gutenberg, Genf, 2011).
Bloetscher, F., Sham, C. H., Danko III, J. J. & Ratick, S. Lessons learned from aquifer storage and recovery (ASR) systems in the United States. JWARP 06 , 1603–1629 (2014).
Martin, R. Clogging issues associated with managed aquifer recharge methods. IAH commission on managing aquifer recharge 214 (2013).
McDonough, L. K. et al. A new conceptual framework for the transformation of groundwater dissolved organic matter. Nat. Commun. 13 , 2153 (2022).
Dillon, P. et al. Sixty years of global progress in managed aquifer recharge. Hydrogeol. J. https://doi.org/10.1007/s10040-018-1841-z (2018).
Zhang, H., Xu, Y. & Kanyerere, T. A review of the managed aquifer recharge: historical development, current situation and perspectives. Phys. Chem. Earth 118–119 , 102887 (2020).
Bellona, C. Brine management. In: a multidisciplinary introduction to desalination (River Publishers, 2018).
Jones, E., Qadir, M., van Vliet, M. T. H., Smakhtin, V. & Kang, S. The state of desalination and brine production: a global outlook. Sci. Total Environ. 657 , 1343–1356 (2019).
Cao, T., Han, D. & Song, X. Past, present, and future of global seawater intrusion research: a bibliometric analysis. J. Hydrol. 603 , 126844 (2021).
Global Solar Atlas 2.0. https://globalsolaratlas.info/map (2023).
Global Wind Atlas 3.0. https://globalwindatlas.info (2023).
Wang, L., Violet, C., DuChanois, R. M. & Elimelech, M. Derivation of the theoretical minimum energy of separation of desalination processes. J. Chem. Educ. 97 , 4361–4369 (2020).
Plakas, K., Sioutopoulos, D. & Karabelas, A. Energy consumption and minimization. In: A multidisciplinary introduction to desalination (River Publishers, 2018).
Voutchkov, N. Desalination project cost estimating and management. (CRC Press, 2019).
Voutchkov, N. Pretreatment. In: A multidisciplinary introduction to desalination (River Publishers, 2018).
Patel, S. K., Biesheuvel, P. M. & Elimelech, M. Energy consumption of brackish water desalination: identifying the sweet spots for electrodialysis and reverse osmosis. ACS EST Eng. 1 , 851–864 (2021).
Aboutalebi, S. H., Yfantis, A. & Yfantis, N. State-of-the-art desalination research. In: A Multidisciplinary Introduction to Desalination (River Publishers, 2018).
Bazargan, A. & Salgado, B. Fundamentals of desalination technology. In: A multidisciplinary introduction to desalination (ed. Bazargan, A.) 41–92 (IWA Publishing, London, 2018).
Al-Mutaz, I. S. Thermal processes. In: A multidisciplinary introduction to desalination (River Publishers, 2018).
Greenlee, L. F., Lawler, D. F., Freeman, B. D., Marrot, B. & Moulin, P. Reverse osmosis desalination: water sources, technology, and today’s challenges. Water Res. 43 , 2317–2348 (2009).
Wang, L. et al. Water transport in reverse osmosis membranes is governed by pore flow, not a solution-diffusion mechanism. Sci. Adv. 9 , eadf8488 (2023).
Dach, H. Comparison of nanofiltration and reverse osmosis processes for a selective desalination of brackish water feeds. (Université d’Angers, 2008).
Alkhadra, M. A. et al. Electrochemical methods for water purification, ion separations, and energy conversion. Chem. Rev. 122 , 13547–13635 (2022).
Strathmann, H. Electrodialysis, a mature technology with a multitude of new applications. Desalination 264 , 268–288 (2010).
Al-Amshawee, S. et al. Electrodialysis desalination for water and wastewater: a review. J. Chem. Eng. 380 , 122231 (2020).
Oren, Y. Capacitive deionization (CDI) for desalination and water treatment — past, present and future (a review). Desalination 228 , 10–29 (2008).
Zhao, R., Porada, S., Biesheuvel, P. M. & van der Wal, A. Energy consumption in membrane capacitive deionization for different water recoveries and flow rates, and comparison with reverse osmosis. Desalination 330 , 35–41 (2013).
Biesheuvel, P. M. & van der Wal, A. Membrane capacitive deionization. J. Membr. Sci. 346 , 256–262 (2010).
Porada, S., Zhao, R., van der Wal, A., Presser, V. & Biesheuvel, P. M. Review on the science and technology of water desalination by capacitive deionization. Prog. Mater. Sci. 58 , 1388–1442 (2013).
Liu, X., Shanbhag, S., Bartholomew, T. V., Whitacre, J. F. & Mauter, M. S. Cost comparison of capacitive deionization and reverse osmosis for brackish water desalination. ACS EST Eng. 1 , 261–273 (2021).
Shin, Y.-U., Lim, J., Boo, C. & Hong, S. Improving the feasibility and applicability of flow-electrode capacitive deionization (FCDI): Review of process optimization and energy efficiency. Desalination 502 , 114930 (2021).
Zhang, C. et al. Flow electrode capacitive deionization (FCDI): recent developments, environmental applications, and future perspectives. Environ. Sci. Technol. 55 , 4243–4267 (2021).
Houben, G. J., Koeniger, P. & Sültenfuß, J. Freshwater lenses as archive of climate, groundwater recharge, and hydrochemical evolution: Insights from depth-specific water isotope analysis and age determination on the island of Langeoog. Ger. Water Resour. Res. 50 , 8227–8239 (2014).
Costabel, S., Siemon, B., Houben, G. & Günther, T. Geophysical investigation of a freshwater lens on the island of Langeoog, Germany—Insights from combined HEM, TEM and MRS data. Appl. Geophys. 136 , 231–245 (2017).
González, E. et al. Modellierung der küstennahen Grundwasserversalzung in Niedersachsen abgeleitet aus aeroelektromagnetischen Daten. Grundwasser 26 , 73–85 (2021).
Kim, J.-H. et al. Application of cluster analysis for the hydrogeochemical factors of saline groundwater in Kimje, Korea. Geosci. J. 7 , 313–322 (2003).
Ahmed, M. A., Abdel Samie, S. G. & Badawy, H. A. Factors controlling mechanisms of groundwater salinization and hydrogeochemical processes in the quaternary aquifer of the Eastern Nile Delta, Egypt. Environ. Earth Sci. 68 , 369–394 (2013).
Elewa, H. H., Shohaib, R. E., Qaddah, A. A. & Nousir, A. M. Determining groundwater protection zones for the Quaternary aquifer of northeastern Nile delta using GIS-based vulnerability mapping. Environ. Earth Sci. 68 , 313–331 (2013).
Patel, S. K., Qin, M., Walker, W. S. & Elimelech, M. Energy efficiency of electro-driven brackish water desalination: electrodialysis significantly outperforms membrane capacitive deionization. Environ. Sci. Technol. 54 , 3663–3677 (2020).
Hand, S., Guest, J. S. & Cusick, R. D. Technoeconomic analysis of brackish water capacitive deionization: navigating tradeoffs between performance, lifetime, and material costs. Environ. Sci. Technol. 53 , 13353–13363 (2019).
Bales, C. et al. Predictive performance and costing model for Membrane Capacitive Deionization (MCDI) at operational scale. Desalination 557 , 116595 (2023).
Wittholz, M. K., O’Neill, B. K., Colby, C. B. & Lewis, D. Estimating the cost of desalination plants using a cost database. Desalination 229 , 10–20 (2008).
Sajtar, E. T. & Bagley, D. M. Electrodialysis reversal: process and cost approximations for treating coal-bed methane waters. Desalin. Water Treat. 2 , 284–294 (2009).
Werber, J. R., Deshmukh, A. & Elimelech, M. The critical need for increased selectivity, not increased water permeability, for desalination membranes. Environ. Sci. Technol. Lett. 3 , 112–120 (2016).
Patel, S. K., Lee, B., Westerhoff, P. & Elimelech, M. The potential of electrodialysis as a cost-effective alternative to reverse osmosis for brackish water desalination. Water Res. 250 , 121009 (2024).
CAS Google Scholar
Qin, M. et al. Comparison of energy consumption in desalination by capacitive deionization and reverse osmosis. Desalination 455 , 100–114 (2019).
Qin, M. et al. Response to comments on “comparison of energy consumption in desalination by capacitive deionization and reverse osmosis”. Desalination 462 , 48–55 (2019).
Ramachandran, A. et al. Comments on “Comparison of energy consumption in desalination by capacitive deionization and reverse osmosis”. Desalination 461 , 30–36 (2019).
Guyes, E. N., Shocron, A. N., Chen, Y., Diesendruck, C. E. & Suss, M. E. Long-lasting, monovalent-selective capacitive deionization electrodes. npj Clean. Water 4 , 1–11 (2021).
Shocron, A. N., Roth, R. S., Guyes, E. N., Epsztein, R. & Suss, M. E. Comparison of ion selectivity in electrodialysis and capacitive deionization. Environ. Sci. Technol. Lett. 9 , 889–899 (2022).
Ma, J., Zhou, R. & Yu, F. Hotspots and future trends of capacitive deionization technology: a bibliometric review. Desalination 571 , 117107 (2024).
Abdulbaki, D., Al-Hindi, M., Yassine, A. & Abou Najm, M. An optimization model for the allocation of water resources. J. Clean. Prod. 164 , 994–1006 (2017).
Wreyford, J. M., Dykstra, J. E., Wetser, K., Bruning, H. & Rijnaarts, H. H. M. Modelling framework for desalination treatment train comparison applied to brackish water sources. Desalination 494 , 114632 (2020).
Suss, M. E. et al. Water desalination via capacitive deionization: what is it and what can we expect from it? Energy Environ. Sci. 8 , 2296–2319 (2015).
Ishizaka, A. & Nemery, P. Multi‐criteria decision analysis: methods and software. (Wiley, 2013). https://doi.org/10.1002/9781118644898 .
AbdulBaki, D., Mansour, F., Yassine, A., Al-Hindi, M. & Abou Najm, M. Multi-criteria decision making for the selection of best practice seawater desalination technologies. In: Frontiers in water-energy-nexus—nature-based solutions, advanced technologies and best practices for environmental sustainability (eds. Naddeo, V., Balakrishnan, M. & Choo, K.-H.) 489–492 (Springer International Publishing, Cham, 2020). https://doi.org/10.1007/978-3-030-13068-8_122 .
Wencki, K. et al. Approaches for the evaluation of future-oriented technologies and concepts in the field of water reuse and desalination. J. Water Reuse Desalin. 10 , 269–283 (2020).
Download references
Acknowledgements
This research was funded by a European Social Fund (ESF-doctoral scholarship) from the free-state of Saxony, Germany. Further, we acknowledge support by the Open Access Publication Funds of the SLUB/Technische Universität Dresden. The funders played no role in study design, data collection, analysis and interpretation of data, or the writing of this manuscript.
Open Access funding enabled and organized by Projekt DEAL.
Author information
Authors and affiliations.
Chair of Process Engineering in Hydro Systems, Institute of Urban and Industrial Water Management, Department of Hydro Sciences, Faculty of Environmental Sciences, TUD Dresden University of Technology, 01069, Dresden, Germany
Hanna Rosentreter & André Lerch
Chair of Forest Biometrics and Systems Analysis, Department of Forest Sciences, Faculty of Environmental Sciences, TUD Dresden University of Technology, 01069, Dresden, Germany
Marc Walther
You can also search for this author in PubMed Google Scholar
Contributions
Conceptualisation by M.W., A.L., and H.R. H.R. prepared the literature research, calculations and analysed the data. H.R. wrote the manuscript. M.W. and A.L. reviewed and edited the manuscript. All authors read and approved the final manuscript.
Corresponding author
Correspondence to Hanna Rosentreter .
Ethics declarations
Competing interests.
The authors declare no competing interests.
Additional information
Publisher’s note Springer Nature remains neutral with regard to jurisdictional claims in published maps and institutional affiliations.
Supplementary information
Supplementary material, rights and permissions.
Open Access This article is licensed under a Creative Commons Attribution 4.0 International License, which permits use, sharing, adaptation, distribution and reproduction in any medium or format, as long as you give appropriate credit to the original author(s) and the source, provide a link to the Creative Commons licence, and indicate if changes were made. The images or other third party material in this article are included in the article’s Creative Commons licence, unless indicated otherwise in a credit line to the material. If material is not included in the article’s Creative Commons licence and your intended use is not permitted by statutory regulation or exceeds the permitted use, you will need to obtain permission directly from the copyright holder. To view a copy of this licence, visit http://creativecommons.org/licenses/by/4.0/ .
Reprints and permissions
About this article
Cite this article.
Rosentreter, H., Walther, M. & Lerch, A. Assessing the suitability of desalination techniques for hydraulic barriers. npj Clean Water 7 , 40 (2024). https://doi.org/10.1038/s41545-024-00331-8
Download citation
Received : 12 August 2023
Accepted : 19 April 2024
Published : 15 May 2024
DOI : https://doi.org/10.1038/s41545-024-00331-8
Share this article
Anyone you share the following link with will be able to read this content:
Sorry, a shareable link is not currently available for this article.
Provided by the Springer Nature SharedIt content-sharing initiative
Quick links
- Explore articles by subject
- Guide to authors
- Editorial policies
Sign up for the Nature Briefing newsletter — what matters in science, free to your inbox daily.


An official website of the United States government
The .gov means it’s official. Federal government websites often end in .gov or .mil. Before sharing sensitive information, make sure you’re on a federal government site.
The site is secure. The https:// ensures that you are connecting to the official website and that any information you provide is encrypted and transmitted securely.
- Publications
- Account settings
Preview improvements coming to the PMC website in October 2024. Learn More or Try it out now .
- Advanced Search
- Journal List
- Elsevier - PMC COVID-19 Collection

A comprehensive framework for thermoeconomic analysis of desalination systems
Muhammad ahmad jamil.
a Department of Mechanical Engineering, Khwaja Fareed University of Engineering and Information Technology, Rahim Yar Khan, Pakistan
b Mechanical & Construction Engineering Department, Northumbria University, Newcastle Upon Tyne, UK
Muhammad Wakil Shahzad
Syed m. zubair.
c Mechanical Engineering Department, KFUPM Box # 1474 King Fahd University of Petroleum & Minerals, Dhahran 31261, Saudi Arabia
Thermoeconomic analysis, a combined application of thermodynamic and economic analyses, has emerged as an important tool to optimize the performance of desalination systems. Contrary to conventional economic analysis, it offers flexibility to investigate and improve the performance of each component in the system, individually. The current paper presents a comprehensive framework for conducting thermoeconomic analysis of desalination systems. In this regard, different energy calculation methods are discussed first. Then a detailed review of theoretical developments of thermoeconomic analyses is conducted to summarize the correlations/magnitude of important economic parameters. This is followed by a discussion on cost balance equations for important desalination components. Finally, a systematic thermoeconomic analysis model is developed for the mechanical vapor compression desalination system operating under different arrangements as an example. The monetary value of each stream calculated using appropriate fiscal parameters in the system is presented in the form of a cost flow diagram. The study can be used to conduct the thermoeconomic analysis of other commercial desalination systems.
Nomenclature
Greek symbols, superscripts, abbreviations, 1. introduction.
Energy consumption, environmental impacts, and water production cost are the decisive parameters frequently used to investigate the performance of desalination systems [1] , [2] . For the last few years, the research is particularly focused on minimizing the environmental impacts and cost through advancement in materials [3] , [4] , retrofitting of conventional systems [5] , [6] , and development of novel hybrid methods [7] , [8] . The conventional desalination processes involve multi-effect desalination (MED) with evaporation effects [9] , multi-stage flash (MSF) with flashing chambers [10] , mechanical/thermal vapor compression (MVC/TVC) [11] , humidification-dehumidification (HDH) [12] , and reverse osmosis (RO) systems [13] . These systems have been investigated extensively by researchers to discover improvement possibilities [14] . In this regard, the thermoeconomic analysis has emerged as an important tool to assess and improve the thermodynamic and economic performance of desalination systems, simultaneously [15] , [16] , [17] .
The objective of this study is to present a detailed background for thermoeconomic analysis of desalination systems. The novelty of the study lies in its comprehensive presentation of energy calculation methods, summarizing the important capital cost correlations, fiscal parameter ranges, and up-to-date cost balance equations for major desalination system components. All the models introduced in this study can be employed to conduct rigorous thermoeconomic design and analysis of all thermal desalination systems.
The manuscript is structured systematically to make data useful for the readers. In this regard, the first part of the study is focused on the literature review on thermoeconomics of desalination systems, with particular emphasis on thermal desalination systems. The next section presents the input energy calculation methods, followed by discussion and review of important correlations and ranges of fiscal parameters like interest rate, electricity cost, chemical cost, thermal energy cost, and labor cost, etc. The second last section illustrates a comprehensive analysis procedure followed by solved examples for calculation of local and overall water production costs presented in the form of cost flow diagrams. In the end, a road map is suggested based on the findings of the present study and ongoing efforts in the form of different awards for sustainable developments in the future.
2. Literature review
The economic analysis of desalination systems has been widely adopted to estimate, compare, and improve the performance of desalination systems. For example, Madani [18] suggested the use of a solar still, solar-assisted MED, and RO at small scales and MVC, MED and MSF for medium and large scale applications based on economic analysis. Gaggioli et al. [19] applied the Second Law analysis to evaluate the improvement opportunities in the existing systems to minimize exergy destruction and fuel cost. Likewise, El-Mudir et al. [20] studied the performance of a small scale TVC system, and Nafey et al. [21] conducted the thermo-economics of different desalination systems, including MSF, MEE, and MVC system using a custom made visual design and simulation (VDS) software package.
Similarly, thermoeconomic performance analysis of hybrid desalination units coupled with power plants [22] , [23] and energy recovery devices (ERDs) have been conducted by researchers for low water costs. For example, Darwish et al. [24] studied the cost of three different cogeneration power desalting plants and reported the product cost as RO 0.36–0.51 $/m 3 , MVC 0.7–0.97 $/m 3 , and MSF 2.21–3.99 $/m 3 . Manesh et al. [25] performed a component-based exergy-economic optimization of an MSF system coupled with a nuclear reactor and proposed improvement in materials for minimum exergy destruction and cost. Najafi et al. [26] optimized an MSF system coupled with a solid oxide fuel cell (SOFC) driven gas turbine and they found exergetic efficiency, capital cost, and the payback period as 46.7%, M$ 3.76/year and 9 years, respectively under optimum conditions. Alhazmy [27] studied the economic feasibility of a novel MSF desalination with brine-feed mixing and cooling. They reported the breakeven unit product cost from 0.5 to 0.9 $/m 3 . Wang and Lior [28] showed that the coupling of the absorption heat pump (ABHP) improves the thermoeconomic performance of conventional MED systems. Likewise, Esfahani et al. [29] reported the product cost of the MED-ABHP hybrid system could be as low as 0.13 $/m 3 . Sadri et al. [30] investigated the adsorption desalination system with silica gel and found the specific water production cost 0.57 $/m 3 .
Zhang et al. [31] studied the heat pump driven HDH system and reported the product cost 0.0412 $/kg. They also presented that optimization of the system can reduce the water production cost to 0.03846 $/kg [28] . Similarly, Ghofrani and Moosavi et al. [32] compared the exergo-economic performance of three brine recycle BR-HDH systems with, heat-driven (BR-HDH-H), heat pump driven with evaporator after the humidifier (BR-HDH-HP-EAH), heat pump driven with evaporator as dehumidifier (BR-HDH-HP-EAD). They reported the product cost of the mentioned 3 systems as 5.1, 6.2, 10.6 $/m 3 , respectively. Gasmi et al. [33] performed the techno-economic analysis of a large scale SWRO plant integrated with pressure exchanger (PX) and Pelton turbine (PT) as ERDs. They reported a profit of 120,000 (for PX) and 181,000 (for PT) Tunisian Dinars, annually. A similar effect of retrofitting RO plants with ERDs was presented by Penate and Rodriguez [34] and Jamil et al. [35] . The unit water production cost was reported from 0.54 to 0.66 €/m 3 for different retrofits. Djebedjian et al. [36] investigated an actual MVC system at Abu Soma Bay and reported the water production and transportation costs 6.06 and 4.753 EGYP-pounds/m 3 , respectively, based on energetic framework.
In addition to conventional and hybrid systems, some studies have also been focused on investigating the economic feasibility of renewable energy-based systems [37] , [38] , [39] . For instance, Fiorenza et al. [40] examined the economics of solar-driven (SD) desalination plants, including PV-RO and SD-MED, and reported high costs compared to conventional systems. Banat and Jwaied [41] studied a solar-driven membrane distillation (MD) system and reported the membrane and plant life to be the most influential economic parameters. The freshwater cost for small and large scale units was reported as 15 $/m 3 and 18 $/m 3 , respectively. Karagiannis and Soldatos [42] reviewed the product cost of different desalination systems. They reported the range of product cost for conventional methods for seawater from 0.4 to 3 €/m 3 and brackish water from 0.2 to 1.5 €/m 3 . They also found that the arrangements with renewable energy sources can produce water with a cost of reaching up to 15 €/m 3 . Some other recent studies in this regard include life cycle assessment [43] , total revenue requirement analysis [44] , and exergo-environmental analysis [45] .
It is important to emphasize that the water production cost in most of the above-mentioned studies and literature is calculated directly considering the whole plant as a single unit. The total expenses, including equipment, pretreatment, labor, operation, and maintenance costs invested at system boundaries, are divided by the production capacity of the plant to evaluate the unit production cost [9] , [46] . Though the method gives a quick preliminary estimation of the product cost, a component level investigation and optimization are not achievable with this approach. Therefore, thermoeconomic analysis has emerged as an important tool for the detailed design and analysis of desalination systems at the component level.
The method employs thermodynamics and economic analyses simultaneously to assess how efficiently the input resources are utilized throughout the system by each component [47] . This joint application of exergetic analysis and economics can satisfactorily calculate product cost while offering flexibility for design improvement and malfunction diagnosis at the same time. Thus the effect of all endogenous (i.e., irreversibility) and exogenous factors (i.e., fiscal parameters) can be analyzed, individually [48] , [49] . Although this is an important and most accurate tool for desalination processes evaluation, there is no complete framework available in the literature as per author knowledge. Therefore, the novelty of this study is to present a comprehensive framework for thermoeconomic analysis of commercial desalination systems.
3. Input energy calculation
In thermoeconomic analysis, input energy cost is of paramount importance because of its major share in the plant economics, which governs the permeate cost. It depends upon the plant performance, unit energy price, and energy input mechanism. The appropriate calculation/allocation of input energy requires its quantity, quality (thermal, mechanical, or electrical) as well as supply source (i.e., from the grid, steam from a boiler, extracted from a cogeneration plant or waste heat recovery unit). Therefore, a comparison of energy consumption per unit permeate for different desalination systems operating with different types of energy inputs needs all facets to be considered, as shown in Fig. 1 [50] .

Factors affecting input energy calculations [50] .
To address the energy calculation issues, many approaches are presented in the literature such as exergetic analysis [51] , equivalent electricity consumption (to append auxiliary energy inputs) [52] , and universal performance ratio (for appropriate energy allocation in cogeneration water and power systems) [53] , [54] . The application of these methodologies depends on specific parameters and can handle energy calculations satisfactorily across desalination plants. The most appropriate approaches are briefly discussed below.
3.1. Exergy
It is a valuable tool to assess the performance from thermodynamic viewpoint [55] and measures the maximum theoretical useful work that can be obtained from a system as it is brought into a complete thermodynamic equilibrium with the dead state [56] . Darwish et al. [50] used this approach to estimate the exergetic value of each stream in the desalination systems. The specific exergy of a fluid stream with negligible kinetic and potential energies is calculated.
where h and S represent the enthalpy and entropy of the stream and h 0 , S 0 represents the enthalpy and entropy of the stream at dead state ( T 0, P 0 ).
In some cases, the chemical potential of feed and brine also contributes as chemical exergy, and, in those cases, the total specific exergy of a stream is calculated as.
This e ¯ x ¯ che represents the specific chemical exergy calculated about a reasonably selected dead state (usually intake seawater conditions). For calculation of specific exergy of seawater streams, different models have been developed over the years, as discussed by Fitzsimons et al. [57] . Among these models, the seawater functions developed by Sharqawy et al. [58] , [59] are reported to be user-friendly and accurate for a wide range of salinity and temperature. These functions were later updated with pressure dependency by Nayar et al. [60] and are extensively used in the latest studies.
Once the specific amount is known, the total exergy X ( kW ) of each stream is calculated using the respective flow rate m ˙ ( kg/s ) as.
Finally, the specific energy consumption (SEC) in kWh/m 3 of the system is calculated as,
where W ˙ is the work input to the plant in kW and m ˙ D the mass flow rate of the product in kg/s.
3.2. Equivalent electricity consumption
The equivalent electricity consumption ( E eq ) measures the amount of electricity that could have been produced using thermal energy, which was bled to a desalination system [52] . It is used to rationally compare the performance of thermal and electricity-driven desalination systems. Also, the ( E eq ) is used to reasonably append the auxiliary energy in the total energy consumption of the system i.e., the electricity consumption of pumps in steam-driven MED and MSF systems. Likewise, in MVC systems, the makeup steam used can also be converted into equivalent electricity consumption to estimate the SEC. The electrical work is calculated, assuming that the steam used in the desalination plant was instead expanded in a steam turbine, as discussed by Naryan et al. [61] .
where η gen is the efficiency of the electrical generator, and it is assumed 95% [52] , [61] . The exit stream temperature is taken to be 35 °C, and the isentropic efficiency of 85% is assumed for the steam turbine [52] , [61] . The equivalent electricity consumption is calculated as.
where W ˙ S represents the turbine work produced in kW , m ˙ D is the distillate flow rate in kg/s and 3.6 is the conversion factor from kJ/kg to kWh/m 3 taking water density 1000 kg/m 3 .
3.3. Universal performance ratio
Shahzad et al. [62] proposed the universal performance ratio (UPR) method to evaluate the desalination processes on a common platform based on primary energy consumption. The method is particularly useful for cogeneration plants that face unfair primary fuel cost apportionment to electricity and desalination. Using the UPR method the authors investigated that in a cogeneration combined cycle power and desalination plant, the gas turbine was undercharged by 40%, the steam turbine was overcharged by 71% and desalination was overcharged by 350% by conventional energetic apportionment methods. They suggested that the use of exergetic analysis for primary fuel percentage apportionment to all components in the cycle according to the quality of working fluid utilized on a large scale exterminate this issue.
In contrast to conventional performance ratio (PR) formula (given in Eq. (7) ) which is based on derived energy and does not accommodate the quality (work potential) of different derived energies, a new UPR was proposed. In the proposed UPR (given in Eq. (8) ), the derived energies are multiplied with respective conversion factors (CF) to convert into primary energies; those can be then added to calculate total input to desalination process. The conversion factors are calculated based on the exergy destruction across the components corresponding to primary fuel exergy.
The above discussion asserts that the exergy (available energy) gives the real picture of plant input energy. Therefore, the framework presented in the later sections of this paper is based on the calculation of exergetic cost of each fluid stream as it enters or leaves any component in the desalination plant.
4. Parameters of thermoeconomic analysis
The product cost of desalination systems depends upon a wide range of economic parameters as shown in Fig. 2 [63] , [64] . However, in thermoeconomic analysis, the main targeted economic parameters are capital expenditure (CAPEX), energy cost, fiscal parameters, operation, and maintenance cost a detailed explanation of these parameters is presented in the subsequent sections.

Summary of critical economic parameters [64] .
4.1. Capital cost
The thermoeconomic analysis starts with the provision/ calculation of capital cost (Z) of each component of the system. It generally reflects the component’s purchasing cost; however, in some cases, the commissioning and outset operation and maintenance costs are also included [65] . The most reliable option to obtain Z is through the market survey or supplier’s quotations as it fluctuates with location, the distance of the delivery site, local taxes, and availability of alternatives. However, the cost obtained by this method is only suitable for specified conditions and does not offer design flexibility.
Therefore, to accommodate these variations in the equipment cost, different correlations have been developed that can satisfactorily approximate the equipment cost [66] . These correlations are modeled as a function of design parameters such as mass flow rate, heat transfer rate, duty, efficiency, energy consumption, pressure, and temperature differentials [67] , [68] , [69] . The correlations for the capital cost of common desalination system equipment are presented in Table A.1 of the appendix.
4.2. Cost index factor
The cost index factor ( C index ) is used to escalate the capital cost of equipment from the original year to the current year in correlation development [70] . For instance, the capital cost of equipment calculated in 2019 using a correlation proposed 10–20 years back cannot reliably predict the required expenses [71] . Therefore, it is recommended to escalate the capital cost to the current year using cost indices that accommodate the inflation and variations in market scenarios that happened over the years [72] . For this purpose, a systematic methodology is used to calculate the C index using the Chemical Engineering Plant Cost Index (CEPCI) of the reference year and the current year, as given below [73] .
Then the current capital cost of the equipment is given as [74] ,
For understanding, C index is 1.7 for a correlation developed in 1990 as CEPCI @1990 is 390 [75] , and CEPCI @2020 is 650 [76] . However, for rigorous design and analysis purposes, the effect of C index is studied for a wide range of values, as presented in the study [15] .
4.3. Capital recovery factor
The capital recovery factor (CRF) defines the annuities in terms of money transacted out of a present value after a defined period throughout the economic life of the equipment [21] . It is used to estimate the annual rate of capital investment Z ˙ ( $ / y r ) of equipment or a plant using interest rate ( i ) and amortization years/economic life of the plant ( y ) as given by Esen et al. [77] :
Finally, the rate of fixed cost ζ ( i n $ / s ) is calculated based on the plant availability Λ as [78] ,
4.4. Cost balance equations
In thermoeconomic analysis, each component of the plant is investigated as a system with its local inputs, outputs, and fixed cost. For this purpose, a cost balance equation relating to the rate of expenditures (inputs) to the products (output) is applied to each component as given below [13] .
where C ˙ o represents the cost of the local output stream, C ˙ i the cost of the input stream, and ζ the rate of capital cost.
For the systems with inclusive operation and maintenance costs, the above equation is written as [79] .
It is important to mention that for the components with a single outlet stream (i.e., pumps, compressors, blowers, etc.), the cost balance equation can be solved by providing the inlet costs. While for the components with multiple outlet streams (i.e., heat exchangers, evaporators, flash chambers and membrane modules, etc.), additional auxiliary equations are required. For a system with “k” outlet streams, “k − 1” number of auxiliary equations are required to solve the system [80] . These equations are based on the equality of the average cost of inlet and outlet streams and are of the form as given in Eq. (16) [51] .
The cost balance equations for commonly used desalination system components and the corresponding auxiliary equations are presented in the following sections.
4.4.1. Pump
Pumps are used to maintain pressure and flow rate of different water streams, including feed, permeate, and brine. In membrane-based systems (particularly RO) pumps have a significant share in the total plant cost because of high pumping pressures (≥6000 kPa) that require robust size and high energy input. Contrarily, in thermal-based systems, pumps maintain pressure merely to overcome the pressure drops in pipes, heat exchangers and evaporators. As the pressure in these systems is slightly above atmospheric pressure (≤500 kPa), their energy consumption and cost do not contribute significantly. Resultantly, in most of the thermally driven desalination processes, the energy consumption of pumps is either ignored or calculated under auxiliary energy inputs, as mentioned above (refer 2.2). However, for a detailed and accurate analysis, a complete plant layout is to be considered (including pumps). Fig. 3 demonstrates the cost flow diagram of pumps, and the corresponding cost balance equation is given in Eq. (17) .

Process flow diagram for the pump.
4.4.2. Compressor
A compressor is an integral part of mechanical vapor compression based thermal desalination systems. It is used to increase the temperature and pressure of water vapors produced during the desalination process. These compressed vapors are used to desalinate the subsequent feed water streams. The process offers two major benefits including flexibility to operate on electrical energy (suitable for remote areas) and a significant reduction in intake steam flow rate which abates the demand of a robust steam generation facility. Therefore, in such systems compressor is a major energy-consuming (~95%) device and thus have a major share in plant economics. Fig. 4 illustrates the cost-flow diagram of a vapor compressor and the corresponding cost balance is given in Eq. (18) .

Process flow diagram for the compressor.

4.4.3. Membrane module
It is the main component of RO systems and constitutes almost 60% of the total plant cost. The feedwater is pumped to the membrane module using a high-pressure pump (HPP) where the freshwater is extracted from the feed stream, and the remaining is rejected as brine (refer to Fig. 5 ). The economics of the membrane module mainly depends upon its capacity, pore size, and pressure withstanding capability, which are controlled by the feed concentration. The cost balance equation for the membrane module is given in Eq. (19) .

Process flow diagram for membrane module.
It is important to emphasize that the module has one inlet (feed) and two outlet streams (brine and permeate), so an auxiliary equation is required to solve this equation, which is given below.
4.4.4. Preheater
In thermal desalination systems, preheaters are used to heat the intake seawater to the required feed temperature by recovering heat from the brine and distillate streams. For this purpose, plate heat exchangers are preferred because of close temperature control and easy maintenance [81] . The flow diagram and cost balance equation for preheaters (heat exchangers) are given in Fig. 6 and Eq. (21) , respectively.

Process flow diagram for heat exchanger/preheater.
The auxiliary equation required to solve this balance equation is given as:
4.4.5. Evaporator
In evaporation based thermal desalination systems, a major portion of the total energy interactions and cost is concentrated in the evaporation section. This unit defines the plant capacity, operating parameters as well as capital expenses. In the desalination industry, horizontal tube falling film evaporators are generally preferred because of better thermal performance compared to other counterparts. In these evaporators, a thin film of feedwater is sprayed over the evaporator tubes, which vaporizes by taking heat from the steam inside the tubes, as shown in Fig. 7 . In single-effect systems, these vapors are condensed in a condenser as a distillate, but in multi-effect systems, these are used as steam in the subsequent effects. The cost balance equation for the evaporator is given in Eq. (23) and the auxiliary equations are given in Eqs. (24) , (25) .

Process flow diagram for an evaporative-condenser [82] .
4.4.6. Flash chamber
In flashing-based thermal-desalination systems, e.g., MSF, the flash chambers are used to produce vapors. First, the intake seawater is heated to the feed temperature by passing through the condenser tubes. It is then fed to the brine pool at the bottom of the chamber, where it vaporizes partly and the remaining leaves as brine, which serves as a feed for subsequent effects. The flashed vapors pass through a demister and are collected as condensate in the distillate tray after exchanging heat with the intake seawater. The distillate stream (from previous effects) is mixed with condensate and is collected at the outlet, as shown in Fig. 8 . The cost balance equation for the flash chamber is given as [21] .

Process flow diagram for the MSF flash chamber.
The two auxiliary equations required to solve this cost balance are given in Eq. (27) , (28) .
4.4.7. Humidifier and dehumidifier
In HDH systems, the feed water is sprayed in a humidifier where it humidifies the air using the evaporative potential of air. The moist air from the humidifier is directed to the dehumidifier, where it condenses by exchanging heat with the intake seawater, as shown in Fig. 9 , Fig. 10 . The cost balance equations and auxiliary equations for a humidifier are given in Eqs. (29) , (30) and for a dehumidifier in Eqs. (31) , (32) given below [83] .

Process flow diagram of the humidifier.

Process flow diagram of the dehumidifier.
It is important to mention that in addition to the above-discussed parameters, some other indicators like payback period, Levelized cost, etc. have also been used by some researchers to illustrate the economics of desalination systems. These indicators are not an intrinsic part of thermoeconomic analysis and are rarely used. However, they provide valuable information from a monetary viewpoint. A brief overview of these economic indicators is presented in the appendix.
Besides, a detailed review of existing studies on thermoeconomic analysis of desalination technologies is conducted to summarize the range of important fiscal parameters as presented in the appendix. Table A.2 shows that water production cost varies remarkably with location, technology, and time. The prime reasons for fluctuations in the prices are the variations in system performance and fiscal parameters. The technological advancements and development of better materials have considerably reduced the energy consumption of desalination systems, thus providing the potential for cutting the operational expenses. However, the associated research and development expenses, inflation in material costs, hikes in energy prices, and strict environmental legislations have imposed additional monetary overheads.
It is important to mention that the calculation of water production cost (presented above) is a multifaceted procedure and necessitates a systematic approach for the calculation of required parameters. Keeping in view, a component-based comprehensive theoretical framework calculating local and overall water production cost, is presented in the subsequent section. For this purpose, a technically sophisticated desalination system, i.e., Mechanical Vapor Compression Desalination System working under different operating scenarios, is selected as an example. The system demonstrates all major thermal, hydraulic, and thermodynamic processes involved in the conventional desalination systems. Therefore, the developed model can be applied to other systems with minor relevant modifications.
5. A sample thermoeconomic model
Based on the earlier presented capital cost correlations, cost balance equations, and fiscal parameters, a comprehensive thermoeconomic model is developed for MVC systems, as an example. Different operating arrangements are considered for a complete plant layout. Firstly, the exergy analysis is conducted to calculate the flow exergy (kW) of all the streams, including feed, distillate, brine, and vapors. At the second stage, exergy destruction in each component is calculated to assess the thermodynamic performance. Lastly, the cost balance equations are employed to calculate the monetary cost of all the streams using exergetic cost-based auxiliary equations.
5.1. Single effect MVC system
A single effect MVC system consists of an evaporator, a compressor, pumps, and two preheaters. The governing equations for thermoeconomic analysis of SEE-MVC are summarized in Eqs. (33)-(47) [15].
where, C ˙ misc is the miscellaneous cost such as blowdown, cooling, condensate, chemical, post-treatment, etc.
The thermoeconomic analysis for the SEE-MVC system is conducted using the above-presented model. The important thermodynamic and economic parameters used in the analysis are summarized in Table 1 . The cost-flow diagram presenting the cost rate (in $/h) at each component’s outlet is presented in Fig. 11 [15] , [84] .
Input data for thermoeconomic analysis [15] , [85] .

Single effect MVC system diagrams (a) process flow and (b) cost flow [15] , [84] .
5.2. Multi-effect MVC systems
The multi-effect MVC systems use many evaporation effects (normally 2 to 10) to desalinate feed water. The vapor produced in each evaporator is used as a heat source for subsequent effects. Finally, the vapors from the last effect are directed to a vapor compressor where they are compressed and superheated to serve as a heat source for the first effect. However, in some high capacity systems, makeup steam is also added with the compressed vapor to reduce the compressor size, energy consumption, and cost. It is worth mentioning that the multi-effect systems can operate in different feed flow arrangements (see Fig. 12 ), which have different operating conditions, energy requirements, and product costs for the same capacity. The thermoeconomic models for three commonly used flow configurations are presented below:

Multi-effect MVC system operating under (a) forward feed, (b) parallel feed, and (c) parallel crossfeed [84] , [85] .
5.2.1. Forward feed (FF)
In this arrangement, the total feed is sprayed in the first evaporator, and the brine of each evaporator serves as a feed for the next effects. Finally, the brine from the last effect is directed to the brine preheater to preheat the intake seawater. The cost balance equation is generalized in terms of the number of evaporators which can vary as j = 2 to N. The governing equations in the general form are given as [82] :
For the first effect [j = 1], the vapor and feed stream costs are calculated as:
For [j = 2 to N]
5.2.2. Parallel feed (PF)
In this configuration, the feed is equally disbursed in all the evaporators at feed temperature. The brine from all effects is collected and sent to the brine preheater and the remaining operation same as that of forward feed. The general form of equations for calculation of different costs are given below [85] .
5.2.3. Parallel crossfeed (PCF)
In this arrangement, the feed is equally distributed in all the effects like PF; however, brine from each effect is introduced in the next effect at the bottom. The addition of brine in the subsequent effects results in addition to vapor production with flashing because of the difference in temperature and pressure. The governing cost equations are given below [85] .
It is important to emphasize that the feed flow arrangements discussed above have their advantages and limitations from thermodynamic and economic viewpoints, which have been investigated in detail by Jamil et al. [84] , [85] . The cost flow diagram for 4-effects MVC systems operating under the above-discussed arrangements is presented in Fig. 13 .

Cost flow diagram of a multi-effect MVC system [83,84].
6. Discussion of results
The thermoeconomic analysis conducted above presented the cost rate (in $/h) and water production cost (in $/m 3 ) for different MVC system configurations. For example, in a single effect system, the feed is assigned a cost rate of 4.6 $/h at a preheater inlet because of pretreatment. After being heated to feed temperature (at evaporator inlet) from the intake seawater temperature, the cost of stream surged to 795 $/h because of the capital cost of preheaters and the hot water stream costs coming from the evaporator. The cost of vapors produced in the evaporator is calculated as 5216 $/h, which increased to 5274 $/h at the compressor outlet because of the addition of compressor fixed cost as well as input energy cost. Finally, the distillate and brine stream costs after releasing its heat in the preheater are calculated as 94 $/h and 33 $/h, respectively. The water production cost for this system with a distillate capacity of 13 kg/s is calculated as 1.7 $/m 3 .
Similarly, for multi-effect systems, the pretreated intake seawater cost is taken the same as 4.61 $/h for all the feed flow arrangements. However, the preheaters are different in size for all three systems because of different inlet and outlet temperatures; therefore, the feed water cost is calculated as 338 $/h, 122 $/h, 116 $/h for FF, PF and PCF thus showing the largest investment in the case of FF. This is because of the reason that in FF, the total feed is sprayed in the first evaporator, which has significantly higher evaporation temperature requirements (i.e., 47 °C) than PF and PCF (i.e., 36 °C). The distillate cost at the outlet of evaporator and compressor section is calculated as 316 $/h, 156 $/h, and 183 $/h, and the corresponding brine stream costs are 117 $/h, 62 $/h, and 32 $/h. Finally, the water production cost is calculated as the highest for FF with 0.867 $/m 3 , followed by PCF with 0.865 $/m 3 and PF with 0.842 $/m 3 . Therefore, the analysis concluded that the single effect system has the highest water production cost, followed by FF, PCF, and PF, respectively.
7. Closing remarks and future road map
The current study presents a comprehensive theoretical framework for a component-based thermoeconomic analysis of commercial-scale desalination systems. The key economic parameters that govern the analysis and water product cost are discussed in detail. A comprehensive review of existing studies is conducted to cluster the magnitude of critical fiscal parameters. The cost balance equations are developed for commonly used desalination system components. Finally, a detailed mathematical procedure is developed for thermoeconomic analysis of desalination systems using one of the most panoptic and sophisticated systems, i.e., MVC. The proposed procedure can be satisfactorily used to investigate the thermoeconomic performance of other desalination systems as well.
In conclusion, an extensive review and assessment of literature reveal that despite lots of improvement efforts, the leveling-off water production cost for existing desalination systems is hovering around 0.9 ± 0.3 $/m 3 (refer Fig. 14 ). Most of the developing countries are unable to pay such a high cost, where the average salary is close to $1–2/day [86] . Hence, for a quantum decrease in water production cost, a technological breakthrough is essential that can address the key limitations like operational limits (~0.78 kWh/m 3 ), material properties, brine management, monetary expenses, and energy consumption, etc., simultaneously. For affordable water supply to all developing countries, the estimated operational cost must be closer to 0.3 ± 0.05 $/m 3 [87] . In this regard, some noticeable efforts are underway such as Global Water Award (SUQIA UAE) [88] , the Water Challenge (Water Aid Australia) [89] , Global Water Awards [90] , the IWA Global Water Award [91] and the IDA Water Awards [92] to motivate water experts for innovative solutions to reduce the water cost.

The leveling-off water production cost of existing desalination technologies and future roadmap.
The overall target is to achieve low cost and sustainable water supply zone, as shown in Fig. 14 . Based on recent COVID-19 global pandemic experience when US oil prices dropped below zero, water has emerged as the new oil, and its importance is endorsed in every sector of life. To cope this kind of uncertain situation in the future, water security, and low-cost supply are the most important. So, it is very timely to investigate out-of-box solutions for uninterrupted and low-cost water supply to save lives.
Declaration of Competing Interest
The authors declare that they have no known competing financial interests or personal relationships that could have appeared to influence the work reported in this paper.
Acknowledgments
The authors acknowledge the support provided by the Mechanical Engineering Department, Khwaja Fareed University of Engineering, and Information Technology (KFUEIT), Rahim Yar Khan. In particular, Dr. Muhammad Wakil Shahzad acknowledges the support provided by Northumbria University, UK under reference # RDF20/EE/MCE/SHAHZAD. Also, Syed Zubair would like to acknowledge the support received from King Fahd University of Petroleum & Minerals (KFUPM) through the project IN171048.
A-1. Additional economic indicators
The following additional economic indicators are also used in the literature to present the economic performance of desalination systems.
Payback period
It refers to the period in which total capital investment is recovered by selling the product [79] . The equations for calculation of payback period is given as [26] .
where, “ E c,p ” represents the worth of the plant in “ p th ” year of operation with “ Z ” as capital cost, “ i ” as interest rate, “ C f ” as fuel cost rate, “ p ” as the payback period, and “ N ” as operational hours per year.
Levelized cost
It measures and compares the cost of different alternative systems giving the same output. It gives the real price for long term projects in net present value in terms of Levelized Capital Cost (LCC) and Levelized Operational Cost (LOC). The term Levelized asserts that these costs are presented in the form of a series of equivalent payments [93] .
where LCC is given by
Here, “ K t ” is the capital expense ($) accruing in the year “ t ”, “ V ˙ t ” is distillate amount (m 3 ) in “ t ”, “ r ” is the required rate of return, and “ y ” is the amortization period.
Likewise, the LOC is calculated in terms of operational cost “ O t ($)” accruing in year “ t ” as.
In addition to LCC and LOC, another parameter called Constant Escalation Levelization Factor (CELF) is also calculated. It gives a reliable estimate of the present value of a system, and is given as [13] , [79] .
where CRF is the capital recovery factor, “ £ ” is economic life, and “ κ ” is constant and calculated as.
In the above equation “ r n ” is the nominal escalation rate of energy and O&M costs with time and “ i eff ” is the effective discount rate.
A-2. Summary of various cost elements
The capital cost of different desalination system components is calculated using the correlations that are summarized in Table A1 , whereas the ranges of important fiscal parameters and the product cost are described in Table A2 .
Correlations for capital cost.
where, all values are in SI units i.e. Q ˙ ( kW ), A(m 2 ), T i ( K ), Δ T (°C), P (kPa), m ˙ (kg/s), W ˙ (kW), r P = P o / P i , e = η / 1 - η ,
IF: Installation factor for PHX ranging 1.5–2.0 [98]
---: not mentioned.
Ranges of economic parameters used by different authors.
i = interest rate, £ = contractual period/plant life, Γ = maintenance cost, Ω = labor cost, Φ = pretreatment/ chemical, ε = unit electricity cost, δ = unit steam cost, Λ = plant availability factor, γ = annual rate of membrane replacement, α = amortization factor, λ = inflation rate, y = amortization period, C index = cost index factor, Π = intake cost, C f = fuel cost, β = brine management.
The Infona portal uses cookies, i.e. strings of text saved by a browser on the user's device. The portal can access those files and use them to remember the user's data, such as their chosen settings (screen view, interface language, etc.), or their login data. By using the Infona portal the user accepts automatic saving and using this information for portal operation purposes. More information on the subject can be found in the Privacy Policy and Terms of Service. By closing this window the user confirms that they have read the information on cookie usage, and they accept the privacy policy and the way cookies are used by the portal. You can change the cookie settings in your browser.
- Login or register account
INFONA - science communication portal
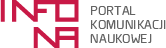
- advanced search
- conferences
- Collections
Water desalination cost literature: review and assessment $("#expandableTitles").expandable();
- Contributors
Fields of science
- Bibliography
Desalination > 2008 > 223 > 1-3 > 448-456
Identifiers
User assignment, assignment remove confirmation, you're going to remove this assignment. are you sure.
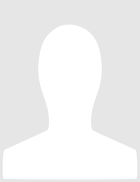
Ioannis C. Karagiannis
Petros g. soldatos.
Desalination methods Brackish Seawater Hybrid systems Renewable energy sources Desalination tools
Additional information

- Read online
- Add to read later
- Add to collection
- Add to followed

Export to bibliography

- Terms of service
Accessibility options
- Report an error / abuse
Reporting an error / abuse
Sending the report failed.
Submitting the report failed. Please, try again. If the error persists, contact the administrator by writing to [email protected].
You can adjust the font size by pressing a combination of keys:
- CONTROL + + increase font size
- CONTROL + – decrease font
You can change the active elements on the page (buttons and links) by pressing a combination of keys:
- TAB go to the next element
- SHIFT + TAB go to the previous element
Climate change vulnerability of Kuwait: a cross-sectoral assessment
- Original Paper
- Published: 20 May 2024
- Volume 17 , article number 183 , ( 2024 )
Cite this article
- Mohammad M. M. Alsahli ORCID: orcid.org/0000-0003-3225-7452 1 &
- Dhary S. Alkandary ORCID: orcid.org/0000-0001-9491-9816 2
Climate change is expected to be severe in many countries, mainly in arid countries such as Kuwait. Thus, assessing the vulnerability of these countries is fundamental in designing mitigation and adaptation plans. In this study, a qualitative approach was used to conduct a climate change vulnerability assessment (CCVA) targeting Kuwait’s most impacted sectors: marine and fisheries, water resources, land management, and health. Increasing sea surface temperature and seawater salinity significantly affect the marine and fisheries sectors; the consequences are very complicated and interconnected with water resources. Mitigation and adaptation options to these stressors are minimal. In the land management sector, establishing adaptive land-use policies will minimize the impact of sea-level rise, land degradation, and floods. Increasing dust storm events and heat waves have a diverse effect on public health. The CCVA framework efficiently revealed the interrelationships among the studied sectors and can be applied to design CCVAs for other countries.
This is a preview of subscription content, log in via an institution to check access.
Access this article
Price includes VAT (Russian Federation)
Instant access to the full article PDF.
Rent this article via DeepDyve
Institutional subscriptions

References
Abahussain AA, Abdu AS, Al-Zubari WK, El-Deen NA, Abdul-Raheem M (2002) Desertification in the Arab region: analysis of current status and trends. J Arid Environ 51(4):521–545. https://doi.org/10.1006/jare.2002.0975
Article Google Scholar
Abotalib M, Jacob J, Alhamadi H, Alkandari D (2021) The environmental life cycle assessment of different electricity options in Kuwait. J Eng Res [Internet] [accessed 2022 Nov 29] 9(2):308–319. https://doi.org/10.36909/jer.v9i2.9853
Article CAS Google Scholar
Achilleos S, Al-Ozairi E, Alahmad B, Garshick E, Neophytou AM, Bouhamra W, Yassin MF, Koutrakis P (2019) Acute effects of air pollution on mortality: a 17-year analysis in Kuwait. Environ Int 126:476–483. https://doi.org/10.1016/J.ENVINT.2019.01.072
Al-Awadhi JM, Misak R (2000) Field assessment of aeolian sand processes and sand control measures in Kuwait. Kuwait J Sci Eng 27(1):158–176
Google Scholar
Al-Awadhi JM, Omar SA, Misak RF (2005) Land degradation indicators in Kuwait. L Degrad Dev 16(2):163–176. https://doi.org/10.1002/ldr.666
Al-Damkhi AM, Khuraibet AM, Al-Attar FAH, Abdul-Wahab SA (2008) Integrating environmental impact assessment within Kuwait master plans as a tool for human and ecological risk control. Hum Ecol Risk Assess. https://doi.org/10.1080/10807030802387846
Al-Dousari A, Doronzo D, Ahmed M (2017) Types, indications and impact evaluation of sand and dust storms trajectories in the Arabian Gulf. Sustain 9(9):1–14. https://doi.org/10.3390/su9091526
Al-Dousari AM, Alsaleh A, Ahmed M, Misak R, Al-Dousari N, Al-Shatti F, Elrawi M, William T (2019) Off-road vehicle tracks and grazing points in relation to soil compaction and land degradation. Earth Syst Environ 3(3):471–482. https://doi.org/10.1007/s41748-019-00115-y
Al-Dousari A, Ramadan A, Al-Qattan A, Al-Ateeqi S, Dashti H, Ahmed M, Al-Dousari N, Al-Hashash N, Othman A (2020) Cost and effect of native vegetation change on aeolian sand, dust, microclimate and sustainable energy in Kuwait. J Taibah Univ Sci [Internet] 14(1):628–639. https://doi.org/10.1080/16583655.2020.1761662
Al-Harbi M, Eidan H, Al-Holan N, Al-Abdullrazaq F, Alsahli M, Hassan A, Altheyabi N, Ramadan E, Aldashti H, Alnassar M, Aldousiri A, Alhazeem S, Bahzad J, Gholoum M, Alshatti A, Lujain Saidan M, Hussein S (2018) The second national communication report: vulnerability and adaptation to climate change. Kuwait Environmental Public Authority
Al-Hemoud A, Gasana J, Al-Dabbous AN, Al-Shatti A, Al-Khayat A (2018) Disability adjusted life years (Dalys) in terms of years of life lost (yll) due to premature adult mortalities and postneonatal infant mortalities attributed to pm2.5 and pm10 exposures in Kuwait. Int J Environ Res Public Health 15(11):1–15. https://doi.org/10.3390/ijerph15112609
Al-Hemoud A, Gasana J, Al-Dabbous A, Alajeel A, Al-Shatti A, Behbehani W, Malak M (2019) Exposure levels of air pollution (PM2.5) and associated health risk in Kuwait. Environ Res 179:108730. https://doi.org/10.1016/J.ENVRES.2019.108730
Al-Hemoud A, Al-Enezi A, Al-Dashti H, Petrov P, Misak R, AlSaraf M, Malek M (2023) Hazard assessment and hazard mapping for Kuwait. Int J Disaster Risk Sci [Internet] [accessed 2023 Jun 8] 14(1):143–161. https://doi.org/10.1007/S13753-023-00473-2/FIGURES/12
Al-Husaini M, Bishop JM, Al-Foudari HM, Al-Baz AF (2015) A review of the status and development of Kuwait’s fisheries. Mar Pollut Bull 100(2):597–606. https://doi.org/10.1016/j.marpolbul.2015.07.053
Al-Muqdadi SWH (2019) Developing strategy for water conflict management and transformation at Euphrates–Tigris basin. Water 11:2037. https://doi.org/10.3390/W11102037
Al-Mutairi N, Alsahli M, El-Gammal M, Ibrahim M, Samra RA (2021) Environmental and economic impacts of rising sea levels: a case study in Kuwait’s coastal zone. Ocean Coast Manag [Internet] 205:105572. https://doi.org/10.1016/j.ocecoaman.2021.105572
Al-Qallaf H, Aliewi A, Abdulhadi A (2020) Assessment of the effect of extreme rainfall events on temporal rainfall variability in Kuwait. Arab J Geosci 13(21). https://doi.org/10.1007/s12517-020-06086-z
Al-Rashidi TB, El-Gamily HI, Amos CL, Rakha KA (2009) Sea surface temperature trends in Kuwait Bay, Arabian Gulf. Nat Hazards. https://doi.org/10.1007/s11069-008-9320-9
Al-Said T, Al-Ghunaim A, Subba Rao DV, Al-Yamani F, Al-Rifaie K, Al-Baz A (2017) Salinity-driven decadal changes in phytoplankton community in the NW Arabian Gulf of Kuwait. Environ Monit Assess 189(6). https://doi.org/10.1007/s10661-017-5969-4
Al-Shayji K, Aleisa E (2018) Characterizing the fossil fuel impacts in water desalination plants in Kuwait: a life cycle assessment approach. Energy 158:681–692. https://doi.org/10.1016/J.ENERGY.2018.06.077
AL-Yamani F, Bishop J, Ramadhan E, AL-Husaini M, Al-Ghadban AN (2004) Physico-chemical Characteristics and Biological Oceanography of Kuwait’s Waters. In Oceanographic Atlas of Kuwait’s Waters. Kuwait Institute for Scientific Research, pp 93–95
Al-Yamani F, Polikarpov I, Saburova M (2021) In: Jawad LA (ed) Northern Gulf marine biodiversity in relevance to the river discharge BT - Southern Iraq’s marshes: their environment and conservation [Internet]. Springer International Publishing, Cham, pp 379–437. https://doi.org/10.1007/978-3-030-66238-7_20
Al-Zubari W, Al-Turbak A, Zahid W, Al-Ruwis K, Al-Tkhais A, Al-Muataz I, Abdelwahab A, Murad A, Al-Harbi M, Al-Sulaymani Z (2017) An overview of the GCC unified water strategy (2016–2035). Desalin Water Treat 81:1–18. https://doi.org/10.5004/dwt.2017.20864
Alahmad B, Shakarchi AF, Khraishah H, Alseaidan M, Gasana J, Al-Hemoud A, Koutrakis P, Fox MA (2020) Extreme temperatures and mortality in Kuwait: who is vulnerable? Sci Total Environ 732:139289. https://doi.org/10.1016/j.scitotenv.2020.139289
Albahar S, Li J, Al-Zoughool M, Al-Hemoud A, Gasana J, Aldashti H, Alahmad B (2022) Air pollution and respiratory hospital admissions in Kuwait: The Epidemiological Applicability of Predicted PM2.5 in Arid Regions. Int J Environ Res Public Health 19(10). https://doi.org/10.3390/ijerph19105998
Aldosari D, Almedeij J, Alsumaiei AA (2020) Update of intensity–duration–frequency curves for Kuwait due to extreme flash floods. Environ Ecol Stat [Internet] 27(3):491–507. https://doi.org/10.1007/s10651-020-00454-4
Aldousari EA, Alsahli MMM (2017) Studying the spatial distribution of asthma patients in the state of Kuwait using GIS. J Soc Sci 45(1):11–35
Alghais N (2019) Evaluation of the impacts of lack of geoinformation data in crisis management during the 2018 Kuwait flood. Int Arch Photogramm Remote Sens Spat Inf Sci - ISPRS Arch 42(3/W8):17–24. https://doi.org/10.5194/isprs-archives-XLII-3-W8-17-2019
Alghais N, Pullar D (2018) Modelling future impacts of urban development in Kuwait with the use of ABM and GIS. Trans GIS 22(1):20–42. https://doi.org/10.1111/tgis.12293
Aliewi A, Alomirah H (2020) Assessment of the significance of water-energy-food nexus for Kuwait BT. In: Kumar M, Munoz-Arriola F, Furumai H, Chaminda T (eds) Resilience, response, Risk Water Syst shifting manag nat Forcings paradig [Internet]. Springer Singapore, Singapore, pp 357–367. https://doi.org/10.1007/978-981-15-4668-6_19
Chapter Google Scholar
Aliewi A, El-Sayed E, Akbar A, Hadi K, Al-Rashed M (2017) Evaluation of desalination and other strategic management options using multi-criteria decision analysis in Kuwait. Desalination 413:40–51. https://doi.org/10.1016/j.desal.2017.03.006
Aliewi A, Al-Kandari J, Al-Khalid A, Bhandary H, Al-Qallaf H (2021) Modelling the effect of high level of total dissolved solids (TDS) for the sustainable utilization of brackish groundwater from saline aquifers in Kuwait. Environ Dev Sustain [Internet] 23(2):2204–2223. https://doi.org/10.1007/s10668-020-00670-9
Almutairi NS (2016) Causes of delays on construction projects in Kuwait according to opinion of engineers working in Kuwait. IJERA Journal 6:84–96
Alolayan MA, Brown KW, Evans JS, Bouhamra WS, Koutrakis P (2013) Source apportionment of fine particles in Kuwait City. Sci Total Environ 448:14–25. https://doi.org/10.1016/J.SCITOTENV.2012.11.090
Alosairi Y, Pokavanich T (2017) Seasonal circulation assessments of the Northern Arabian/Persian Gulf. https://doi.org/10.1016/j.marpolbul.2016.12.065 . Mar Pollut Bull
Alotaibi S (2011) Energy consumption in Kuwait: prospects and future approaches. Energy Policy [Internet] 39(2):637–643. https://doi.org/10.1016/j.enpol.2010.10.036
AlSabah R, Refaat O (2019) Assessment of construction risks in public projects located in the state of Kuwait. J Eng Res [Internet] [accessed 2022 Nov 3] 7(3). https://kuwaitjournals.org/jer/index.php/JER/article/view/4757
Alsahli MMM (2009) Characterizing surface temperature and clarity of Kuwait’s seawaters using remotely sensed measurements and GIS analyses [Internet]. [place unknown]: the University of Kansas. http://hdl.handle.net/1808/5969
Alsahli MM, Al-Harbi M (2018) Allocating optimum sites for air quality monitoring stations using GIS suitability analysis. Urban Clim. https://doi.org/10.1016/j.uclim.2017.11.001
Alsahli MMM, AlHasem AM (2016) Vulnerability of Kuwait coast to sea level rise. Geogr Tidsskr J Geogr [Internet] 116(1):56–70. https://doi.org/10.1080/00167223.2015.1121403
Bauman AG, Feary DA, Heron SF, Pratchett MS, Burt JA (2013) Multiple environmental factors influence the spatial distribution and structure of reef communities in the northeastern Arabian Peninsula. Mar Pollut Bull 72(2):302–312. https://doi.org/10.1016/j.marpolbul.2012.10.013
Bercht AL (2021) How qualitative approaches matter in climate and ocean change research: uncovering contradictions about climate concern. Glob Environ Chang 70:102326. https://doi.org/10.1016/J.GLOENVCHA.2021.102326
Bhandary H, Sabarathinam C, Al-Khalid A (2018) Occurrence of hypersaline groundwater along the coastal aquifers of Kuwait. Desalination 436(October 2017):15–27. https://doi.org/10.1016/j.desal.2018.02.004
Clarke L, Jiang K, Akimoto K, Babiker M, Blandford G, Fisher Vanden K, Hourcade J-C, Krey V, Kriegler E, Löschel A et al (2014) Chapter 6 - assessing transformation pathways. In: Chen W, Weyant J (eds) Clim Chang 2014 Mitig Clim Chang Contrib Work Gr III to Fifth assess Rep Inter- Gov Panel Clim Chang [Internet]. Cambridge University Press;, Cambridge, United Kingdom, pp 413–510. [accessed 2022 Nov 21] https://www.ipcc.ch/site/assets/uploads/2018/02/ipcc_wg3_ar5_chapter6.pdf
De Bruin K, Dellink RB, Ruijs A, Bolwidt L, Van Buuren A, Graveland J, De Groot RS, Kuikman PJ, Reinhard S, Roetter RP et al (2009) Adapting to climate change in the Netherlands: an inventory of climate adaptation options and ranking of alternatives. Clim Change. https://doi.org/10.1007/s10584-009-9576-4
Dudley AL, Meza I, Naumann G, Hagenlocher M (2022) Do global risk assessments leave countries behind? How the selection of countries influences outcomes of drought risk assessments. Clim Risk Manag [Internet] [accessed 2022 Sep 7] 37:100454. https://doi.org/10.1016/J.CRM.2022.100454
Fakhruddin BSHM, Boylan K, Wild A, Robertson R (2019) Assessing vulnerability and risk of climate change. In: SILLMANN J, SIPPEL S, RUSSO S (eds) Clim Extrem their implic impact risk assess. Elsevier, Amsterdam, Netherlands, pp 217–241. https://doi.org/10.1016/B978-0-12-814895-2.00012-4
Füssel HM, Klein RJT (2006) Climate change vulnerability assessments: an evolution of conceptual thinking. Clim Change 75(3):301–329. https://doi.org/10.1007/s10584-006-0329-3
Geravandi S, Sicard P, Khaniabadi YO, De Marco A, Ghomeishi A, Goudarzi G, Mahboubi M, Yari AR, Dobaradaran S, Hassani G et al (2017) A comparative study of hospital admissions for respiratory diseases during normal and dusty days in Iran. Environ Sci Pollut Res. https://doi.org/10.1007/s11356-017-9270-4
Ghazanfar SA, Böer B, Al Khulaidi AW, El-Keblawy A, Alateeqi S (2019) Plants of Sabkha ecosystems of the Arabian Peninsula. In: Gul B, Böer B, Khan MA, Clüsener-Godt M, Hameed A (eds) Sabkha Ecosyst vol VI Asia/Pacific [Internet]. Springer International Publishing, Cham, pp 55–80. https://doi.org/10.1007/978-3-030-04417-6_5
Gorai AK, Tuluri F, Tchounwou PB (2014) A GIS based approach for assessing the association between air pollution and asthma in New York State, USA. Int J Environ Res Public Health. https://doi.org/10.3390/ijerph110504845
Gueymard CA, Al-Rasheedi M, Ismail A, Hussain T (2017) Long-term variability of aerosol optical depth, dust episodes, and direct normal irradiance over Kuwait for CSP applications. ISES Sol World Congr 2017 - IEA SHC Int Conf Sol Heat Cool Build Ind 2017, Proc.(December 2018):75–84. https://doi.org/10.18086/swc.2017.04.04
Gulseven O (2016) Forecasting population and demographic composition of Kuwait until 2030. Int J Econ Finan Issues 6(4):1429–1435
Hawchar L, Naughton O, Nolan P, Stewart MG, Ryan PC (2020) A GIS-based framework for high-level climate change risk assessment of critical infrastructure. Clim Risk Manag 29. https://doi.org/10.1016/j.crm.2020.100235
Hoegh-Guldberg O, Mumby PJ, Hooten AJ, Steneck RS, Greenfield P, Gomez E, Harvell CD, Sale PF, Edwards AJ, Caldeira K et al (2007) Coral reefs under rapid climate change and ocean acidification. Science. https://doi.org/10.1126/science.1152509
Huang J, Ji M, Xie Y, Wang S, He Y, Ran J (2016) Global semi-arid climate change over last 60 years. Clim Dyn [Internet] [accessed 2022 Nov 3] 46(3–4):1131–1150. https://doi.org/10.1007/s00382-015-2636-8
Ibrahim HD, Eltahir EAB (2019) Impact of brine discharge from seawater desalination plants on Persian/Arabian Gulf Salinity. J Environ Eng 145(12):1–12. https://doi.org/10.1061/(asce)ee.1943-7870.0001604
KEPA (2017) eMISK - environmental atlas of the state of Kuwait [Internet]. [accessed 2022 Nov 2]. https://enterprise.emisk.org/env-atlas/en/
KEPA (2019a) State of Kuwait Second National Communication [Internet]. Kuwait City, Kuwait. https://epa.org.kw/Portals/0/PDF/StateOfKuwaitSecondEN.pdf
KEPA (2019b) Climate change mitigation and adaptation workshop held on the third floor of the Kuwait Environmental Public Authority (KEPA) building
Khadadah M (2013) The cost of asthma in Kuwait. Med Princ Pract 22(1):87–91. https://doi.org/10.1159/000341154
Khalaf FI, Al-Saleh S, Al-Houty F, Ansari L, Shublaq W (1979) Mineralogy and grain size distribution of dust fallout in Kuwait. Atmos Environ 13(12):1719–1723. https://doi.org/10.1016/0004-6981(79)90334-2
Kim Y, Calzada A, Scott O, Zermoglio F (2018) Designing climate vulnerability assessments. United States Agency for International Development, Washington, DC. https://www.climatelinks.org/projects/atlas
Kuwait Meteorological Department (2019) Climatological data of international Kuwait airport station from 2007 to 2016. Kuwait Meteorological Department of the Directorate General of Civil Aviation, Kuwait. https://www.met.gov.kw/
Lam VWY, Cheung WWL, Reygondeau G, Rashid Sumaila U (2016) Projected change in global fisheries revenues under climate change. Sci Reports 6(1):1–8. https://doi.org/10.1038/srep32607
Lasage R, Verburg PH (2015) Evaluation of small scale water harvesting techniques for semi-arid environments. J Arid Environ 118:48–57. https://doi.org/10.1016/J.JARIDENV.2015.02.019
Latcharote P, Al-Salem K, Suppasri A, Pokavanich T, Toda S, Jayaramu Y, Al-Enezi A, Al-Ragum A, Imamura F (2018) Tsunami hazard evaluation for Kuwait and Arabian Gulf due to Makran Subduction Zone and Subaerial landslides. Nat Hazards 93:127–152. https://doi.org/10.1007/s11069-017-3097-7
Maliva R (2021) Sea level rise and groundwater. Springer International Publishing, Cham, pp 113–153. https://doi.org/10.1007/978-3-030-66813-6_6 . In: Clim Chang Groundw Plan Adapt a Chang Uncertain Futur WSP Methods Water Resour Eval Ser No 6 [Internet]
McNeeley SM, Even TL, Gioia JBM, Knapp CN, Beeton TA (2017) Expanding vulnerability assessment for public lands: the social complement to ecological approaches. Clim Risk Manag 16:106–119. https://doi.org/10.1016/j.crm.2017.01.005
Mengist W, Soromessa T, Legese G (2020) Method for conducting systematic literature review and meta-analysis for environmental science research. MethodsX 7:100777. https://doi.org/10.1016/J.MEX.2019.100777
MEW (2022) Statistical year book: water. Kuwait
Mohammad ER, Al-Dashti H, Aldosari A, AlNassar M (2018) Vulnerability and adaptation: climatic conditions. In: Al-Harbi M (ed) Vulnerability adapt to Clim Chang team Rep. Kuwait Environment Public Authority, Kuwait, pp 1–10
Omar F (2022) Kuwait’s outdoor labor ban: 730 violations in June. Kuwait Times [Internet]. [accessed 2022 Nov 3]. https://www.kuwaittimes.com/kuwaits-outdoor-labor-ban-730-violations-in-june/
Omar Asem S, Roy WY (2010) Biodiversity and climate change in Kuwait. Int J Clim Chang Strateg Manag 2(1):68–83. https://doi.org/10.1108/17568691011020265
PACI (2019) Kuwait demographic data of 2019 - The Public Authority for Civil Information [Internet]. Kuwait. https://www.paci.gov.kw/stat/
Pal JS, Eltahir EAB (2015) Future temperature in southwest Asia projected to exceed a threshold for human adaptability. Nat Clim Chang 6(2):197–200. https://doi.org/10.1038/nclimate2833
Sabarathinam C, Bhandary H, Al-Khalid A (2019) A geochemical analogy between the metal sources in Kuwait Bay and territorial sea water of Kuwait. Environ Monit Assess [Internet] [accessed 2022 Dec 11] 191(3):1–19. https://doi.org/10.1007/s10661-019-7219-4
Sabbah I, Al-Mudhaf HF, Al-Kandari A, Al-Sharifi F (2012) Remote sensing of desert dust over Kuwait: long-term variation. Atmos Pollut Res [Internet] 3(1):95–104. https://doi.org/10.5094/APR.2012.009
Sebastian M, Kaaya LT (2018) Impacts of Sea Surface temperature on Coral Reefs in Mafia Island, Tanzania. J Mar Sci Res Dev 8(3):5
Segar DA (2018) Chapter 12: Foundation of life in the oceans. Introd to Ocean Sci. [place unknown]
Sousa DS, Neves CF, Silva HVO, Schaffel SB, Luigi G, La Rovere EL (2022) A systemic approach for climate risk assessment applied to thermoelectric power plants in northeastern coast of Brazil. Clim Risk Manag [Internet] 36:100424. https://doi.org/10.1016/j.crm.2022.100424 . May 2021
Stocker TF, Qin D, Plattner GK, Tignor MMB, Allen SK, Boschung J, Nauels A, Xia Y, Bex V, Midgley PM (2013) Climate change 2013 the physical science basis: Working Group I contribution to the fifth assessment report of the intergovernmental panel on climate change. Clim Chang 2013 Phys Sci Basis Work Gr I Contrib to Fifth Assess Rep Intergov Panel Clim Chang 9781107057999:1–1535. https://doi.org/10.1017/CBO9781107415324
Uddin S, Al Ghadban AN, Khabbaz A (2011) Localized hyper saline waters in Arabian Gulf from desalination activity-an example from South Kuwait. Environ Monit Assess 181(1–4):587–594. https://doi.org/10.1007/s10661-010-1853-1
Water F (2022) Water resources in Kuwait - Fanack Water [Internet]. [accessed 2022 Dec 10]. https://water.fanack.com/kuwait/water-resources-in-kuwait/#_ftn11
WBG (2021) World Bank Group. Washington, DC 20433. https://doi.org/10.1596/978-1-4648-0484-7_world_bank_group
WHO (2022) Asthma [Internet]. [accessed 2023 Jan 6]. https://www.who.int/news-room/fact-sheets/detail/asthma
Younis M, Al-Hajeri M, Celik Y, Kisa A, Richard P, Parkash J (2015) Healthcare of aging population of Kuwait. Ageing Int 40(1):36–43. https://doi.org/10.1007/s12126-012-9151-6
Download references
Acknowledgements
The authors would like to thank Ms. Anfal Alenezi for her valuable support in the interviews, the Kuwait Environmental Public Authority (KEPA) for hosting the workshop and participating in the interviews (especially the Coastal and Desertification Monitoring Department employees), the Public Authority for Civil Information (PACI) for providing the demographic data, and the United Nations Development Programme (UNDP) for their support. The authors would also like to express their gratitude to Eng. Fatin Al-Tamimi from the Ministry of Electricity, Water and Renewable Energy for the invaluable information about the desalination plant operations and issues.
Author information
Authors and affiliations.
Department of Geography, College of Social Sciences, Kuwait University, P.O. Box 5969, Kuwait, Safat, 13060, Kuwait
Mohammad M. M. Alsahli
Department of Earth and Environmental Sciences, College of Science, Kuwait University, P.O. Box 5969, Kuwait, Safat, 13060, Kuwait
Dhary S. Alkandary
You can also search for this author in PubMed Google Scholar
Corresponding author
Correspondence to Mohammad M. M. Alsahli .
Ethics declarations
Conflict of interest.
The authors declare no competing interests.
Additional information
Communicated by Zhihua Zhang.
Publisher’s Note
Springer Nature remains neutral with regard to jurisdictional claims in published maps and institutional affiliations.
Electronic supplementary material
Below is the link to the electronic supplementary material.
Supplementary Material 1
Rights and permissions.
Springer Nature or its licensor (e.g. a society or other partner) holds exclusive rights to this article under a publishing agreement with the author(s) or other rightsholder(s); author self-archiving of the accepted manuscript version of this article is solely governed by the terms of such publishing agreement and applicable law.
Reprints and permissions
About this article
Alsahli, M.M.M., Alkandary, D.S. Climate change vulnerability of Kuwait: a cross-sectoral assessment. Arab J Geosci 17 , 183 (2024). https://doi.org/10.1007/s12517-024-11992-7
Download citation
Received : 04 January 2024
Accepted : 10 May 2024
Published : 20 May 2024
DOI : https://doi.org/10.1007/s12517-024-11992-7
Share this article
Anyone you share the following link with will be able to read this content:
Sorry, a shareable link is not currently available for this article.
Provided by the Springer Nature SharedIt content-sharing initiative
- Arid environment
- Desertification
- Urban susceptibility
- Environmental resilience
- Spatial investigation
- Find a journal
- Publish with us
- Track your research

IMAGES
VIDEO
COMMENTS
Many studies of water desalination costs appear regularly in water desalination and renewable energy related publications. Cost estimates seem to be very much site specific and the cost per cubic metre ranges from installation to installation. ... Water desalination cost literature: review and assessment Desalination 223 (2008) 448-456 0011 ...
For brackish water desalination, the water production cost of large-capacity BWRO plants (40,000 to 46,000 m 3 / day) varies from 0.26 to 0.54 $/m 3 , while the production cost of ED plants ranges ...
Desalination. Volume 223, Issues 1-3, 1 March 2008, Pages 448-456. Water desalination cost literature: review and assessment. Presented at the conference on Desalination and the Environment. Sponsored by the European Desalination Society and Center for Research and Technology Hellas (CERTH), Sani Resort, Halkidiki, Greece, April 22-25, 2007.
Desalination 223 (2008) 448-456 Water desalination cost literature: review and assessment Ioannis C. Karagiannis*, Petros G. Soldatos Agricultural University of Athens, Department of Agricultural Economics & Rural Development, 75 Iera Odos Street, GR 11855 Athens, Greece Tel. +30 210 5294769; Fax +30 210 5294776; email: [email protected] Received 21 December 2006; accepted 28 February ...
The Economics of Desalination for Various Uses. Carlos Campos. Environmental Science, Economics. 2009. Water scarcity is a major problem that needs to be efficiently solved to ensure water availability for future generations. Desalination is an alternative technology for water production based on salt…. Expand. 33. Highly Influenced.
For conventional systems the cost for seawater ranges from .4h/m3 to more than 3h/m3, while for brackish water desalination the cost is almost half. When renewable energy sources are used the cost is much higher, and in some cases can reach even 15h/m3, due to most expensive energy supply systems. However, this cost is counterbalanced by the ...
As water resources are rapidly being exhausted, more and more interest is paid to the desalination of seawater and brackish water concentrations. Today, current desalination methods require large amounts of energy which is costly both in environmental pollution and in money terms. <P />Many studies of water desalination costs appear regularly in water desalination and renewable energy related ...
Water desalination cost literature: review and assessment. Desalination (2008) A. Al-Karaghouli et al. ... allowing for accurate cost assessments. The results show that levelized cost of water between 2.51 and 3.69 €/m 3 can be reached depending on the configuration of the system. Moreover, the cash flow analysis demonstrates that the ...
Other approaches in the literature to model desalination costs specifically are based on the development of empirical cost models calibrated with historical data on capital costs (Loutatidou et al., 2014) or "total water costs" (annualized capital cost + annual operation and maintenance cost) (Dore, 2005; Gao et al., 2017; Wittholz et al ...
A literature study for costs and SEC for brackish water desalination demonstrate the suitability of the preselected desalination technologies according to the before described requirements ...
Studies have shown variations in prices of desalinated water produced with conventional and renewable energy. Using conventional energy for desalination is most cost-efficient: $0.2-1.3/m 3 ($0.76-4.9/kgal) for desalinated brackish groundwater and $0.2-3.2/m 3 ($0.76-12.1/kgal) for desalinated seawater.
In conclusion, an extensive review and assessment of literature reveal that despite lots of improvement efforts, the leveling-off water production cost for existing desalination systems is hovering around 0.9 ± 0.3 $/m 3 (refer Fig. 14).
Eventually, this article discusses to what extent desalination constitutes a radical break with the old 20th century hydraulic paradigm and interrogates whether it risks repeating the same mistakes, thus becoming a maladaptive strategy to cope with 21st century global water issues. WIREs Water 2015, 2:231-243. doi: 10.1002/wat2.1073
As water resources are rapidly being exhausted, more and more interest is paid to the desalination of seawater and brackish water concentrations. Today, current desalination methods require large amounts of energy which is costly both in environmental pollution and in money terms.Many studies of water desalination costs appear regularly in water desalination and renewable energy related ...
As water resources are rapidly being exhausted, more and more interest is paid to the desalination of seawater and brackish water concentrations. Today, current desalination methods require large amounts of energy which is costly both in environmental pollution and in money terms. Many studies of water desalination costs appear regularly in water desalination and renewable energy related ...
Hence, the authors in the present paper attempt to the evaluation of the true cost of desalinated water. In this context, to date, a fundamental review paper concerning an exhaustive assessment of desalination cost is felt missing, because the related data is highly scattered and out of date in the literature.
1. Introduction. To overcome the ever increasing freshwater demand due to population growth and welfare concerns, the first desalination plants were installed in the late 1950s [ 1, 2, 3 ]. The first technologies were thermally driven due to the low cost of fossil fuels (less than $ 3 for one barrel of oil).
In 2013, there were over 17,000 active desalination plants, providing about 80 × 10 6 m 3 /day water to 300 million people in 150 countries [ 9 ]. By 2015, the production capacity increased to nearly 97.5 × 10 6 m 3 /day [ 10 ]. The supply of desalinated water is expected to increase to 192 × 10 6 m 3 /day by 2050 [ 11 ].
The major present hindrance in using desalination to help alleviate global water scarcity is the cost of this technology, which, in turn is due to energy cost involved. This study examines historical trends in desalination and breaks up the cost of desalination into energy based and nonenergy based.
AbstractOne of the major difficulties in the assessment of costs of desalination projects is that key investment parameters and operation-related parameters are project-specific data. ... Water desalination cost literature: review and assessment. Ioannis Karagiannis P. Soldatos. Environmental Science. 2008; 704. Save. Evaluating the costs of ...
This chapter summarizes the techno-economical aspect of conventional desalination technologies. As the total energy required and the efficiency of the process are the main parameters to decide the system for commercialization hence these calcualtions are performed and determined by a detailed energy analysis for conventional methods and hydrate based desalaintion.
Desalination tools. Desalination in Egypt. 1. Introduction. However, water covers approximately three-quarters of the surface of the earth but over 97.2 % of the total water resources are saline or brackish water bodies. More than 2.1 % of the remaining 2.8 % of the available freshwater is tied in ice caps, glaciers, atmosphere and soil moisture.
A comprehensive review of solar-driven desalination technologies for off-grid greenhouses ... be considered when selecting solar-driven desalinations for greenhouses and presents a detailed comparison between the water production rate and cost as well as the energy consumption of these systems. ... Citing Literature. Volume 43, Issue 4. 25 ...
Data collection and analysis approach. The climate change vulnerability of four sectors (i.e., marine and fisheries, water resources, land management, and health) at a country level was assessed using qualitative and semi-quantitative approaches adopted in many climate change studies (McNeeley et al. 2017; Sousa et al. 2022).Experts and stakeholders identified these sectors in the SNC as the ...
None of the desalination cost assessment papers reviewed did explicitly account for the costs of the initial design and the costs of the process required to go through for securing all necessary permits. ... Water desalination cost literature: review and assessment. Desalination, 223 (2008), pp. 448-456, 10.1016/j.desal.2007.02.071. View PDF ...