Advertisement

Industry 4.0, a revolution that requires technology and national strategies
- Survey and State of the Art
- Open access
- Published: 27 January 2021
- Volume 7 , pages 1311–1325, ( 2021 )
Cite this article
You have full access to this open access article
- Fengwei Yang 1 &
- Sai Gu ORCID: orcid.org/0000-0002-0619-0840 2
55k Accesses
139 Citations
2 Altmetric
Explore all metrics
Since 2011, when the concepts of Industry 4.0 were first announced, this industrial revolution has grown and expanded from some theoretical concepts to real-world applications. Its practicalities can be found in many fields and affect nearly all of us in so many ways. While we are adapting to new changes, adjustments are starting to reveal on national and international levels. It is becoming clear that it is not just new innovations at play, technical advancements, governmental policies and markets have never been so intertwined. Here, we generally describe the concepts of Industry 4.0, explain some new terminologies and challenges for clarity and completeness. The key of this paper is that we summarise over 14 countries’ up-to-date national strategies and plans for Industry 4.0. Some of them are bottom-up, such as Portugal, some top-down, such as Italy, a few like the United States had already been moving in this direction long before 2011. We see governments are tailoring their efforts accordingly, and industries are adapting as well as driving those changes.
Similar content being viewed by others
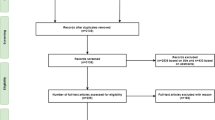
Artificial Intelligence and Business Value: a Literature Review
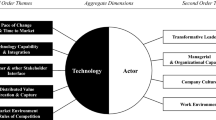
Digital transformation: a review, synthesis and opportunities for future research
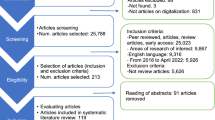
The role of digitalization in business and management: a systematic literature review
Avoid common mistakes on your manuscript.
Introduction
Looking back to the previous industrial revolutions: the first started at the end of the eighteenth century with the increasing use of steam and water power and resulted in a transition from hand production methods to machines (i.e. mechanisation); the second began in the late nineteenth century, utilised on electrical energy and enabled mass production (i.e. intensive use of electrical power); the third made use of electronics and internet technology from the 1970s and automated productions (i.e. digitalisation) [ 1 , 2 , 3 , 4 , 5 , 6 , 7 , 8 , 9 ]. We may see from previous industrial revolutions that technological advances met broader industrial demands amongst their trigger conditions.
Right now, we are on the brink of a new industrial revolution, namely the Fourth Industrial Resolution or Industry 4.0 [ 1 , 2 , 3 , 5 , 10 , 11 , 12 , 13 , 14 , 15 , 16 , 17 , 18 , 19 , 20 ]. Why now? A convincing reason pointed out by many, such as Lasi et al. [ 1 ], is that we have reached a point that there exists a clear application-pull (industrial demands) and technology-push (technological advances), acting together as a driving force for this new revolution.
On the one hand, amongst general social, economic and political fields, there is a remarkable need for change and Lasi et al. [ 1 ] listed five particular points.
Shortening the development period with the use of highly innovative means.
Using ultra-customisation to end the traditional “one for all” and to promote uniqueness or is sometimes called “batch size one” in manufacturing.
Productions are integrated with higher flexibility.
Enabling faster decision-making procedures by decentralisation as opposed to lengthy organisational hierarchy.
Promoting sustainability and resource efficiency in the context of the ecological aspect.
On the other hand, the recent decade saw numerous technologies booming from novel research and development of all sides. To name a few: radio frequency identification [ 21 ], various wireless network protocols [ 2 , 22 , 23 ], enterprise resource planning [ 3 , 24 , 25 , 26 , 27 ], knowledge management [ 28 , 29 , 30 , 31 , 32 ], Internet of Things [ 6 , 33 ], cloud-based computation [ 17 , 34 , 35 ] and manufacturing [ 2 , 7 , 36 , 37 , 38 , 39 , 40 ]. Typically, we summarise this technology-push as the following [ 1 ],
more extensive use of mechanisation and automation;
digitalisation and networks linking all components within the industry;
revolutionary miniaturisation which has made a significant impact on electronics, transportation and logistics.
The idea of Industry 4.0 arose from the Hannover Fair in 2011, and the German government officially announced it in 2013 as a German strategic initiative, to play a pioneering role in its manufacturing sector [ 4 , 5 , 6 , 7 , 8 , 41 , 42 , 43 , 44 , 45 , 46 , 47 , 48 , 49 , 50 , 51 , 52 , 53 , 54 , 55 , 56 , 57 , 58 , 59 , 60 ]. As Zhong et al. [ 7 ] have summarised, in the context of Industry 4.0, intelligent manufacturing systems feed on advanced information from every corner of the relevant fields, so that they become flexible, smart and customisable to address a dynamically ever-changing global market. The key is to have all information flow through the whole holistic manufacturing supply chain and all relevant industries. Some argue this concept had already been raised, as early as 1988 by Rostow [ 2 , 61 ].
According to [ 62 , 63 ], the goals of Industry 4.0 are to provide IT-enabled mass customisation of manufactured products; to make automatic and flexible adaptation of the production chain; to track parts and products; to facilitate communication among parts, products, and machines; to apply human–machine interaction (HMI) paradigms; to achieve IoT-enabled production optimization in smart factories; and to provide new types of services and business models of interaction in the value chain. [ 8 ] concludes “the principles of Industry 4.0 are interoperability, virtualization, decentralization, real-time capability, service orientation, and modularity”. Similarly, [ 5 , 20 ] point out, the five major features of Industry 4.0 are digitization, optimization, and customisation of production; automation and adaptation; human–machine interaction (HMI); value-added services and businesses, and automatic data exchange and communication. [ 62 , 63 ] discuss flexibility, reduce lead times, customize with small batch sizes, and reduce costs. [ 41 , 64 ] expand the talk points to include cloud/intranet, data integration, flexible adaptation, intelligent, self-organizing, interoperability, manufacturing process, optimization, secure communication, and service orientation. [ 1 , 5 , 65 , 66 , 67 ] believe that the key point through various connections (e.g. cyber-physical systems, the Internet of Things and the Internet of services) is the communication of information, thus to do with knowledge management. Communication from various perspectives is discussed in [ 68 , 69 , 70 , 71 , 72 ].
Industry 4.0 is still in its infancy. It is in a conceptual state which intends to integrate very large number of dynamic technological concepts. In this review paper, we aim to explain these concepts of Industry 4.0 and their implications. More importantly, how it has evolved and concerning the large number of new jargon that have developed since 2011, we would like to provide a jargon buster to our reader. In the next section, we summarise several fundamental concepts of Industry 4.0. Due to the fact that there is no single-clear standard for Industry 4.0, each country is implementing its own version of it. Differences in their approaches are typically caused by each market and industry specialisation. This will eventually change the outcomes of Industry 4.0 as what we have predicted now. In Germany, there is a focus on trying to develop fully automated, Internet-based “smart” factories. [ 73 ] mentioned an early-stage example that is called the Amberg plant, where most units in this 100,000-plus square-foot factory can fetch and assemble components without further human input. The European Union encourage research into the field of smart technologies as a whole. Its research program “Horizon 2020” offers funding for research and development projects such as smart cities and communities’ information, strategic roles of smart cities for tackling energy and mobility challenges, analysing the potential for wide-scale rollout of integrated smart cities and communities’ solutions, and so on [ 5 , 74 ]. Other countries are targeting their advantages with different policies. In the following section, we list several countries which have recently announced their particular Industry 4.0 plans and explore the different implications at levels of politics and policy-making. A conclusion with possible topics of future discussions is presented in the final section.
Industry 4.0 concepts
There were nine pillars of Industry 4.0 when it was first announced: cyber-physical systems, Internet of Things, Big data, 3D printing, robotics, simulation, augmented reality, cloud computing and cyber security. As the world keeps exploring the concepts of Industry 4.0 and their practicalities, we see from many literature, [ 4 , 5 , 6 , 7 , 8 , 41 , 75 ] to name a few, that some of the concepts have been shifted around. Whether it is a better-fitting name or some further clarifications, we see an increasing individualization in our understanding of the Industry 4.0 itself. In Table 1 , we would like to provide a jargon buster and further in this section, we summarise these concepts of Industry 4.0 and provide some easy descriptions to the reader. Technical details may be found from the references.
Cyber-physical systems
Cyber-physical systems are the core of Industry 4.0 [ 34 , 53 , 76 , 77 , 78 ]. On a simple term, it could just mean linking a typical equipment to a computer, such as a valve to a electrical switch, but the full picture is far beyond hardware adjustments. When to switch the valve and what the effect it would have towards the whole operation are just some straightforward questions. Furthermore, what is behind the decision of this switch, how confident this decision is made, how good is our prediction of what is going to happen afterwards, and finally what is the error margin in all of these are the mission-critical issues that we must understand.
From 2011, when the idea of Industry 4.0 was announced, to the present, the concept of cyber-physical systems has been subdivided via various perspectives, and interpreted with many new names. When [ 75 ] talks about “learning” and “making high-level cognitive decisions”, it is mainly on the algorithmic level, which is often handled by the software. This is artificial intelligence. Allowing machines to solve problems by self-expanding its own behaviours is driving us towards the world of autonomous, self-regulating systems. The direct benefits of applying appropriate artificial intelligence could mean less downtime in smart factories, optimised production, better energy management. Outside of production, it could mean seamless information flow in logistics, where suppliers, self-organised work orders and schedules and better order fulfilment. According to [ 75 ], the current range of artificial intelligence is low. The figures range from \(4\%\) for small firms with less than 10 employees, to \(9\%\) for firms with between 10 and 250 employees, but this could change very quickly and significantly.
An another view of cyber-physical systems, such as [ 7 , 79 , 80 ], describes it as a mechanism of which physical objects and software are closely intertwined, allowing components to communicate in a myriad of ways. Sometimes this view is termed as “horizontal and vertical integration”. [ 4 , 37 ] state there are three essential integrations in the paradigm of Industry 4.0: (a) horizontal integration across the entire value creation network, (b) vertical integration and networked manufacturing systems (c) end-to-end engineering across the entire product life cycle. A fully digitalised and integrated process in the horizontal and vertical dimension would yield an automation of manufacturing process [ 81 ]. An example is the so-called embedded system. By closely linking the physical devices to their computational elements, it can enable highly coordinated functions [ 82 ]. The latest development employs interactive connections to form a large network. Typically, a large number of devices are involved. Many are sensors such as touch screens, light sensing and force sensing devices.
To sum up, traditional cyber-physical systems have been used in a large range of industries, such as manufacturing, aerospace, automotive, chemical, energy, healthcare and transportation [ 83 , 84 , 85 ]. From 2006, the National Science Foundation of the United States and other United States agencies have sponsored research projects on cyber-physical systems [ 6 ]. Since the launch of the concept of Industry 4.0, many countries and industries have started investing and developing in the cyber-physical domains. Despite differences in definitions of those cyber-physical systems, cyber (software) and physical (hardware) are increasingly interconnected [ 86 ]. A higher level of integration of coordination between all components is on the horizon. The current developing trends aim to achieve reliable, secure and certifiable system and control methodologies [ 87 ]. It is widely shared that integrating systems and subsystems from different hierarchies is time-consuming and costly, sometime the whole system has to be kept operational and yields further complications [ 6 , 7 ]. Multidisciplinary collaboration is believed to be the key [ 7 ]. A large number of interdisciplinary methodologies have been used to develop these systems, such as cybernetics theory, mechanical engineering and mechatronics, design and process science, manufacturing systems, and computer science.
Internet of Things
In simple terms, the Internet of Things consists of physical devices that are embedded with electronic sensors, actuators, and digital devices with specific software enabling communications [ 7 , 75 ]. All of them are connected to an inter-networking world, typically through the Internet [ 7 ]. Typical example of these devices may include almost all suitable home appliance such as kettles and light switches, as well as industrial machines like pumps and motors. Some literature refers to the industrial section as Industrial Internet of Things [ 4 , 6 , 7 , 75 ]. Additionally, it is also known as Internet of Everything which consists of Internet of Service, Internet of Manufacturing Services, Internet of People, an embedded system and Integration of Information and Communication technology [ 4 , 88 ]. Although the connectivity is not unique to the Industry 4.0 revolution, the integration via Internet of Things allows data to be collected and exchanged on a unprecedented scale. According to the research firm Gartner, there were more than eleven billion connected things estimated worldwide in 2018 [ 75 ]. This number becomes nearly 20.8 billion by 2020 [ 89 ]. Industry Internet plays a big part of this industrial revolution [ 90 , 91 ].
On a detailed perspective, to handle a gigantic number of devices and dynamically manage these connections, there are innovative key techniques required. In fact, when the Internet of Things first emerged, it was referred to uniquely identifiable interoperable connected objects using radio-frequency identification technology [ 6 , 92 , 93 ]. One may trace back to 1982, when researchers connected a modified Coke Machine to the Internet at Carnegie Mellon University [ 7 , 94 ]. This identification technique servers as a tag that is uniquely identified and allows tracking in real-time. Later on, this technique is expanded to cooperating via wireless network, Bluetooth, cellular networks or near field communication [ 6 ]. van Kranenburg [ 95 ] defines the Internet of Things as “a dynamic global network infrastructure with self-configuring capabilities based on standard and interoperable communication protocols where physical and virtual ‘Things’ have identities, physical attributes, and virtual personalities and use intelligent interfaces, and are seamlessly integrated into the information network.”
A large number of Internet of Things application have already been applied, for example, monitoring in fields of industries, environments, transportation, and healthcare [ 96 , 97 , 98 , 99 , 100 ]. However, we note that to connect billions of devices, some standard protocols must be followed by all players in the new ear of Industry 4.0. This can cause new issues such as privacy, security as well as proprietary ownership. Thus, innovative interdisciplinary research is ever paramount to address news problems from new solutions.
Big data and data analytics
Imaging the amount of information we have created from the dawn of civilisation to 2003, we now create as much every 2 days, this is according to a former executive chairman of Google Eric Schmidt [ 75 ]. That is approximately five exabytes a day (around 5 million TBs). Initially, storage and handling of the data were at the centre of research and development. Then we worried about the quality of the data. Now it is clear that data has become a commodity [ 7 , 75 ].
In general, Industry 4.0 generates data from a large various channels, such as sensor readings, log files, video/audio, network traffics, transactions, social media feeds [ 4 ]. Industries such as big technology companies have already tasted success at analysing big data and mining valuable information from it [ 75 ]. Those information could give companies insights into the business market and get ahead of its competition. For example, with shop-floor data from all across a large region, advanced algorithm can find patterns and correlations identify future market trends and customer preferences. According to [ 101 ], research has shown an increase of 15–20% in return on investment by introducing big data analysis. Big data visualisation is another research trend to tackle the difficulties of making senses from a gigantic dataset [ 102 ].
Cloud and information technology
Industry 4.0 is based upon the changes from advanced information technologies and cloud has become a major part of the this paradigm. It is clear cloud has become a mainstream in communication and hub of information exchange [ 75 ]. Cloud, or cloud computing, is a general term referring to computational services that offers scalable resources for various activities over the Internet [ 103 , 104 ]. Some literature such as [ 7 , 105 ] considers it as “the fifth utility”, alongside with electricity, gas, water and telephone. Cloud serves as remote servers that is generally available around the clock [ 6 ]. Its high-performance and low-cost feature suits perfectly for information storage, and over the recent years, rapid resource sharing, dynamic allocation, flexible extension have expanded its influence to our day to day life. [ 75 ] reports from a survey that 88% of companies participated stated cloud yields positive outcomes, and often allows access to new markets and new customers. According to a chief officer of Facebook, Sheryl Sandberg, more than 50 million business using Facebook Pages to communicate with customers [ 75 ]. One third of customers now have an expectation to receive an response from a business within half an hour and over 40% expect within an hour.
With the increasing use of cloud, the idea of cloud manufacturing has emerged that allows modularization and service-orientation [ 6 ]. For example, design from cloud manufacturing enables a large number of participants from all stages of the production process. Therefore, customer suggestions and engineer concerns can all be included into the design phase, largely reduce its turnaround time. Apart from the design, there are aspects of cloud manufacturing such as software as a service, customer relationship management, platforms for data analytics, collaboration and business planning [ 75 ].
Robots and automated machinery
Robots play an important role in Industry 4.0. Depending on different requirements, it can be robotics arms, a whole assembly line, vehicle-type rover, android or legged patrol robots. Many can already be seen in the fields of chemical processing, pharmaceutical manufacturing, food and beverage productions [ 75 ]. Here are some examples. KUKA iiwa is a lightweight robot for sensitive industrial tasks, developed by KUKA Robotics. Baxter from Rethink Robotics is an interactive production robot for packaging purpose. BioRob Arm can be used in close proximity with humans. Automated machinery is aimed to perform repeated actions with high speed and precision as well as be able to work where human workers are restricted [ 4 , 106 ]. [ 107 ] illustrates an interface that controls industrial robots with augmented reality.
3D printing
3D printing, officially known as additive manufacturing in the Industry 4.0 terminology, enables construction of various complex geometries of structures using metal or plastic. In the recent years, it has attracted increasing investment. By 2023, the 3D printing market is estimated to be worth $32.78 billion [ 75 ]. The growth is believed in customised products and 3D printing is the ideal way of reducing overall manufacturing costs, flexible and extremely fast at producing small quantity. It also helps to reduce component weight and minimise waste, thus is of particular benefit to the automotive and aerospace industries [ 75 ]. In other cases, 3D printing allows flexible production locations, reduced transportation and stock on hand [ 4 ].
3D printing shows the way forwards as decentralised manufacturing as it makes production faster and cheaper. With increasing digitisation and the demand to decrease product life cycles, more areas find its benefits. Associated technologies have also been focused, such as fused deposition method, selective laser melting and selective laser sintering [ 4 ]. As product individualization becomes the trends, we are expecting to see ever more customised 3D printing services in the coming years.
Simulation is the combination of isolation and recreation. It first identifies variables within a situation and makes hypothesises, then comparing the simulation results to observation. After repeated testing, when the results are satisfactory, we can then make predictions with a given set of variables and conditions.
Simulation requires extensive work and is very important in areas like plant operations. For example, while monitoring real-time data, any oscillations or intended changes can be put into a well-studied simulation to predicted the real-world outcomes. Simulation can be used to ensure the quality of the product, as well as minimising costs from market price changes [ 4 ]. When errors occur, simulations can be used to shorten the down times. If possible outcomes can be predicted, it can also help decision-making [ 108 ].
Portable devices
There is no denial on the rise of popularity in portable devices such as smart phones, laptops and other wearable electronic products in recent years. The change of software development to adapting relatively smaller screen size of a phone has led to a big app market and two massive players (i.e. Google Play Store and Apple Store) dominating the scene. Portable devices also promote remote working, bring-your-own-device schemes and a various series of cross-platform compatibilities [ 75 ]. There are typically more than one connection methods on large portion of the portable devices, such as wireless and cellular networks. This offers manufacturers as well as developers a large degree of freedom in terms of the practical goals they would like to achieve.
Apart from day-to-day mobile app usage nearly becomes a necessity, augmented reality systems have also been developed and made practical in many fields. Augmented reality is a way of communicating via immersing the real-world surroundings and other real-time information. A typical example would be augmented reality headset or goggles that would be able to highlight and graphically demonstrate a series of repair instructions while the user is looking at the actual system [ 4 ].
Issues and challenges in Industry 4.0
There are two facts about Industry 4.0 that we can say with a degree of certainty. A new around of digital revolution is inevitable and this revolution is still in progress [ 4 ]. While we enjoy a wealth of benefits from those new technologies, issues and challenges will arise.
In the short term, first, a lack of autonomy in many current systems will limit industries moving towards smart manufacturing [ 109 ]. Second, a lack of bandwidth in majority of the current network protocols could become the bottleneck that requires decades to improve [ 109 ]. Third, many industries are yet to ensure the quality and integrity of their data recorded. There is a lack of standard approach for the annotations of data entities [ 110 ]. Fourth, modelling and analysis on complex systems are yet to be satisfactory for practical purposes [ 109 ]. Fifth, there are many difficulties to adjust the current production routes to adapt a large dynamical reconfiguration for individualized and customised products [ 4 ]. Finally, it is still unclear how best different sectors (e.g. small- and medium-sized enterprises vs Fortune 500) would invest and what are the optimal support from the governments of each country [ 5 ].
In the long term, one critical issue is cyber security. We have seen cyber-attacks such as WannaCry, Petya and NotPetya in the last 5 years [ 75 ]. A chocolate manufacturer in Australia was hacked in June 2017, leaving their system data locked and demanding a crypto-currency payment as ransom [ 75 ]. Although there are standard methods to increase cyber security, such as end-to-end encryptions, intrusion detection and prevention systems and virtual private networks, the increasing digitalisation still have vulnerabilities [ 5 ]. There have been a large amount of research on improving cyber security, one of them is termed “Blockchain” technique [ 111 ]. Fernandez-Carames and Fraga-Lamas described this concept in [ 111 ] and provided a detailed guide how Blockchain is applied into different Industry 4.0 applications. [ 112 ] illustrates a middleware approach of the Blockchain technique to enable more secured smart manufacturing application. [ 113 ] summarised existing Blockchain techniques within Internet of Things and discussed industry-specific challenges.
Data privacy is another critical issue. Different from being attached, data privacy highlights the possibility that our data may be misused, or the intention of usage was not disclosed initially. There is an increasing debate on social media about who owns the data and what they are allowed to do with it. An issue would need international collaboration on regulation and law making. Further or new education on people is yet another challenge [ 5 ]. With expanding digitalisation, people would have to develop “digital thinking” despite their educational background [ 114 ]. Some worry Industry 4.0 would eventually lead to redundancy in human labour force [ 115 ]. It is yet unclear what adaptation measures would be required to avoid this technological unemployment [ 116 , 117 ].
International efforts
The continuous development and practical applications of Industry 4.0 rely on government policies and supports. At the same time, it is to the governments’ benefits for materialising the outcomes of various Industry 4.0 practices. What has become clear is that many governments quickly followed suit after Germany and announced their versions of Industry 4.0 [ 2 ]. We see a united response from relevant governments across the globe acknowledging Industry 4.0 and in many cases, a competition to achieving this goal. On an international stage, we see for example “the Digital Economy and Society Index” ( https://ec.europa.eu/digital-single-market/en/desi ) of EU Member States to track the evolution and summarises a number of indicators every year on each country’s performance. There are five categories: Connectivity, Human Capital, Use of Internet, Integration of Digital Technology and Digital Public Services. These indices are having a profound influence to motivate each country to develop and practice the latest technologies. As individual countries, we see a so-called “Trilateral Cooperation” between Italy, France and Germany for Industry 4.0. Led by a “steering committee”, the involved countries are to bring together the implementing bodies of national strategies, promote the digitisation of the manufacturing section and provide wider support in this area.
We introduce some of major countries and their Industry 4.0 strategies in Table 2 , and illustrate in this section of their purposes.
Australia has officially announced its Industry 4.0 intention in August 2017 [ 118 ]. Namely, the Testlabs are a strategic initiative from its Prime Minister’s Industry 4.0 Taskforce. The aim is to improve the competitiveness of its manufacturing industries and start a transformation amongst its workforce. It also seeks research organisations and encourages them to be in partnership with its industry. The key actions from this Testlabs initiative are, first, to develop a network involving Australian universities and companies (particularly those small- and medium-sized enterprises), allowing them access to relevant governmental information and regulations; second, to build infrastructures for Industry 4.0 related technologies, for example, computational power, connectivity and energy. The pilot program of this initiative has been established at five Australian universities and awarded businesses $5 million to transition to the smart factories of the future. In 2018, a further $2.4 billion has been invested in growing Australian research, science and technology capabilities. Apart from funding, policies such as tax incentive, kick-start programmes to Australian space industry and job opportunity expansion have also been planned.
Alongside the Testlabs initiative, there is a special collaboration with Germany. The main aims are to expand Testlabs strategies to German companies and universities. promoting collaborations between the two countries.
Belgium has been one of the high-performing countries, its federal system is highly decentralised in policymaking, and this allows it to avoid hierarchy. In Belgium, small- and medium-sized enterprises account for 98% of all manufacturing companies. Thus, decentralisation is ideal for integrating Industry 4.0 concepts, tailored to individual advantages [ 75 ]. From a period of 2014–2019, regional governments of Belgium have made and implemented different policies regarding their digital transformation. At the federal level, “Digital Belgium” is an initiative that was launched in April 2015, supported by many CEO-s from digital companies, entrepreneurs, investors and academics. Its outlined a five-pillar action plan, digitalising economy, infrastructure, skills and jobs, trust and digital security, and government [ 119 ]. At the regional level, the Flemish government further developed transformation policies with sustainability as a leading principle and knowledge development as a driving force. In Wallonia, the smart specialisation strategy becomes the guiding policy framework.
The most eye-catching initiative of Belgium is the so-called “Made Different”, launched in 2012. It is organised by the Belgian federation of the technology industry, Agoria, and Belgian Collective Research Centre, Sirris. They have over 1800 and 2500 member companies, respectively. “Made Different” is an industry-led, bottom-up programme to provide manufacturing companies with advisory services, from many dedicated experts. This customisation service takes into account of each company’s practical situations and advises according to several transformation perspectives: world-class manufacturing technologies; end-to-end engineering; digital factory; human-centred production; production network; eco-production; and smart production system [ 119 ]. Over 300 companies have already completed or in the process of one or more these transformations by the end of 2017.
Denmark ranked 1st out of the 28 EU member states in 2017 and 4th in the latest overall results in the Digital Economy and Society Index. With the broadest 4G converge in Europe and fast connections, Denmark ranked 1st on Connectivity and Use of Internet Services. The iconic “MADE”, stands for Manufacturing Academy of Denmark, started in 2013 is a bottom-up initiative, collaborated between Danish manufacturing companies, five universities, three research and technology organisations. It is similar to Belgium’s initiative but is partly funded by the government as well as the private sector, with the budget totalling around €50 million from 2014 to 2019. The Danish government allows “MADE” to flourish without much state intervention. Such a risky approach paid off: 15 companies participating in various projects reported revenue increases of €135,000 and collective savings of around €5.5 million by the end of 2019 [ 75 ]. In December 2016, “MADE Digital” was launched with a further €26 million governmental funding to strengthen Danish manufacturing and expand participating members.
Denmark has maintained a consistent long-term national policy at delivering online public services and the “Digital Strategy 2016–2024” is amongst latest governmental plans, presented in May 2016 [ 120 ]. It is to enhance close public sector collaboration and deliver efficient, coherent services to the public and businesses. Three general objectives have been listed [ 120 ]:
all citizens have a share in the benefits of digitisation;
business unlock the growth and small- and medium-sized enterprises need at digital upgrade;
good digital framework conditions.
As early as in 2012, there were warnings that the French industry was suffering from significant under-investment in digitisation. To maintain long-term competitiveness, the French government has launched many initiatives and programmes. For example, in September 2013, the “La Nouvelle France Industrielle” (New Industrial France) and the strategic programme “Investissements d’Avenir” (Invest for the Future) with €47 billion was set up to support innovative projects on fundamental research, innovation, technology transfer and maturation [ 121 ]. Subsequently, there have been many industrial plans, but individual programmes were challenging to draw board collaborations. As a consequence, the French government selected 34 industrial plans and the cross-cutting “Industrie du Futur” (Industry of the Future) was launched in April 2015. It aims to support French companies to deploy digital technologies, transform business models and modernise production practices. In detail, this France initiative comprises five pillars,
cutting-edge technologies: to support companies with research funding, subsidies and loans;
business transformation: over 550 experts to help over 2000 small- and medium-sized enterprises identify transformation projects by 2016;
training: to upskill the workforce, create future-joint visions with unions and develop training programmes and curricula;
international cooperation: to establish alliances such as a bilateral approach with Germany on standardisation.
self-promotion: to boost French interest at the European level and its “Creative France Industry” brand.
It gained popularity and quickly found supports from the government, industry, technology and research stakeholders as well as trade unions. Approximately €10 billion has been made available from public sources, €550 million on calls for projects, €250 million for this initiative programme, €100 million for staff training, €4.2 billion for small- and medium-sized enterprises in the form of loans and €5 billion through tax aid for investments for the 2014–2020 period [ 121 ]. To encourage private investments, parts of the funding requires the private sector to invest in the same amount as the government. Through the tax-aid route, every €100 million spent in public fund induces €500 million from private financing.
As the birthplace of Industry 4.0, Germany has made its brand and fortified it with its strong industrial sectors. The first use of the term “Industrie 4.0” can be traced back to 2006 when the German government launched the “High-Tech 2020 Strategy”. In April 2011, three engineers held a press conference at Hannover Fair: Dr Henning Kagermann of the National Academy of Science and Engineering; Dr Wolfgang Wahlster of the German Research Centre for Artificial Intelligence; and Dr Wolf-Dieter Lukas from the Federal Ministry of Research and Education [ 75 ]. The world quickly embraces this technological revolution.
This German initiative has become a global platform, serves as a central point of contact for policy-makers. Approximately 15 million jobs in Germany are either directly or indirectly linked to the new digital evolution. The Boston Consulting Group predicts €90–150 billion worth of benefits over the next decade [ 122 ].
In April 2013, the “Plattform Industrie 4.0” was created by three private associations: the Federal Association for Information Technology, Telecommunications and New Media; the German Association; and the Electrical and Electronic Manufacturers’ Association [ 75 ]. It expanded in 2015 by including companies, associations, trade unions, science and politics nationwide. Today, it has over 300 active players from 159 organisations. It is also cooperating with the “Industrial Internet Consortium” with the USA, the “Alliance Industrie du Futur” with France, the “Robot Revolution Initiative” with Japan and a memorandum of understanding with China [ 133 ].
The German success demonstrates the powerful impact on a global scale from a fledgeling industrial movement. The combination of national-level-policy support and cross-industry technological innovation presents a model worth copying.
In comparison, the “Impresa 4.0”, Italian Industry 4.0 national plan is relatively recent. It was first presented in late September 2016 in Milan and officially launched in February 2017 by the government. This is a top-down approach initiated by the Italian government, with academia, business associations and trade unions actively involved in the Steering Committee [ 134 ]. This national plan has two main focus areas. First, it supports the use of innovative technologies, digital transformation, and in turn, boosts Italian competitiveness. Second, it aims to develop skills through digital innovation hubs, competence centres, education programmes, vocational training and industrial PhDs.
The Italian Ministry of Economic Development details the following measures as its governmental top-down approach [ 123 ].
“Hyper- and super-depreciation”: offering incentives to companies that invest in digital and technological transformation with new capital goods and assets.
“Nuova Sabatini”: offering bank loans to support digital technologies (both hardware and software) in production.
“Tax credit for research and development”: encouraging private investment to ensure and improve the competitiveness.
“Patent box”: attracting investors with a special rate of taxation on intellectual property rights; bringing back aboard assets and keeping domestic assets relocating; favouring research investments.
“Innovative startups and small- and medium-sized enterprises”: supporting and sustaining Italian startup ecosystem.
“Guarantee fund for small- and medium-sized enterprises”: granting sufficient guarantees for businesses and professionals to access bank loans.
“Development contracts”: reducing minimum investment threshold in specific sectors.
“Innovation agreements”: providing financial supports to “Horizon 2020”-related projects.
“Tax credit for Training 4.0”: offering expenditures in relevant training and filling the skill gap.
“Fund for intangible capital, competitiveness and productivity”: funding in strategic areas for both public and private entities globally in line with the national plan.
Thirty Japanese companies, including Mitsubishi Electric, Fujitsu, Nissan Motor and Panasonic commenced an Industrial Value Chain Initiative in 2015. This initiative following the German Industry 4.0 plan, combines manufacturing and information technologies to promote industrial collaboration [ 135 ]. However, this did not satisfy the Japanese ambition. Thinking outside of the box, all previous industrial revolutions, by implementing the most advanced technologies at the time, eventually were to improve the wider society and individual’s wellbeing. Therefore, in 2019, the concept of “Society 5.0” was launched by the Japanese [ 124 ], following what can be called the hunting society (Society 1.0), agricultural society (Society 2.0), industrial society (Society 3.0) and information society (Society 4.0).
“Society 5.0” outlined a basic plan for a future that is benefited by the full implementation of Industry 4.0. The Japanese Cabinet Office defines it as “a human-centred society that balances economic advancement with the resolution of social problems by a system that highly integrates cyberspace and physical space” [ 124 ]. Within our current information society, humans may actively seek relevant information about their needs. This can be seen as a limitation and a burden to individuals. The fundamental concept of “Society 5.0” is by integrating people, things and systems in cyberspace, so that information will be analysed and pushed to individually tailored to each specific needs. This can lead an active and enjoyable life.
The implementation of “Society 5.0” will involve large information collection via various physical space terminals, such as sensors, user inputs to the electronics, big data is then analysed with each individual’s parameters, and results are fed back in different forms. This concept uses the industrial revolution to balance economic advancement and personalised needs and eventually provide an optimal solution to the social problems that we are currently facing. In [ 124 ], many examples in different fields are discussed, including mobility, healthcare and care-giving, manufacturing, agriculture, food, disaster prevention and energy.
The Netherlands
The Netherlands launched its Smart Industry initiative in November 2014. It seeks to utilised existing knowledge and accelerates Information and Communication Technology (ICT) in industry. The Netherlands has a robust ICT infrastructure and a tradition of collaboration in clusters and networks. In comparison, the Smart Industry is on a relatively low budget and limited resources. Thus, with a well-built network-centric production, a boost in the Dutch digital transformation is achievable.
The Smart Industry is a bottom-up approach. It is based on public funding from the government (€25 million for 3 years) and European regional funds (€10 million) [ 125 ]. However, financial contributions from industry and private sectors are expected to cover over 50% of the costs. The Dutch also introduced the concept of “Triple Helix” alludes, which focusses on collaboration between universities, industry and government.
The Dutch government outlined a three-line action plan for rolling out its Industry 4.0 [ 125 ]. First, it provides companies with technological and market understanding, up-to-date best practices from existing knowledge. Second, it aims to build national and regional ecosystems and interrelated networks of industries, hence accelerate innovative and technological practices. The third action is a long-term vision to improve knowledge, skills and ICT conditions.
People’s Republic of China
For the past 4 decades, a concerted effort has been made and China has become an industrial leader. China became the largest exporter in 2010, the largest trading nation in 2013 and the largest economy in 2016, according to the World Factbook [ 75 ]. Its industries around material processing, such as iron, steel, aluminium, coal, and textiles, food, cement, automobile, trains, ships and electronics are amongst the highest gross outputs in the whole world. However, the majority of the Chinese industries are regarded as relatively low skilled, heavily manual-labour-dependent and obvious gaps of innovations in its industrial chain. The Chinese ambition, namely “Made in China 2025” set out in 2015, and was preciously to change its landscape [ 126 ]. Its aim was to upgrade and accelerate technological development, re-structure its manufacturing strategies to adapt competitions from other low-labour-cost countries, and promote Chinese brands. One additional point is that process industries play a major role in the making of this strategy. It is a big component in the Chinese economic and manufacturing. Its advancements will contribute to automation, computer and communication and data science, which are all fundamental challenges to achieve Industry 4.0 [ 136 ].
The underlying philosophy of “Made in China 2025” is to establish its independence from resources, research and technologies of other countries. The principles extends to innovation, high-quality manufacturing, green development, structure optimisation and training its workforce [ 75 ]. There are four stages of this plan. First, by 2020, it is to increase industrialisation, digitalisation, develop core technologies in key areas, strengthen competitiveness and reduce industry pollution. The second stage is set for 2025, focusing on improving quality in all aspects, increasing information technology at an advanced level, and achieving a reduction to pollution to global standards. Third, by 2035, Chinese manufacturing would reach the median level amongst global leaders, improve upon innovation capability and make breakthroughs in major areas. Finally, when it comes to 2049, Chinese industries should be a leading force on the global stage and holding a significant competitive advantage in major manufacturing areas.
“Indústria 4.0”, the Portuguese strategy for Industry 4.0 was launched in January 2017. The Portuguese vision is oriented around three axes: digitalisation, innovation and training. In comparison, the noticeable differences are that it mainly targets small- and medium-sized enterprises and has a strong focus on upskilling the workforce. Over 200 Portuguese companies (120 of which were small- and medium-sized enterprises) participated in the design of the strategy. It aims to have an impact on over 50,000 companies and train over 20,000 workers in Information and Communications Technology skills [ 127 ].
It is a bottom-up approach, initiated by the Ministry of Economy but is managed by COTEC, a private company. €4.5 billion has been made available through Portugal 2020 ERDF funds, and the private sector is expected to invest another half of the measures. €7,500 per each application has been distributed in vouchers to support small- and medium-sized enterprises’ digital transformation. Universities such as Minho and Aveiro have also been participating in the development of digitalisation [ 127 ].
Technology and innovation have been one of Singapore’s traditional cornerstones. Singapore’s universities, such as the National University of Singapore and the Nanyang Technological University, provide world-leading research and development. Together with global collaborations with universities in Asia, Australia, the United States, etc., Singapore benefits from world-renowned research outputs.
Singapore has been focusing to develop a knowledge-based innovation-driven economy and society for over 30 years. Funding from the government to promote innovation and science has been steadily increasing. Every 5 years, Singapore provides a national strategic plan. It started in the “National Technology Plan 1995” with $2 billion. The latest is the “Research, Innovation and Enterprise 2020 Plan” and provides $19 billion from the public investment [ 128 ]. The targeted domains and programmes are separated into seven categories: advanced manufacturing and engineering, health and biomedical sciences, urban solutions and sustainability, services and digital economy, academic research, manpower, and innovation and enterprise.
South Korea
In 2014, South Korean launched its “Manufacturing Industry Innovation 3.0” strategy. A total of $376 million was invested in developing smart manufacturing technologies. There were four categories: smart manufacturing proliferation, creative economy, smart innovation and business re-organisation [ 129 ].
The Spanish strategy, “Industria Conectada 4.0”, was announced in 2014, aimed at digitising and enhancing the competitiveness of Spanish industrial sector [ 130 ]. There have been many resources allocated, such as €97.5 million in loans for innovation and €68 million for Information and Communication Technology companies. The main objectives are threefold [ 130 ]:
improve industrialisation and employment in the relevant sectors;
encourage the development of a Spanish industrial model;
enhance the local supply of digital solutions and boost Spanish industries.
At the initial stage of policymaking, one of the issues was on how to motivate the Spanish industry to participate. To address this, the Spanish government established a strategic group: the Santander bank to provide digital financing knowledge; Telefonica to provide telecommunications; Indra to become the technological consultancy [ 130 ]. Its focus is on Spanish small- and medium-sized enterprises and micro-enterprises. Micro-enterprises are those typically employ fewer than ten people and less than €2 million in turnover.
The United Kingdom
The United Kingdom (the UK) has been traditionally recognised for its strong research and innovation. It is ranked the second for the “Inward Foreign Direct Investment” in 2017 by the United Nations Conference on Trade and Development. The urge for modern innovative technological development can be traced back before the German Industry 4.0 concept [ 131 ]. In 2004, the Department of Trade and Industry established the Technology Strategy Board. It became an independent advisory body, namely Innovate UK, in 2007. In 2017, the Higher Education and Research Act 2017 was passed into law by the House of Parliament. The following year, seven research councils, Innovate UK and parts of the Higher Education Funding Council for England merged with the UK Research and Innovation organisation that directs research funding [ 137 ], all of which share a common purpose, that is, to help develop innovative technologies.
The UK’s industrial strategy is built on nearly 2000 formal responses from all types of organisations in the wide society. It lists five foundations [ 138 ]: ideas, people infrastructure, business environment and places. We summarise each of them here. First, the aim is to develop the world’s most innovative economy, by raising investment equivalent to 2.4% of GDB by 2027, increasing tax credit to 12%, and investing £725 million to the funding programme. Second, the UK is to provide good jobs and greater earning for the public, by establishing technical education systems, investing £406 million to relevant educational departments, and £64 million for re-training people in latest technical fields. Third, £31 billion is to be invested in general infrastructure, £400 million in hardware related to electric vehicles, and over £1 billion digital infrastructure such as 5G and full-fibre networks. Fourth, the UK is set to increase its productivity with £20 billion in high potential businesses. Finally, £1.7 billion is to be invested for intra-city transport and £42 million to pilot a Teacher Development Premium.
On top of this industrial strategy, the UK has set out four Grand Challenges: artificial intelligence and data revolution, clean growth, future of mobility, and ageing society. It promotes interdisciplinary research and development from industry, universities and the government.
The United States of America
In 2011, the USA launched the Advanced Manufacturing Partnership, which aims to bring together industry, academics and the federal government [ 132 ]. This scheme focusses on emerging technologies to create high-quality manufacturing and boost its global competitiveness. $500 million is to be invested as the initial step, and the plan includes four main steps: building domestic manufacturing capabilities in critical industries; reducing the time to develop and deploy advanced materials; next-generation robotics; and developing innovative energy-efficient manufacturing techniques. The following year, General Electric introduced the concept of the Industrial Internet of Things, emphasises on the integration of artificial intelligence, analytics and connected people [ 7 ]. In 2014, with the support of many industries such as General Electric, Intel, IBM, etc., the Industrial Internet Consortium was setup. The main principle of the Industrial Internet is centred around three components: intelligent equipment, intelligent systems and intelligent decision-making.
Soon after, almost all Industrial 4.0 topics have been introduced within the USA. In 2016, the name “Manufacturing USA” was adopted by the National Institute of Standards and Technology. In February 2020, this institute described a strategy to expand the Manufacturing USA network [ 139 ]. Multiple pathways were introduced to included innovation and manufacturing centres which are not receiving federal sponsorship.
From the launch of Industry 4.0 in 2011 to the current day, its influence has been felt across almost all sectors. Many governments have started their tailored policies to support this technological revolution. However, we are still in the beginning of its transition. In this paper, we have looked at the concepts of Industry 4.0. Although we summarise them with its 9-pillar scheme that was initially described, many descriptions have further shifted, highlighting an individualization paradigm. In “Industry 4.0 concepts”, we went through cyber-physical systems, Internet of Things, big data and data analytics, cloud and information technology, robots and automated machinery, 3D printing, simulation, portable devices and Industry 4.0 challenges. From the existing literature, it is clear and have been repeatedly highlighted that within the industries, an interdisciplinary approach is essential to the Industry 4.0 development. In Addition, it is widely accepted that an international coordination and governmental support are necessary. For the first time in this paper, we summarise a range of governmental industrial plans and policies, including Belgium, Denmark, France, Germany, Italy, Japan, the Netherlands, China, Portugal, Singapore, South Korea, Spain, the UK and the US. We can see in many countries, the idea of an industrial revolution has long been on the horizon before 2011. Countries are tailoring their plans in accordance with their strength. We also see international efforts such as the “Trilateral Cooperation”. Through this paper, we would like to communicate to the reader, wherever you are and whatever sector you work in, embrace the positives this revolution is to offer and be ready to change.
Lasi H, Fettke P, Kemper HG, Feld T, Hoffmann M (2014) Industry 4.0. Bus Inf Syst Eng 6:239–242
Article Google Scholar
Chen B, Wan J, Shu L, Li P, Mukherjee M, Yin B (2017) Smart factory of Industry 4.0: key technologies, application case, and challenges. IEEE Access 6:6506–6519
Google Scholar
Pereira AC, Romero F (2017) A review of the meanings and the implications of the Industry 4.0 concept. Procedia Manuf 13:1206–1214
Vaidya S, Ambad P, Bhosle S (2018) Industry 4.0—a glimpse. Procedia Manuf 20:233–238
Roblek V, Mesko M, Krapez A (2016) A complex view of Industry 4.0. SAGE Open 2:1–11
Xu LD, Xu EL, Li L (2018) Industry 4.0: state of the art and future trends. Int J Prod Res 56(8):2941–2962
Zhong RY, Xu X, Klotz E, Newman ST (2017) Intelligent manufacturing in the context of Industry 4.0: a review. Engineering 3:616–630
Lu Y (2017) Industry 4.0: a survey on technologies, applications and open research issues. J Ind Inf Integr 6:1–10
Lukac D (2015) The fourth ICT-based industrial revolution “Industry 4.0”—HMI and the case of CAE/CAD innovation with EPLAN P8. In: 2015 23rd Telecommunications forum Telfor (TELFOR). IEEE, New York, pp 835–838
Baur C, Wee D (2015) Manufacturing’ next act. McKinsey, New York
Georgakopoulos D, Jayaraman PP, Fazia M, Villari M, Ranjan R (2016) Internet of things and edge cloud computing roadmap for manufacturing. IEEE Cloud Comput 3(4):66–73
Kube G, Rinn T (2014) Industry 4.0—the next revolution in the industrial sector. ZKG Int 67(11):30–32
Lin F, Chen C, Zhang N, Guan X, Shen X (2015) Autonomous channel switching: towards efficient spectrum sharing for industrial wireless sensor networks. IEEE Internet Things J 3(2):231–243
Lom M, Pribyl O, Svitek M (2016) Industry 4.0 as a part of smart cities. In: 2016 Smart cities symposium Prague (SCSP). IEEE, New York, p 16107786
Pfeiffer S (2016) Robots, Industry 4.0 and humans, or why assembly work is more than routine work. Societies 6(2):16
Singer P (2016) Are you ready for Industry 4.0? Solid State Technol 58(8):2
Thames L, Schaefer D (2016) Software-defined cloud manufacturing for industry 4.0. Procedia CIRP 52:12–17
Vijaykumar MR (2015) Design and implementation of RVJCS security model. In: 2015 International conference on circuits, power and computing technologies [ICCPCT-2015]. IEEE, New York, p 15295584
Wan J, Tang S, Shu Z, Li D, Wang S, Imran M, Vasilakos A (2016) Software-defined industrial Internet of Things in the context of Industry 4.0. IEEE Sens J 16(22):7373–7380
Posada J, Toro C, Barandiaran I, Oyarzun D, Stricker D, de Amicis R, Vallarino I (2015) Visual computing as a key enabling technology for Industry 4.0 and industrial internet. IEEE Comput Graph Appl 35(2):26–40
Jeon B, Yoon JS, Um J, Suh SH (2020) The architecture development of Industry 4.0 compliant smart machine tool system. J Intell Manuf
Wollschlaeger M, Sauter T, Jasperneite J (2017) The future of industrial communication: automation networks in the era of the Internet of Things and Industry 4.0. IEEE Ind Electron Mag 11(1):17–27
Li X, Li D, Wan J, Vasilakos AV, Lai CF, Wang S (2017) A review of industrial wireless networks in the context of Industry 4.0. Wirel Netw 23:23–41
Wang S, Wan J, Zhang D, Li D, Zhang C (2016) Towards smart factory for Industry 4.0: a self-organized multi-agent system with big data based feedback and coordination. Comput Netw 101:158–168
Zhou K, Liu T, Zhou L (2015) Industry 4.0: towards future industrial opportunities and challenges. In: 12th International conference on fuzzy systems and knowledge discovery (FSKD), pp 2147–2152
Pfohl HC, Yahsi B, Kurnaz T (2015) The impact of Industry 4.0 on the supply chain. In: Innovations and strategies for logistics and supply chains: technologies, business models and risk management. Proceedings of the Hamburg international conference of logistics (HICL), vol 20. epubli GmbH, Berlin, pp 31–58
Heng S (2015) Industry 4.0: upgrading of Germany’s industrial capabilities on the horizon. SSRN
Sung TK (2018) Industry 4.0: a Korea perspective. Technol Forecast Soc Change 132:40–45
Benesova A, Tupa J (2017) Requirements for education and qualification of people in Industry 4.0. Procedia Manuf 11:2195–2202
Jayanthi P, Iyyanki M, Mothkuri A, Vadakattu P (2020) Fourth industrial revolution: an impact on health care industry. In: Advances in artificial intelligence, software and systems engineering. AHFE 2019. Advances in intelligent systems and computing, p 965
Lorenz M, Rubmann M, Strack R, Lueth KL, Bolle M (2015) Man and machine in Industry 4.0—how will technology transform the industrial workforce through 2025? The Boston Consulting Group
Botlikova M, Botlik J(2020) Local extremes of selected Industry 4.0 indicators in the European space—structure for autonomous systems. J Risk Financ Manag 13
Sim S, Choi H (2020) A study on the service discovery support method in the IoT environments. Int J Electr Eng Educ 57(1):85–96
Lee J, Bagheri B, Kao HA (2015) A cyber-physical systems architecture for Industry 4.0-based manufacturing systems. Manuf Lett 3:18–23
Liu Y, Xu X (2017) Industry 4.0 and cloud manufacturing: a comparative analysis. J Manuf Sci Eng 139:034701
Buchi G, Cugno M, Castagnoli R (2020) Smart factory performance and Industry 4.0. Technol Forecast Soc Change 150:119790
Stock T, Seliger G (2016) Opportunities of sustainable manufacturing in Industry 4.0. Procedia CIRP 40:536–541
Uhlemann THJ, Lehmann C, Steinhilper R (2017) The digital twin: realizing the cyber-physical production system for Industry 4.0. Procedia CIRP 61:335–340
Liao Y, Deschamps F, Loures EFR, Ramos LFP (2017) Past, present and future of Industry 4.0—a systematic literature review and research agenda proposal. Int J Prod Res 55(12):3609–3629
Slusarczyk B (2018) Industry 4.0—are we ready? Pol J Manag Stud 17(1):232–249
Vogel-Heuser B, Hess D (2016) Guest editorial Industry 4.0—prerequisites and visions. IEEE Trans Autom Sci Eng 13(2):411–413
Mosconi F (2015) The new European industrial policy: global competitiveness and the manufacturing renaissance. Routledge, London
Book Google Scholar
Dais S (2014) Industry 4.0—offense, vision, approach. In: Industry 4.0 in production, automation and logistics. Application, technologies and migration. Springer, Wiesbaden, pp 625–634
Barreto L, Amaral A, Pereira T (2017) Industry 4.0 implications in logistics: an overview. Procedia Manuf 13:1245–1252
Tjahjono B, Esplugues C, Ares E, Pelaez G (2017) What does Industry 4.0 mean to supply chain? Procedia Manuf 13:1175–1182
Kolberg D, Zuhlke D (2015) Lean automation enabled by Industry 4.0 technologies. IFAC PapersOnLine 48(3):1870–1875
Zezulka F, Marcon P, Vesely I, Sajdl O (2016) Industry 4.0—an introduction in the phenomenon. IFAC PapersOnLine 49(25):8–12
Qin J, Liu Y, Grosvenor R (2016) A categorical framework of manufacturing for Industry 4.0 and beyond. Procedia CIRP 52:173–178
Bartodziej CJ (2017) The concept Industry 4.0—an empirical analysis of technologies and applications in production logistics. Springer Fachmedien Wiesbaden, Wiesbaden
Ustundag A, Cevikcan E (2018) Industry 4.0: managing the digital transformation. Springer series in advanced manufacturing
Rojko A (2017) Industry 4.0 concept: background and overview. Int J Interact Mob Technol 11(5)
Gorecky D, Schmitt M, Loskyll M, Zuhlke D (2014) Human–machine-interaction in the Industry 4.0 era. In: 2014 12th IEEE international conference on industrial informatics (INDIN), p 14718826
Varghese A, Tandur D (2015) Wireless requirements and challenges in Industry 4.0. In: Proceedings of 2014 international conference on contemporary computing and informatics, IC3I 2014, pp 634–638
Shrouf F, Ordieres J, Miragliotta G (2014) Smart factories in Industry 4.0: a review of the concept and of energy management approached in production based on the internet of things paradigm publisher: IEEE. In: 2014 IEEE international conference on industrial engineering and engineering management, p 14983686
Angelopoulos A, Michailidis ET, Nomikos N, Trakadas P, Hatziefremidis A, Voliotis S, Zahariadis T (2020) Tackling faults in the Industry 4.0 era—a survey of machine-learning solutions and key aspects. Sensors 20(1):109
Seyedghorban Z, Tahernejad H, Meriton R, Graham G (2020) Supply chain digitalization: past, present and future. Prod Plan Control 31:96–114
Ramos L, Loures E, Deschamps F, Venancio A (2019) System evaluation methodology to attend the digital projects requirements for Industry 4.0. Int J Comput Integr Manuf
Yin Y, Stecke KE, Li D (2018) The evolution of production systems from Industry 2.0 through Industry 4.0. Int J Prod Res
Tien JM (2019) Towards the fourth industrial revolution on real-time customization. J Syst Sci Syst Eng
Morrar R, Arman H, Mousa S (2017) The fourth industrial revolution (Industry 4.0): a social innovation perspective. Technol Innov Manag Rev 7(11):12–20
Rostow W (1988) Essays on a half century ideas, politics and action. Westview Press, Boulder
Shafiq SI, Sanin C, Toro C, Szczerbicki E (2015) Virtual engineering object (VEO): toward experience-based design and manufacturing for Industry 4.0. Cybern Syst 46:35–50
Shafiq SI, Sanin C, Szczerbicki E, Toro C (2016) Virtual engineering factory: creating experience base for Industry 4.0. Cybern Syst 47:32–47
Ji C, Shao Q, Sun J, Liu S, Pan L, Wu L, Yang C (2016) Device data ingestion for industrial big data platforms with a case study. Sensors 16(3):279
Ning H, Liu H (2015) Cyber-physical-social-thinking space based science and technology framework for the Internet of Things. Sci China Inf Sci 58:1–19
Cooper J, James A (2009) Challenges for database management in the Internet of Things. IETE Tech Rev 26:320–329
Dominici G, Roblek V, Abbate T, Tani M (2015) “Click and drive”: consumer attitude to product development. towards future transformations for driving experience. Bus Process Manag J 22:420–434
Marolt M, Pucihar A, Zimmermann DH (2015) Social CRM adoption and its impact on performance outcomes: a literature review. Organizacija 48:260–271
Roblek V, Bach M, Mesko M, Bertoncelj A (2013) The impact of social media to value added. Kybernetes 42:554–568
Rodriguez M, Trainor K (2016) A conceptual model of the drivers and outcomes of mobile CRM application adoption. J Res Interact Mark 10:67–84
Kagermann H (2015) Change through digitization - value creation in the age of Industry 4.0. In: Management of permanent change. Springer, Wiesbaden, pp 23–45
Yu J, Subramanian N, Ning K, Edwards D (2015) Product delivery service provider selection and customer satisfaction in the era of Internet of Things: a Chinese e-retailers’ perspective. Int J Prod Econ 159:104–116
Hessman T (2013) The down of a smart factory. Technical report, Industry Week. https://www.industryweek.com/technology-and-iiot/article/21959512/the-dawn-of-the-smart-factory . Accessed Feb 2020
European-Commission (2015) Program smart cities. Technical report, The EU. https://ec.europa.eu/energy/en/content/program-smart-cities . Accessed Feb 2020
Proctor M, Wilkins J (2019) 4.0 Sight—digital industry around the world. Technical report, EU Automation. http://www.4sightbook.com/ . Accessed Feb 2020
De Silva P, De Silva P (2016) Ipanera: an Industry 4.0 based architecture for distributed soil-less food production systems. In: Proceedings of the 1st manufacturing and industrial engineering symposium, Colombo, Sri Lanka
Zhou K, Liu T, Liang L (2016) From cyber-physical systems to Industry 40: make future manufacturing become possible. Int J Manuf Res 11(2):167–188
Mladineo M, Veza I, Gjeldum N (2017) Solving partner selection problem in cyber-physical production networks using the HUMANT algorithm. Int J Prod Res 55(9):2506–2521
Baheti R, Gill H (2011) Cyber-physical systems. In: The impact of control technology: overview, success stories, and research challenges. IEEE Control System Society, New York, pp 161–166
Lee EA (2008) Cyber physical systems: design challenges. In: Proceedings of the 11th IEEE symposium on object/component/service-oriented real-time distributed computing. The Institute of Electrical and Electronics Engineers, Piscataway, pp 363–369
Erol S, Jager A, Hold P, Ou K, Sihn W (2016) Tangible Industry 4.0: a scenario-based approach to learning for the future of production. In: 6th CLF—6th CIRP conference on learning factories, procedia CIR, vol 54, pp 13–18
Tan Y, Goddard S, Perez IC (2008) A prototype architecture for cyber-physical systems. ACM SIGBED Rev 5(1):26–27
Yin Y, Zeng Y, Chen X, Fan Y (2016) The internet of things in healthcare: an overview. J Ind Inf Integr 1:3–13
Nunez D, Borsato M (2017) An ontology-based model for prognostics and health management of machines. J Ind Inf Integr 6:33–46
Chen H (2017) Applications of cyber physical systems—a literature review. J Ind Integr Manag 2(3)
Nayak A, Levalle R, Lee S, Nof S (2016) Resource sharing in cyber-physical systems: modelling framework and case studies. Int J Prod Res 54(23):6969–6983
Derler P, Lee EA, Vincentelli AS (2012) Modeling cyber-physical systems. Proc IEEE 100(1):13–28
Neugebauer R, Hippmann S, Leis M, Landherr M (2016) Industrie 4.0: form the perspective of applied research. In: 49th CIRP conference on manufacturing systems, pp 2–6
Lund D, MacGillivray C, Turner V, Morales M (2014) Worldwide and regional internet of things (IoT) 2014–2020 forecast: a virtuous circle of proven value and demand. In: Framingham: International Data Corporation. Report No. IDC 248451
Friess P (2016) Digitising the industry—Internet of Things connecting the physical, digital and virtual worlds. River Publishers, Hakodate
Gilchrist A (2016) Industry 4.0. Apress, Berkeley
Ashton K (2009) That Internet of Things thing. RFID J 22:97–114
Xu L, He W, Li S (2014) Internet of things in industries: a survey. IEEE Trans Ind Inform 10(4):2233–2243
Farooq MU, Waseem M, Mazhar S, Khairi A, Kamal T (2015) A review on internet of things. Int J Comput Appl 113(1):1–7
van Kranenburg R (2008) The Internet of Things: a critique of ambient technology and the all-seeing network of RFID. Institute of Network Cultures, Amsterdam
van Kranenburg R, Anzelmo E, Bassi A, Caprio D, Dodson S, Ratto M (2011) The internet of things. In: Proceedings of 1st Berlin symposium on internet and society, Berlin, Germany, pp 25–27
Li S, Xu L, Wang X (2013) Compressed sensing signal and data acquisition in wireless sensor networks and internet of things. IEEE Trans Ind Inform 9(4):2177–2186
Fan Y, Yin Y, Xu L, Zeng Y, Wu F (2014) IoT based smart rehabilitation system. IEEE Trans Ind Inform 10(2):1568–1577
He W, Xu L (2014) Integration of distributed enterprise applications: a survey. IEEE Trans Ind Inform 10(1):35–42
Article MathSciNet Google Scholar
Kang K, Pang Z, Xu L, Ma L, Wang C (2014) An interactive trust model for application market of the internet of things. IEEE Trans Ind Inform 10(2):1516–1526
Perrey J, Spillecke D, Umblijs A (2013) Smart analytics: how marketing drives short-term and long-term growth. McKinsey and Company, New York
Zhang TK, Ma YF, Xu DP, Liu CX, Ding JL (2020) ihppvis: Interactive visual analysis of industrial data in heavy plate production. In: The 21st IFAC World Congress 2020, Germany, July 11–19
Xu X (2012) From cloud computing to cloud manufacturing. J Robot Comput Integr Manuf 28(1):75–86
Wang L, Haghighi A (2016) Combined strength of holons, agents and function blocks in cyber-physical systems. J Manuf Syst 40(2):25–34
Buyya R, Yeo CS, Venugopal S, Broberg J, Brandic I (2009) Cloud computing and emerging it platforms: vision, hype, and reality for delivering computing as the 5th utility. Future Gener Comput Syst 25(6):599–616
Bayram B, Ince G (2018) Advances in robotics in the era of industry 4.0. In: Industry 4.0: managing the digital transformation. Springer series in advanced manufacturing
Malý I, Sedláček D, Leitão P (2016) Augmented reality experiments with industrial robot in Industry 4.0 environment. In: 2016 IEEE 14th international conference on industrial informatics (INDIN), pp 176–181
Schuh G, Potente T, Wesch-Potente C, Weber AR (2014) Collaboration mechanisms to increase productivity in the context of Industrie 4.0. Procedia CIRP 19:51–56
Wang S, Wan J, Li D, Zhang C (2016) Implementing smart factory of Industrie 4.0: an outlook. Int J Distrib Sens Netw 1–10
Thoben KD, Wiesner S, Wuest T (2017) Industrie 4.0 and smart manufacturing—a review of research issues and application examples. Int J Autom Technol 11(1):4–16
Fernández-Caramés TM, Fraga-Lamas P (2019) A review on the application of blockchain to the next generation of cybersecure industry 4.0 smart factories. IEEE Access 7:45201–45218
Mohamed N, Al-Jaroodi J (2019) Applying blockchain in Industry 4.0 applications. In: 2019 IEEE 9th annual computing and communication workshop and conference (CCWC), pp 0852–0858
Alladi T, Chamola V, Parizi RM, Choo KR (2019) Blockchain applications for industry 4.0 and industrial IoT: a review. IEEE Access 7:176935–176951
Hansen R, Sia SK (2015) Hummel’s digital transformation towards omnichannel retailing: key lessons learned. MIS Q Executive 14:51–66
Kane GC, Palmer D, Phillips AN, Kiron D (2015) Is your business ready for a digital future? MIT Sloan Manag Rev 56:37
Hungerland F, Quitzau J, Zuber C, Ehrlich L, Growitsch C, Rische MC, Hab HJ (2015) The digital economy (No. 21e). Technical report, Strategy 2030—Wealth and Life in the Next Generation. https://www.econstor.eu/handle/10419/121322 . Accessed Feb 2020
Weber E (2015) Industry 4.0 impact on the economy and labor market. Wirtschaftsdienst 95:722–723
Australian Government (2017) Industry 4.0 Testlabs in Australia. Technical report, Department of Industry, Science, Energy and Resources. https://www.industry.gov.au/data-and-publications/industry-40-testlabs-in-australia#:~:text=Industry%204.0%20Testlabs%20were%20a,4.0%20technologies%20and%20workforce%20transformation . Accessed Dec 2020
Larosse J (2017) Analysis of national initiatives on digitising European industry: Belgium. Technical report, European Commission—Futurium. https://ec.europa.eu/futurium/en/system/files/ged/be_country_analysis.pdf . Accessed Feb 2020
Digital-Transformation-Monitor (2017) Denmark: manufacturing academy of Denmark (MADE). Technical report, European Commission. https://ec.europa.eu/growth/tools-databases/dem/monitor/sites/default/files/DTM_MADE_DK%20v3_0.pdf . Accessed Feb 2020
Digital-Transformation-Monitor (2017) France: Industrie du Futur. Technical report, European Commission. https://ec.europa.eu/growth/tools-databases/dem/monitor/sites/default/files/DTM_Industrie%20du%20Futur%20v1.pdf . Accessed Feb 2020
Digital-Transformation-Monitor (2017) Germany: Industrie 4.0. Technical report, European Commission. https://ec.europa.eu/growth/tools-databases/dem/monitor/sites/default/files/DTM_Industrie%204.0.pdf . Accessed Feb 2020
Ministry of Economic-Development (2017) National Industry 4.0 Plan (Impresa 4.0). Technical report, Governo Italiano. https://www.mise.gov.it/index.php/en/202-news-english/2036690-national-industry-4-0-plan . Accessed Feb 2020
Cabinet-Office of Japan (2018) Society 5.0. Technical report, The Government of Japan. https://www8.cao.go.jp/cstp/english/society5_0/index.html . Accessed Feb 2020
Digital-Transformation-Monitor (2017) The Netherlands: Smart Industry. Technical report, European Commission. https://ec.europa.eu/growth/tools-databases/dem/monitor/sites/default/files/DTM_Smart%20Industry%20v1.pdf . Accessed Feb 2020
(2015) http://www.gov.cn . Premier Li pushes forward “Made-in-China 2025” at State Council meeting. Technical report, The State Council of the People’s Republic of China. http://english.www.gov.cn/premier/news/2015/03/25/content_281475077518617.htm . Accessed Feb 2020
Digital-Transformation-Monitor (2017) Country: Portugal “Indústria 4.0”. Technical report, European Commission. https://ec.europa.eu/growth/tools-databases/dem/monitor/sites/default/files/DTM_Ind%C3%BAstria%204.pdf . Accessed Feb 2020
Prime-Minister’s-Office of Singapore (2016) Research, innovation and enterprise 2020 plan: winning the future through science and technology. Technical report, National Research Foundation of Singapore Government. https://www.nrf.gov.sg/rie2020 . Accessed Feb 2020
Kang HS, Lee JY, Choi SS, Kim H, Park JH, Son JY, Kim BH, Noh SD (2016) Smart manufacturing past research, present findings, and future directions. Int J Precis Eng Manuf Green Technol 3:111–128
Digital-Transformation-Monitor (2017) Spain: Industria Conectada 4.0. Technical report, European Commission. https://ec.europa.eu/growth/tools-databases/dem/monitor/sites/default/files/DTM_Industria%20Connectada%20v1.pdf . Accessed Feb 2020
Foresight (2013) The Future of Manufacturing: a new era of opportunity and challenge for the UK. Technical report, The Government Office for Science, London. https://assets.publishing.service.gov.uk/government/uploads/system/uploads/attachment_data/file/255922/13-809-future-manufacturing-project-report.pdf . Accessed Feb 2020
The-White-House (2011) President Obama launches Advanced Manufacturing Partnership. Technical report, Office of the Press Secretary. https://obamawhitehouse.archives.gov/the-press-office/2011/06/24/president-obama-launches-advanced-manufacturing-partnership . Accessed Feb 2020
Banthien H (2020) Implementation of an Industry 4.0 strategy—the German Plattform Industrie 4.0. Technical report, Secretary General of the Plattform Industrie 4.0, Germany. https://ec.europa.eu/digital-single-market/en/blog/implementation-industry-40-strategy-german-plattform-industrie-40 . Accessed Feb 2020
Digital-Transformation-Monitor (2017) Italy: “Industria 4.0”. Technical report, European Commission. https://ec.europa.eu/growth/tools-databases/dem/monitor/sites/default/files/DTM_Industria4.0_IT%20v2wm.pdf . Accessed Feb 2020
What is IVI? (2017) Tokyo: Industrial Value Chain Initiative. Technical report, Industrial Value Chain Initiative. https://iv-i.org/wp/en/ . Accessed Feb 2020
Chai TY, Ding JL (2018) Smart and optimal manufacturing for process industry. Strateg Study Chin Acad Eng 20(4):51–58
(2017) legislation.gov.uk. Higher education and research act. Technical report, The National Archives. c. 29
(2020) http://www.gov.uk . Innovate UK. Technical report, Government Digital Service. https://www.gov.uk/government/organisations/innovate-uk . Accessed Feb 2020
National Institute of Standards and Technology (2020) Request for information regarding Manufacturing USA institutes and processes. Technical report, Federal Register, https://www.federalregister.gov/documents/2020/02/27/2020-03896/request-for-information-regarding-manufacturing-usa-institutes-and-processes . Accessed Feb 2020
Download references
Acknowledgements
Both authors would like to thank EPSRC (Grant EP/R001588/1) for the funding and support.
Author information
Authors and affiliations.
Department of Chemical and Process Engineering, University of Surrey, Guildford, UK
Fengwei Yang
School of Engineering, University of Warwick, Coventry, UK
You can also search for this author in PubMed Google Scholar
Corresponding author
Correspondence to Sai Gu .
Ethics declarations
Conflict of interest.
The authors declare no conflict of interest.
Additional information
Publisher's note.
Springer Nature remains neutral with regard to jurisdictional claims in published maps and institutional affiliations.
Rights and permissions
Open Access This article is licensed under a Creative Commons Attribution 4.0 International License, which permits use, sharing, adaptation, distribution and reproduction in any medium or format, as long as you give appropriate credit to the original author(s) and the source, provide a link to the Creative Commons licence, and indicate if changes were made. The images or other third party material in this article are included in the article’s Creative Commons licence, unless indicated otherwise in a credit line to the material. If material is not included in the article’s Creative Commons licence and your intended use is not permitted by statutory regulation or exceeds the permitted use, you will need to obtain permission directly from the copyright holder. To view a copy of this licence, visit http://creativecommons.org/licenses/by/4.0/ .
Reprints and permissions
About this article
Yang, F., Gu, S. Industry 4.0, a revolution that requires technology and national strategies. Complex Intell. Syst. 7 , 1311–1325 (2021). https://doi.org/10.1007/s40747-020-00267-9
Download citation
Received : 26 October 2020
Accepted : 23 December 2020
Published : 27 January 2021
Issue Date : June 2021
DOI : https://doi.org/10.1007/s40747-020-00267-9
Share this article
Anyone you share the following link with will be able to read this content:
Sorry, a shareable link is not currently available for this article.
Provided by the Springer Nature SharedIt content-sharing initiative
- Industry 4.0
- Find a journal
- Publish with us
- Track your research
Industry 4.0: Towards future industrial opportunities and challenges
Ieee account.
- Change Username/Password
- Update Address
Purchase Details
- Payment Options
- Order History
- View Purchased Documents
Profile Information
- Communications Preferences
- Profession and Education
- Technical Interests
- US & Canada: +1 800 678 4333
- Worldwide: +1 732 981 0060
- Contact & Support
- About IEEE Xplore
- Accessibility
- Terms of Use
- Nondiscrimination Policy
- Privacy & Opting Out of Cookies
A not-for-profit organization, IEEE is the world's largest technical professional organization dedicated to advancing technology for the benefit of humanity. © Copyright 2024 IEEE - All rights reserved. Use of this web site signifies your agreement to the terms and conditions.
The “dark side” of Industry 4.0: How can technology be made more sustainable?
International Journal of Operations & Production Management
ISSN : 0144-3577
Article publication date: 20 June 2023
Issue publication date: 26 April 2024
A positive outlook on the impact of Industry 4.0 (I4.0) on sustainability prevails in the literature. However, some studies have highlighted potential areas of concern that have not yet been systematically addressed. The goal of this study is to challenge the assumption of a sustainable Fourth Industrial Revolution by (1) identifying the possible unintended negative impacts of I4.0 technologies on sustainability; (2) highlighting the underlying motivations and potential actions to mitigate such impacts; and (3) developing and evaluating alternative assumptions on the impacts of I4.0 technologies on sustainability.
Design/methodology/approach
Building on a problematization approach, a systematic literature review was conducted to develop potential alternative assumptions about the negative impacts of I4.0 on sustainability. Then, a Delphi study was carried out with 43 experts from academia and practice to evaluate the alternative assumptions. Two rounds of data collection were performed until reaching the convergence or stability of the responses.
The results highlight various unintended negative effects on environmental and social aspects that challenge the literature. The reasons behind the high/low probability of occurrence, the severity of each impact in the next five years and corrective actions are also identified. Unintended negative environmental effects are less controversial than social effects and are therefore more likely to generate widely accepted theoretical propositions. Finally, the alternative hypothesis ground is partially accepted by the panel, indicating that the problematization process has effectively opened up new perspectives for analysis.
Originality/value
This study is one of the few to systematically problematize the assumptions of the I4.0 and sustainability literature, generating research propositions that reveal several avenues for future research.
- Industry 4.0
- Digitalization
- Smart manufacturing
- Sustainability
- Problematization
Dieste, M. , Orzes, G. , Culot, G. , Sartor, M. and Nassimbeni, G. (2024), "The “dark side” of Industry 4.0: How can technology be made more sustainable?", International Journal of Operations & Production Management , Vol. 44 No. 5, pp. 900-933. https://doi.org/10.1108/IJOPM-11-2022-0754
Emerald Publishing Limited
Copyright © 2023, Marcos Dieste, Guido Orzes, Giovanna Culot, Marco Sartor and Guido Nassimbeni
Published by Emerald Publishing Limited. This article is published under the Creative Commons Attribution (CC BY 4.0) licence. Anyone may reproduce, distribute, translate and create derivative works of this article (for both commercial and non-commercial purposes), subject to full attribution to the original publication and authors. The full terms of this licence may be seen at http://creativecommons.org/licences/by/4.0/legalcode
1. Introduction
In the existing literature on Industry 4.0 (I4.0) and sustainability, the prevailing assumption is that digitalization is the key to more efficient and sustainable manufacturing companies (see Beltrami et al. , 2021 ; Birkel and Müller, 2021 ; Ghobakhloo, 2020 for a review on the topic). For example, some authors suggest that new technologies such as the IoT, sensors and big data analytics can reduce the resource and energy consumption of manufacturing activities through detection and data analysis ( Bai et al. , 2020 ). Similarly, social sustainability benefits, such as better working conditions due to more ergonomic and safer tasks, can also be achieved through the adoption of human–machine interaction technologies ( Müller and Voigt, 2018 ).
In contrast, some studies have highlighted possible negative unintended effects of I4.0 technologies on environmental and social sustainability ( Bohnsack et al. , 2022 ; Dieste et al. , 2022 ; Ghobakhloo et al. , 2021 ). Scholars argue, for instance, that fully automated production could lead to higher primary resource consumption ( Stock et al. , 2018 ); that blockchain or cloud computing applied to production and supply chain management may lead to higher energy consumptions ( Singh and Bhanot, 2020 ); and that cloud technologies could cause the loss of employees' autonomy due to continuous sharing of data ( Cirillo et al. , 2021 ). However, while there is already some evidence challenging established knowledge about I4.0 and sustainability, no previous studies have systematically questioned the main assumptions of the literature by analyzing potential unintended sustainability impacts of I4.0 ( Birkel and Müller, 2021 ; Ghobakhloo et al. , 2021 ; Beltrami et al. , 2021 ).
According to MacCarthy et al. (2013) , relevant contemporary issues such as the one presented (i.e. the unintended negative impacts of I4.0 on sustainability) can be addressed through research approaches that are not gap-spotting driven, such as through a process of problematization. Therefore, this paper uses a problematization approach ( Alvesson and Sandberg, 2011 ) to formulate alternative assumptions that challenge, rather than build on and extend an established body of literature that highlights the positive impact of I4.0 technologies on sustainability performance.
Through this approach, this paper aims to formulate and evaluate alternative assumptions that can potentially develop new research propositions regarding the sustainability impacts of I4.0. More in detail, this study: (1) identifies the possible unintended negative impacts of I4.0 technologies on firms' environmental and social sustainability aspects; (2) highlights qualitative elements that support the alternative assumptions such as motivations, mechanisms behind and possible mitigation actions; and (3) develops and evaluates alternative assumptions about the unintended negative effects of I4.0 technologies on sustainability.
To achieve these aims, the six methodological principles for problematization proposed by Alvesson and Sandberg (2011) were fulfilled through a combined methodological approach. First, a comprehensive review of the literature on the negative sustainability outcomes of I4.0 technologies was conducted, which identified 12 unintended negative impacts challenging extant literature's assumptions. Second, a Delphi study involving 43 international experts was performed to evaluate the alternative assumptions by assessing the probability of occurrence in the next five years and the severity of each I4.0 unintended negative effect. Moreover, Delphi participants were asked to illustrate the mechanisms behind each negative impact and to propose mitigation actions at firm, supply chain and policy levels. A Delphi study method was selected due to the exploratory and interdisciplinary nature of the research problem. As suggested by Alvesson and Sandberg (2011) , a heterogeneous group of experts was involved in the problematization process.
The findings present a systematic overview and preliminary evaluation of the most important and controversial unintended negative impacts of I4.0 technologies on the environmental and social dimensions of sustainability. This evidence challenges the current state of knowledge in I4.0 and sustainability research and therefore contributes to the academic debate by opening a set of important avenues for future research. The results are also relevant to managers who plan and oversee the effective and sustainable implementation of I4.0 technologies. Furthermore, the corrective actions proposed can be valuable to policymakers, as some prevention and mitigation strategies are beyond the scope of companies.
2. Background
2.1 the problematization approach.
The aim of problematization is “ to come up with novel research questions through a dialectical interrogation of the domain of literature targeted for assumption challenging, instead of spotting gaps within a literature domain ”; in doing so this method encourages the creation of new theories ( Alvesson and Sandberg, 2011 , p. 252). To this end, the authors developed a set of six methodological principles (see Table 1 ) on how assumptions in existing theory can be problematized and used to generate new research propositions.
In this study, the problematization approach was used to identify and challenge the assumptions underlying the existing literature on I4.0 and sustainability. Alvesson and Sandberg's (2011) principles were applied as follows: (1) identifying the literature domain and selecting influential texts on the research topic of I4.0 and sustainability; (2) identifying the assumptions underlying this literature; (3) evaluating these assumptions by identifying preliminary evidence that suggests they are worthy of being problematized; (4) developing alternative assumptions through a systematic literature review (SLR); (5) considering assumptions related to the audience through a multi-round Delphi study; and (6) evaluating the alternative assumptions' ground. The problematization process followed throughout this article is summarized in Table 1 .
2.2 Underlying assumptions in the I4.0 and sustainability literature
The first principle of problematization involves an in-depth reading of key texts on the specific area of literature targeted. This allows for the subsequent identification of the underlying assumptions to be challenged. Some of the most relevant works in the field have suggested that I4.0 can be a significant factor in achieving improvements in sustainability performance. For instance, the seminal I4.0 report by Kagermann et al. (2013) states that smart factories will ensure that production is “simultaneously attractive and sustainable”. Stock and Seliger (2016) suggest that I4.0 offers a great opportunity to achieve economic, social and environmental sustainability. Frank et al. (2019) suggests that various I4.0 technologies can promote sustainable manufacturing by improving energy efficiency and producing less waste than traditional manufacturing.
This evidence has paved the way for an overwhelming number of articles on I4.0 and sustainability. As recommended by Alvesson and Sandberg (2011) , more recent literature was analyzed to understand whether a positive relationship between I4.0 and sustainability is still relevant. For instance, Kamble et al. (2018a) consider sustainability among the many elements of I4.0, concluding that smart technologies have the potential to reduce waste and energy consumption. Li et al. (2020) , after conducting a survey of Chinese manufacturing firms, conclude that digital technologies have a positive impact on economic and environmental performance. Bai et al. (2020) highlight that I4.0 technologies positively affect several dimensions of firms' environmental performance, such as promoting resource efficiency and reducing the materials used in production processes. Ghobakhloo (2020) indicates that I4.0 technologies such as simulation, IoT and AI can facilitate the reduction of carbon emissions. Finally, Zheng et al. (2021) argue that I4.0 offers energy efficiency opportunities to the manufacturing industry by applying optimization algorithms, modelling and simulation.
In addition, recent studies have also highlighted a set of positive implications of I4.0 on social sustainability performance. For instance, Kiel et al. (2020) suggest that fair wage assessments, human learning and employee motivation are among the social implications of I4.0. Cagliano et al. (2019) indicate that workers may benefit from more autonomy in performing tasks, resulting in more social interactions and teamwork. Occupational health and safety can be positively improved due to the substitution of heavy manual work, reducing the risk of injury (Birkel and Müller, 2021) .
There is a positive relationship between the adoption of I4.0 technologies and firms' environmental sustainability performance.
There is a positive relationship between the adoption of I4.0 technologies and firms' social sustainability performance.
2.3 Evaluating the underlying assumptions in the I4.0 and sustainability literature
The third problematization principle suggests evaluating whether the identified assumptions ( u1 , u2 ) are worth challenging. Recent reviews such as Ghobakhloo et al. (2021) and Beltrami et al. (2021) reveal that the literature is generally over-optimistic regarding the economic and socio-environmental impacts of I4.0. Bohnsack et al. (2022) explain that the introduction of digital technology can have both positive and negative unintended effects that either create additional sustainable value or destroy existing sustainable value.
According to Merton's (1936) seminal paper, unintended consequences must be viewed in relation to their intended consequences. In this study, the intended consequences are defined as the improvements in socio-environmental sustainability resulting from the implementation of I4.0, according to the underlying assumptions presented in Section 2.2 . Thus, the unintended consequences are those unwanted negative sustainability impacts of I4.0 technologies that are not considered intended outcomes ( Sugiyama et al. , 2017 ). Various studies have, in fact, already acknowledged some unintended negative effects of I4.0 on sustainability performance. Chiarini (2021) , for instance, found empirical evidence of increased energy consumption and e-waste generation and highlights the need for a deeper investigation of the environmental issues emerging from I4.0 implementation. Birkel and Müller (2021) also raise concerns about who will be responsible for increased waste and predict rising energy consumption levels due to the rapid proliferation of data centers.
Similarly, Schneider and Kokshagina (2021) recommend further investigation into I4.0 unintended negative sustainability outcomes, including consideration of social aspects. The authors discuss the replacement of workers by new digital technologies and the challenge for employees to find their own balance between on and off time. Similarly, Bai et al. (2020) , discuss the potential ethical, privacy and autonomy issues associated with data sharing in the cloud. According to the authors, the social implications of I4.0 have been understudied.
In conclusion, several key literature reviews and empirical studies have already suggested some unintended negative impacts of I4.0 on both environmental and social dimensions of sustainability. This evidence justifies the objective of this study, that is to question the underlying assumption of a sustainable Fourth Industrial Revolution.
3. Methodology
3.1 developing alternative assumptions through a slr.
To address the fourth problematization principle – i.e. developing alternative assumptions – a SLR of the negative impacts of I4.0 was conducted. Durach et al. ’s (2017) six sequential steps for SLRs were adopted: (1) define the research question, (2) determine the required characteristics of primary studies, (3) retrieve a sample of potentially relevant literature (baseline sample), (4) select pertinent literature (synthesis sample), (5) synthesize the literature and (6) report the results.
(“Industry 4.0” OR “Industrie 4.0” OR “fourth industrial revolution” OR “4th industrial revolution” OR “Digital transformation” OR “Industrial automation” OR “Smart manufacturing” OR “Smart production” OR “Smart factory” OR “Smart industr*” OR “Cyber physical system*” OR “Cyber physical production system*” OR “Intelligent manufacturing” OR “Internet of things” OR “Digital twin” OR “Software-defined manufacturing”) AND (“Sustainab*” OR “Green” OR “Environmental Performance” OR “Social Performance”).
A total of 6,140 contributions resulted from the search, which made up the baseline sample.
Fourth, the inclusion/exclusion criteria were gradually applied. Papers with non-coherent abstracts were removed – that is, those articles that are not primarily focused on I4.0 and environmental and social sustainability or that discuss I4.0 and sustainability independently – leaving 269 articles in the dataset. A total of 117 publications discussing the relationship between I4.0 and sustainability were identified through full-text analysis. Consistent with other literature reviews, subsequent cross-referencing was conducted for further relevant publications ( Seuring and Gold, 2012 ). Finally, 39 papers were selected that focused on unintended negative sustainability impacts of I4.0.
Fifth, descriptive and content analyses were conducted on the data set. The articles were classified according to the following criteria: authors, year of publication, research topic, methodology, sample used, technologies analyzed and unintended negative impacts supported. The content analysis methodology was carried out by identifying the specific findings displaying negative effects of I4.0 on sustainability performance. For each paper, specific findings regarding unintended negative sustainability impacts on both environmental and social dimensions were categorized according to the indicators included in the Global Reporting Initiative (2018) framework.
Sixth, the results were then grouped into 12 statements, 5 for environmental [E1-E5] and 7 for social [S1-S7] unintended negative impacts, which are reported in Section 4.1 with supporting arguments. A descriptive overview of the results is provided in the Appendix .
3.2 Relating assumptions to audience through a Delphi study
As mentioned in the fifth principle , the problematization methodology suggests considering assumptions in relation to the audience. Alvesson and Sandberg (2011) point out that the “audience” is usually not a uniform group, and therefore it is important to combine academic and practical expertise to understand their different views. A Delphi study approach was considered the most appropriate research method for this purpose due to the incompleteness of the available knowledge and the exploratory, interdisciplinary and forward-looking nature of the research ( Linstone and Turoff, 1975 ). As Delphi studies are based on gathering expert opinions in a structured manner, they are very appropriate for structuring group communication processes, allowing individuals to deal with complex and interdisciplinary problems ( Okoli and Pawlowski, 2004 ).Therefore, this Delphi study aims to provide a systematic assessment (probability/severity) to explore whether the interested audiences agree or disagree with the unintended negative impacts presented, thus evaluating alternative assumptions. It also aims to provide possible motivations, additional details and mitigation actions. In doing so, this study systematically collects qualitative evidence from experts that goes beyond the mostly anecdotal knowledge present in the literature.
3.2.1 Selection of the expert panel
Following the methodological suggestions above, the research sample included experts from both academia and practice, working in different functions, with different nationalities and years of experience ( Okoli and Pawlowski, 2004 ). A rigorous selection of the panel of expert members was carried out ( Landeta, 2006 ). A panel size of at least 30 participants with heterogeneous backgrounds was sought, as suggested by previous studies ( Kembro et al. , 2017 ).
Consistent with prior research, selection criteria were defined to ensure that the experts were knowledgeable about I4.0 and sustainability topics. Initial sampling was carried out by contacting academics who had authored publications related to the topic and leveraging networks of personal contacts ( Culot et al. , 2020 ). Nevertheless, most of the experts contacted were industry professionals working in the manufacturing sector such as digital transformation specialists, general managers, operations managers and supply chain managers. Management consultants working for manufacturing companies were also considered. This was done as it was intended to reach a wide range of interest groups. Further potential participants were scouted through LinkedIn social network and alumni databases.
An initial list of 150 participants was established, 63 of whom accepted to participate in the Delphi study. Moreover, to ensure rigor during the participant selection process, the experts who agreed to participate were asked to self-rate their perceived level of knowledge of the topic by answering three Likert-type scale questions (1: Very low, 5: Very high) regarding I4.0, sustainability and the manufacturing sector ( Landeta, 2006 ). 20 respondents were excluded from the sample due to low overall scores or incomplete responses. The final panel consists of 43 experts familiar (median = 4) with I4.0, sustainability and manufacturing: Table 2 illustrates its composition. 5 participants dropped out during the second round.
3.2.2 Data collection and analysis
During a 10-month time span, reiterated rounds of data collection were carried out, as suggested for Delphi studies, to obtain convergence or stability ( Linstone and Turoff, 1975 ; von der Gracht, 2012 ). The first round began in early July 2021, and the feedback was collected over five months. An invitation letter and the link to a web-survey commercial software containing the questions for the first round were sent. The Delphi questionnaire started with a brief background to the study and defined the scope and objectives of the project; it then moved on to various questions aimed at assessing participants' suitability for the panel. In addition, various demographic and qualification questions were asked.
Next, respondents found a series of rating-scale and open-ended questions written in a comprehensible writing style to avoid ambiguous statements. Panel experts were asked to assess the importance of each of the preliminary I4.0 negative sustainability impacts (see Section 4.1 ) in terms of probability of occurrence (over the next five years) and severity of the problem (level of impact). Five-point Likert-type scale questions were used for this purpose (1: Very low, 5: Very high). Respondents were requested to provide arguments for high and low probability/severity and illustrate the mechanisms behind each negative effect. Moreover, experts were asked to propose corrective actions at firm, supply chain and policy levels and to comment or provide additional discussion elements.
Once data had been collected, the median values for the probability and severity of each negative effect were calculated, and the level of consensus was determined by the interquartile range (IQR) ( von der Gracht, 2012 ). Qualitative data were approached through a content analysis, resulting in a list of arguments supporting high and low probability/severity for each I4.0 negative impact and various relevant corrective actions at the three levels proposed.
After the data and qualitative responses of the first round were analyzed, a s econd round was performed. Each participant received an online form including the statistics (median and IQR), arguments and his/her previous assessment during the first round ( Okoli and Pawlowski, 2004 ; Seuring et al. , 2022 ). Moreover, the second-round questionnaire contained the reorganized comments and mitigation actions provided by the panel for each I4.0 negative effect. The questionnaire form thus allowed participants to either modify their answers or maintain their initial assessments and provide additional remarks ( Linstone and Turoff, 1975 ). The second round of data collection started in mid-January 2022 and lasted 3 months.
Finally, the research team performed data analysis consistently with the first round, enabling a comparison between the two rounds in terms of consistency of responses (i.e. stability) and calculating the Spearman's rank-order correlation coefficient (ρ) ( von der Gracht, 2012 ). After the second round, the assessments of the 12 Likert-type items (E1-E5 and S1-S7) reached either consensus (IQR ≤1) or stability ( ρ ≥ 0.75), thus making further iterations of the questionnaire unnecessary ( Culot et al. , 2020 ). This process resulted in some arguments with high consensus accepting or rejecting alternative assumptions (see Section 4.1 ), and others with contrasting results and low consensus.
3.3 Reliability and validity
Both the SLR and Delphi methods were tested for reliability and validity using different approaches. Regarding the SLR process, two researchers independently reviewed the evidence from the literature to improve the reliability of the unintended negative impacts of I4.0 found. Both researchers followed a systematic approach to ensure the objectivity of the literature review ( Durach et al. , 2017 ). Moreover, several rounds of discussion were held within the research group to discuss disagreements during the coding process and to refine the final list of unintended negative I4.0 sustainability impacts identified in the literature.
For the Delphi study, before the questionnaires were sent to the panel of experts, two pre-tests were conducted with four senior experts (two academics and two practitioners) to ensure consistency and comprehensiveness for each round ( von der Gracht, 2012 ). The tests were developed in the following manner: (1) informing the four experts about the research aims, (2) sending out the Delphi questionnaire and (3) interviewing the four experts about the clarity and the appropriateness of the questions.
During the pre-tests, various comments were extracted and discussed. The insights obtained from this process were employed to correct, rectify and validate the questionnaire. The final version of the Delphi questionnaire was reviewed one last time by the four experts involved in the pre-tests and by the research group. Subsequently, data collection was conducted in the same manner to pursue internal validity of the findings. Pre-test participants were not part of the final panel of experts.
Finally, to ensure the validity of the findings, both the first- and second-round results were presented at important operations management conferences to collect feedback and comments.
This section is divided into three subsections. Section 4.1 presents the alternative assumption ground based on the SLR results ( fourth principle ). In Section 4.2 , the evidence supporting the alternative assumptions is related to the audience through a Delphi study ( fifth principle ). Finally, Section 4.3 discusses some strategies proposed by the Delphi participants to mitigate the identified negative sustainability impacts of I4.0 technologies.
4.1 Developing alternative assumptions – SLR results
Table 3 summarizes the 12 potential unintended negative effects of I4.0 found in the literature on both environmental [E1-E5] and social [S1-S7] dimensions (literature review articles were not included in the supporting references to avoid redundancies).
4.1.1 Environmental sustainability
Previous research indicates that I4.0 may have various unintended negative impacts on the environmental performance of manufacturing companies. First, connectivity and data processing following the adoption of I4.0 technologies in production and supply chain management (e.g. big data analytics, AI, cloud computing, autonomous robots and blockchain) lead to higher levels of energy consumption [E1]. Müller and Voigt (2018) and Stock et al. (2018) , conclude that ecological challenges may appear due to the increased energy used by data generation and interchange. Singh and Bhanot (2020) suggest that many devices communicating with each other generate large amounts of data which can overwhelm computing infrastructures, leading to higher energy consumption. Similarly, Biswas et al. (2022) capture the trade-off between traceability and sustainability and indicate that blockchain is characterized by high energy consumption.
Second, I4.0 technologies' adoption (e.g. robots, CPS, IoT and additive manufacturing devices) imply the obsolescence and replacement of previous devices, increasing material waste, which, in some cases, is hazardous waste [E2]. Müller et al. (2018) point out that the adoption of I4.0 often involves the need to replace entire production processes. In fact, it is difficult and complex to upgrade individual machines with different degrees of automation and at different life stages. Ghobakhloo and Fathi (2019) highlight, the high cost of dismantling obsolete devices. Birkel et al. (2019) and Di Carlo et al. (2021) also indicate that the time and cost required to replace obsolete equipment may be unsustainable, as most of the old machinery must be discarded and ends up in landfill.
Third, wireless technologies include components and consumables (e.g. batteries, antennas) that raise the production of waste of electrical and electronic equipment (WEEE) [E3]. Integrated production infrastructures involve the use of devices, such as sensors and actuators, which can lead to an increase in electronic waste ( Birkel et al. , 2019 ). In fact, the recent increase in sales of electrical and electronic equipment is causing worldwide concern about the management of WEEE, according to Garrido-Hidalgo et al. (2020) . Moreover, Chiarini (2021) outlines that managers are greatly concerned about the vast quantities of WEEE produced and its treatment.
Fourth, hardware needed for I4.0 implementation (e.g. sensors, chips, connectivity infrastructure) requires higher consumption of natural resources (e.g. metals, water, energy) than traditional manufacturing technologies [E4]. According to Stock et al. (2018) and Birkel et al. (2019) , technologies in I4.0 require large amounts of critical raw materials used for RFID, sensors, displays, semiconductors and micro-energy harvesting. Experts believe that rare natural resources are increasingly needed in manufacturing activities and products ( Culot et al. , 2020 ). Chiarini (2021) agrees that there has been higher consumption of rare metals and other natural resources due to the implementation of I4.0 technologies.
Fifth, several studies point out that additive manufacturing (AM) particularly leads to higher energy consumption than traditional manufacturing processes [E5]. According to Yoon et al. (2014) , the specific energy consumption of AM processes is estimated to be higher than that of conventional processes. Similarly, Ford and Despeisse (2016) argue that while AM is more energy-intensive per unit produced, it offers higher levels of customization and less material use. Stock et al. (2018) indicate that, due to the frequent use of laser technologies to sinter the material, AM processes are still energy-inefficient.
In summary, the literature has already identified some preliminary evidence of unintended negative impacts of I4.0 technologies on the environmental performance of manufacturing firms. This alternative rationale challenges the existing assumption in the literature that I4.0 is the key to achieving environmental performance outcomes (i.e. u1 ).
4.1.2 Social sustainability
Some unintended negative social implications of I4.0 have also been highlighted by the literature. First, since its inception, one of the most concerning challenges of I4.0 technologies is that they have an overall negative impact on employment [S1] ( Kamble et al. , 2018b ). According to Grigore et al. (2021) , I4.0 displaces some of the low-skilled workers who perform simple and repetitive tasks. However, this loss of jobs should be balanced by the increased demand for more skilled positions. Authors such as Müller et al. (2018) and Birkel et al. (2019) state that the existing literature cannot provide a unified perspective on whether I4.0 will cause an increase or a decrease in the total number of employees in manufacturing.
Second, some studies suggest that I4.0 technologies (e.g. CPS, IoT, cloud computing) cause a loss of employees' privacy and personal autonomy [S2]. Sugiyama et al. (2017) remark that this is one of the unintended side effects of the digital transition. More recently, Bai et al. (2020) suggest that cloud technologies and big data have a positive impact on social sustainability. However, the authors highlight potential privacy and personal autonomy issues related to sharing data in the cloud. Cirillo et al. (2021) conclude that I4.0 reduces employee autonomy and increases management control.
Third, the connectivity facilitated by I4.0 technologies could lead to an unhealthy work – life balance, causing stress or mental health problems [S3]. Coldwell (2019) suggests that the digital era allows employees to continue working even when away from the office. Bad practices associated with remote work and working outside of business hours can lead to depression and mental illness. Grigore et al. (2021) claim that digital technologies facilitate inclusive and flexible working practices. However, these same technologies also raise concerns regarding surveillance, exploitative employment contracts and data privacy. As reported by Schneider and Kokshagina (2021) , the digital workplace can be more exhausting, requiring employees to balance work time and off-time. In addition, the social and emotional skills required and the difficulties in managing remote work and evaluating individual contributions can lead to additional stress. This topic has gained relevance as the COVID-19 pandemic inevitably prompted new ways of working that may become an integral part of the post-pandemic reality ( Narayanamurthy and Tortorella, 2021 ).
Fourth, some recent studies suggest that firms adopting I4.0 technologies will relocate production and related activities, such as research and development (R&D) and logistics, to developed countries [S4]. The impact of this change is ambivalent, being positive for the countries where manufacturing will be relocated but negative for those abandoned. Ancarani et al. (2019) and Dachs et al. (2019) show a positive relationship between the adoption of I4.0 and firms' backshoring propensity. This occurs mainly due to the implications of I4.0 on the cost and quality of products. Moreover, Pegoraro et al. (2022) suggest that technology adoption supports manufacturing reallocation strategies. Barbieri et al. (2022) remark the importance of I4.0 policies to attract innovative I4.0 firms back to their country of origin.
Fifth, some scholars acknowledge that autonomous and collaborative robots can cause health and safety issues when interacting with employees [S5]. One of the main concerns about collaborative robotics is related to safety issues such as mechanical, electrical and thermal hazards ( Leso et al. , 2018 ). Li et al. (2019) affirm that accidental collisions can happen in the process of human-robot interaction in a limited and shared physical space. Furthermore, according to Dalmarco et al. (2019) , the integration of collaborative robotics in the shop floor can generate risks in interactions with workers.
Sixth, virtual (VR) and augmented reality (AR) produce headache, dizziness and other symptoms [S6]. Studies such as Tsai and Huang (2018) report that most smart glasses' users complained of dizziness. Wang et al. (2019) state that several users noticed visual fatigue after performing maintenance activities wearing Google Glasses. Rodriguez et al. (2021) show that it took time for users' eyes to adapt to smart glasses, that they were uncomfortable, and that operators experienced headaches after wearing them for a while.
Seventh, the utilization of AM in production environments causes harsh skin reactions, eye irritation and allergies to the operators involved [S7]. Ford and Despeisse (2016) conclude that AM may have several implications for social sustainability, including health and safety. Väisänen et al. (2019) measured the concentrations of gaseous and particulate contaminants originating from AM processes in an occupational environment and found that AM operations emitted potentially harmful contaminants. Furthermore, Chan et al. (2020) findings suggest that emissions increase when multiple AM devices operate simultaneously, and the authors recommend adherence to good safety and hygiene practices when this technology is deployed.
In summary, preliminary evidence from the literature challenges the current assumption that smart technologies lead to better social performance outcomes (i.e. u2 ). I4.0 may indeed entail various unitented negative impacts on social sustainability. This alternative assumption ground, which suggests negative unintended impacts of I4.0 on socio-environmental performance, needs to be evaluated by the relevant audiences. To this end, a Delphi study was conducted (see Sections 4.2 and 4.3 ).
4.2 Relating assumptions to audience – Delphi study results
The analysis of the Delphi statements is outlined in Table 4 . The median values and IQR for both “probability” ( p ) and “severity” (s) assessments are shown for the two rounds. In addition, the stability between rounds (Spearman's ρ) is indicated for the whole panel. Based on the results of the second round, several negative effects of I4.0 in the uniform opinion of experts (consensus IQR≤1) show a medium-high probability of occurring and/or severity. These effects concern aspects of both environmental sustainability [E1, E2, E3, E4] and social sustainability [S2, S3, S6].
Furthermore, the findings show a growing convergence of responses caused by the iteration of the questionnaire. After the first round, 4 out of 24 items (12 potential negative impacts assessed in terms of “probability” and “severity”) reached consensus. After the second round, consensus was reached on 15 out of 24 items. Spearman's ρ generally shows high stability in values related to the probability of occurrence and severity.
The median of the second-round probability and severity scores for the entire panel of experts;
The level of agreement reached by the panel;
Arguments for high and low probability and severity that emerged from the content analysis of the experts' comments.
Regarding the unintended negative environmental impacts ( Table 5 ), the experts suggest that wireless technologies will increase the generation of electrical and electronic equipment waste [E3]. This problem received the highest ratings in terms of probability of occurrence and severity (Me p = Me s = 4; IQR p = IQR s = 1) due to the use of electronic components and consumables with short lifespans. Experts also agreed that I4.0 leads to a higher consumption of natural resources than traditional manufacturing technologies, due to hardware needs [E4] (Me p = 4, Me s = 3; IQR p = IQR s = 1). In particular, the advancement of I4.0 technologies might cause an increase in the consumption of rare materials.
Furthermore, the shorter life cycle of new devices and the lack of compatibility and limited refurbishment options of old equipment are among the main reasons for the increase in waste due to the obsolescence of old equipment [E2]. The participants considered that this is a problem with a high probability of occurrence, but less agreement was reached concerning the severity, which is medium (Me p = 4, Me s = 3; IQR p = 1 , IQR s = 2). The lower severity and consensus values are mainly due to the “retrofitting opportunities available”, the new recycling technologies and available recyclable materials.
Besides, panel experts considered that the higher levels of energy consumption produced by connectivity and data processing constitute a problem of medium severity [E1] (Me s = 3; IQR s = 1). However, they achieved less consensus when evaluating the probability of occurrence (Me p = 3; IQR p = 2). Relevant arguments for both high and low probability/severity were provided. For instance, “data centers and the cooling needed” require a significant amount of energy; in contrast, I4.0 technologies “should lead to greater efficiency of the entire process”.
Lastly, respondents stated with a high consensus that there is low probability that AM leads to higher energy consumption than traditional manufacturing processes [E5]. Panel experts considered that this issue is less likely to happen in the future due to the limited implementation of AM. The experts' low level of concern about this problem was because “current AM applications are already leading to energy savings” (Me p = Me s = 2; IQR p = IQR s = 1).
As regards the unintended negative social impacts of I4.0, Table 6 shows that technologies such as CPS, IoT and cloud computing could cause loss of privacy and autonomy among employees [S2]. Respondents recognized “greater traceability” as a current problem, this may have a greater impact on developing countries (Me p = Me s = 3; IQR p = 1, IQR s = 2). Furthermore, I4.0 technologies may lead to an unhealthy work – life balance, stress and mental health problems [S3]. Remote work generally provides flexibility and support for employees but may cause monotony and “fear of being closely monitored”. This has already been observed in some sectors (Me p = Me s = 3; IQR p = 1, IQR s = 2). Regarding workplace safety and ergonomics, the Delphi participants considered that VR and AR caused problems such as headache and dizziness [S6]. The experts warned that VR and AR technologies must be used only in suitable workstations and for short periods (Me p = Me s = 3; IQR p = IQR s = 1).
Participants did not support the negative implications of autonomous robots (Me p = Me s = 2; IQR p = 1, IQR s = 2) [S5] and AM on the safety of workers (Me p = 2, Me s = 3; IQR p = IQR s = 1) [S7]. Reasons provided included the fact that “robots are now much safer” and that laws and certifications address this issue. For AM, limited production usage and personal protection tools made this issue less likely for experts.
Finally, the experts did not reach a high level of consensus on job replacement, with some acknowledging that layoffs will be offset by more efficient companies growing and hiring (Me p = Me s = 3; IQR p = 1.75, IQR s = 2) [S1]. Similarly, regarding the relocation of production and related activities to developed countries [S4], participants argued that “I4.0 will make the economies of developed countries competitive again” but at the same time “allows regionalization of supply chains” (Me p = Me s = 3; IQR p = IQR s = 2).
4.3 Potential mitigation actions
The experts highlighted various corrective actions to counteract the adverse sustainability effects of I4.0. These actions were classified after the first round of the Delphi study into firm-level actions, supply chain-level actions and policy interventions. In the second round, the experts were asked to confirm the mitigation actions, comment on them and add more possible interventions. Table 7 shows the full list of mitigation actions proposed.
4.3.1 Environmental sustainability
The respondents suggested some mitigation actions at the company level [F1-F24]. For instance, participants proposed accurate monitoring and forecasting of energy and waste through key performance indicators (KPIs) [e.g. F2, F8, F9], offsetting additional resource consumption and waste by using renewable resources [F1, F16, F22], preventing issues from the design phase by using environmentally friendly components and materials [F13, F21, F23], encouraging gradual implementations or retrofitting/modular solutions [F3, F10, F12] and reusing/implementing durable technologies [F11, F14, F17].
Regarding the supply chain level [C1-C10], collaborating with environmentally friendly suppliers and service providers [e.g. C1, C3, C6], establishing recycling and reusing initiatives [e.g. C4, C5, C8] and sharing data to avoid hardware duplicities and thus save energy throughout the supply chain [C2] are the most relevant mitigation actions.
Furthermore, the panel experts called for policy interventions [P1-P10] such as encouraging “green” initiatives through national/international economic incentives, laws and standards [e.g. P3, P5, P8], supporting research [P7, P10] and requiring additional energy consumption to be balanced with renewable energy resources [P2].
4.3.2 Social sustainability
At company level [F25-F49], firms may implement preventive actions such as promoting cybersecurity and data privacy [e.g. F28, F29, F30], monitoring and preserving workers' wellbeing [e.g. F31, F32, F40], and using safety systems [e.g. F36, F46, F48]. In addition, the experts suggested redesigning workplaces [e.g. F38, F41, F49], implementing technology gradually [F34, F45] and developing training plans in I4.0 [F25, F37] to avoid some of the social concerns produced by I4.0 technologies.
Throughout the supply chain [C11-C19], cooperation is essential to ensure that I4.0 equipment meets all safety recommendations [C17, C18, C19], support and coordinate I4.0 adoption at partner companies in developing countries [C14, C15, C16] and to retrain and relocate employees [C11]. In addition, outsourcing services such as data management and monitoring of the welfare of workers [C12, C13] are considered appropriate solutions.
Finally, policy interventions acquire special relevance for social sustainability [P11-P24]. Policymakers could update laws and standards to protect data privacy and integrate physical and psychological risks derived from I4.0 technologies [e.g. P13, P15, P22]. Moreover, institutions should support I4.0-focused training and research [P11, P12, P24] and foster collaboration between firms and developing economies [P17, P18].
5. Discussion
I4.0 technologies may have unintended negative impacts on firms' environmental sustainability performance.
I4.0 technologies may have unintended negative impacts on firms' social sustainability performance.
On the contrary, some of the results of this research are indeed consistent with the underlying I4.0-sustainability assumptions ( u1 , u2 ). For example, the increase in energy consumption associated with the implementation of AM ( Huang et al. , 2013 ) was judged unlikely by experts [E5]. Studies such as Dalmarco et al. (2019) point to safety problems as one of the main restrictions of collaborative robotics [S5]. Nonetheless, the Delphi participants considered that, robots are much safer than they used to be and improve social sustainability by assisting workers at unsafe workplaces. Chan et al. (2020) suggest that emissions of particulates and volatile organic compounds (VOCs) increase when multiple 3D printers are running simultaneously, posing a risk to operators [S7]. However, due to the limited use of AM and the safety measures that have been put in place, experts are minimizing the unintended effects.
In general, greater consensus was found for the negative impacts on environmental aspects. The social drawbacks of I4.0 in terms of potential job losses due to automation [S1] are indeed still very controversial due to the low consensus on both probability and severity, and the high stability reached in the second round. Studies such as Birkel and Müller (2021) acknowledge this dilemma between job creation and loss. Likewise, low agreement levels and high stability were obtained regarding the possible reshoring phenomenon produced by I4.0, which could increase the gap between developed and developing countries [S4]. These results agree with the findings of Barbieri et al. (2022) .
Figure 1 illustrates the results of this research from a theoretical perspective to evaluate what evidence supports or rejects the alternative assumption ground. The upper part of the figure shows the evidence supporting the alternative assumptions ( a1 , a2 ) with medium-high probability and/or severity (probability x severity ≥ 9). While the evidence with the greatest consensus is shown in the right part of the figure (IQR p x IQR s = 1). The results highlight, with high consensus on both probability and severity, several negative unintended consequences for both environmental [E3, E4] and social [S6] aspects (upper right quadrant of figure). These consequences challenge the current assumptions in the literature ( u1 , u2 ). On the other hand, some results support with high consensus the underlying hypotheses, as some unintended impacts have low probability and severity scores [E5, S7], therefore no additional perspectives are needed (right lower quadrant of the figure).
The results related to specific technologies that achieved low consensus (IQR p x IQR s > 1) but high stability during the Delphi rounds can be considered controversial. These findings are indicators of the success of the problematization approach and may stimulate the audience to take the challenging assumptions ( a1 , a2 ) seriously, generating interest and opening new research directions (see upper left quadrant of Figure 1 ) ( Alvesson and Sandberg, 2011 ). Although the panel does not reach a high consensus on the probability of occurrence of connectivity and computing energy consumption [E1] and the severity of obsolescence [E2], the unintended negative impact of I4.0 on the environmental sustainability performance is partially supported by the expert panel and further research may be needed to confirm a1 . On the other hand, the unintended impacts of I4.0 on social performance are more controversial due to the lower consensus reached and the contrasting evidence supporting and rejecting a2 . Therefore, further research is needed to confirm the negative effects on privacy and personal autonomy [S2] and work – life balance [S3]. These partially support a2 , but there was little consensus on the severity ratings (IQR p x IQR s = 2). Impacts on employment [S1] and backshoring [S4] implications are partially agreed but an even lower level of consensus was reached (IQR p x IQR s > 2) and therefore require further investigation.
The safety problems derived from the implementation of autonomous robots [S5] were considered of low importance (probability x severity = 4) and reached low consensus (IQR p x IQR s = 2). There is limited room for new perspectives, further research may confirm the underlying assumption regarding the social benefits of I4.0 ( u2 ).
There are actions that mitigate the unintended negative impacts of I4.0 technologies on firms' environmental sustainability performance.
There are actions that mitigate the unintended negative impacts of I4.0 technologies on firms' social sustainability performance.
Some of the proposed actions may help prevent or mitigate some unintended negative impacts, but at the same time may unintentionally conflict with other sustainability objectives. For instance, environmental actions in the supply chain, such as “developing the circular economy and recycling”, were suggested to mitigate the material waste generated by I4.0. Lopes de Sousa Jabbour et al. (2018) suggest that I4.0 may help develop a path towards the circular economy and closed-loop supply chains, reducing material waste. While I4.0 can facilitate the circular economy through better traceability of waste, it can also lead to more data processing with a consequent increase in energy consumption [E1] (see Table 5 ). The findings also indicate that the use of sensors and traceability devices could increase the generation of waste [E3], thus limiting the benefits of the circular economy. Firms need to be cautious even when implementing I4.0 for sustainability purposes, understanding the benefits but also the unintended negative impacts.
Regarding proposed policy interventions, the respondents primarily recommended incentivizing “green” behaviors through tax reductions, providing financing and developing new targeted frameworks and roadmaps coupling digital transformation and sustainability. Moreover, respondents recommended more regulatory actions addressing privacy and data security issues. Besides, Bai et al. (2020) suggest that sustainability trade-offs may not only exist across technology and sustainability dimensions, but also across industries, a result which was also observed in this study. For instance, if regulators decide to support industry investments in autonomous and collaborative robots to enhance workers' safety [S5] – increasing social sustainability – the resulting unintended impact may be a higher level of obsolescence and electronic waste in the manufacturing industry [E2], thus decreasing environmental sustainability. Currently, governments are supporting the I4.0 transition with national plans to promote competitiveness ( Chiarini, 2021 ). Respondents suggest that policy incentives to obtain new technology increase the rate of replacement of technology, leading to increased waste and resource use [E2, E3, E4]. Experts recommended that governments should be aware of the unintended negative sustainability effects of I4.0 technologies and deploy I4.0-focused actions to prevent them before they occur. In this sense, panel experts suggested further governmental actions coupling I4.0 and sustainability through standards, laws, training and research.
6. Conclusions, limitations and future research directions
Previous studies such as Bohnsack et al. (2022) , Beltrami et al. (2021) and Ghobakhloo et al. (2021) suggest that the positive implications of I4.0 for firms do not automatically translate into positive effects for the environment or society, which raises the need to challenge the current literature on I4.0 and sustainability.
This study makes several contributions to theory, practice and policy. The research findings accept and reject some of the arguments underlying the existing literature ( u1 , u2 ). Thus, perfectly accomplishing the principles and objectives of problematization and generating further interest among the targeted audiences ( Alvesson and Sandberg, 2011 ). A high degree of consensus has been reached on the existence of unintended environmental impacts. Social unintended consequences of I4.0 adoption remain instead controversial.
Quantify the unintended negative impacts that are likely to occur, are severe, and have a high level of consensus [E3, E4, S6] thus going beyond general statements which lack quantitative foundations. Case study research used in conjunction with survey-based research can be useful to confirm the alternative assumption ground ( a1 , a2 ).
Resolve controversial assumptions about unintended negative environmental [E1, E2] and social impacts [S1, S2, S3, S4, S5]. Survey studies may be useful to definitively support or reject alternative assumptions ( a1 , a2 ).
Test the effectiveness of the proposed actions ( a1.1 and a2.1 ) to prevent or mitigate the negative impacts of I4.0. Interview-based multiple case studies might be effective.
This study can be relevant for managers who plan and oversee the effective and sustainable implementation of I4.0 technologies. Practitioners can become more aware of the negative sustainability implications of I4.0 and may adopt the specific mitigation actions proposed by experts at the company and supply chain levels. Based on the Delphi results, some severe negative socio-environmental unintended implications are likely to occur in the coming years; therefore, I4.0 practitioners can prevent them before they become apparent.
In light of the results, policy makers can promote supply chain collaboration and international cooperation to achieve sustainability goals. The policy interventions presented are especially relevant in the context of “Industry 5.0”. This novel concept, which is being supported by the European Commission, complements the existing I4.0, providing a vision of industry that aims beyond efficiency and productivity and strengthens industry's role in and contribution to society. The policymaking actions suggested by the panel of experts may help to shape this approach by transitioning to a “sustainable, human-centric and resilient industry” ( European Commission, 2022 ) rather than the technology-oriented vision of I4.0.
This research presented a two-round Delphi study with a large and heterogeneous sample of professionals. This methodological approach nevertheless has some limitations that need to be acknowledged. First, some studies recommend three rounds of data collection for Delphi studies. In this study, two rounds were sufficient to achieve adequate levels of agreement (IQR) or stability (Spearman's ρ). This approach ensured that participant fatigue was minimized, which in turn facilitated a higher response rate and validity of responses ( Mitchell, 1991 ). However, it is possible that a third round might have revealed additional insights. Second, respondents' perceptions may be influenced by factors such as the level and scope of digitalization, maturity, and sector of the companies in which they work. Nonetheless, one of the goals of problematization is to obtain contrasting arguments to generate interest on the topic.
To conclude, during the two rounds of this Delphi study, experts – especially management consultants and practitioners – showed great interest in the research topic, confirming the success of the problematization approach. According to the general opinion of the panel, there is indeed a “dark side” to I4.0 that needs to be mitigated with specific actions. The research findings suggest that collaboration between academia, practice and institutions is crucial to address these issues in a timely manner.
Evaluation of the alternative assumption ground
Research process based on the problematization approach
Source(s): Authors’ elaboration
Alvesson , M. and Sandberg , J. ( 2011 ), “ Generating research questions through problematization ”, Academy of Management Review , Vol. 36 , pp. 247 - 271 .
Ancarani , A. , Di Mauro , C. and Mascali , F. ( 2019 ), “ Backshoring strategy and the adoption of industry 4.0: evidence from Europe ”, Journal of World Business , Vol. 54 , pp. 360 - 371 .
Bai , C. , Dallasega , P. , Orzes , G. and Sarkis , J. ( 2020 ), “ Industry 4.0 technologies assessment: a sustainability perspective ”, International Journal of Production Economics , Vol. 229 , 107776 .
Barbieri , P. , Boffelli , A. , Elia , S. , Fratocchi , L. and Kalchschmidt , M. ( 2022 ), “ How does industry 4.0 affect international exposure? The interplay between firm innovation and home-country policies in post-offshoring relocation decisions ”, International Business Review , Vol. 31 No. 4 , 101992 .
Beltrami , M. , Orzes , G. , Sarkis , J. and Sartor , M. ( 2021 ), “ Industry 4.0 and sustainability: towards conceptualization and theory ”, Journal of Cleaner Production , Vol. 312 , 127733 .
Birkel , H. and Müller , J.M. ( 2021 ), “ Potentials of industry 4.0 for supply chain management within the triple bottom line of sustainability – a systematic literature review ”, Journal of Cleaner Production , Vol. 289 , 125612 .
Birkel , H.S. , Veile , J.W. , Müller , J.M. , Hartmann , E. and Voigt , K.-I. ( 2019 ), “ Development of a risk framework for Industry 4.0 in the context of sustainability for established manufacturers ”, Sustainability , Vol. 11 , 384 .
Biswas , D. , Jalali , H. , Ansaripoor , A.H. and De Giovanni , P. ( 2022 ), “ Traceability vs Sustainability in supply chains: the implications of blockchain ”, European Journal of Operational Research , Vol. 305 No. 1 , pp. 128 - 147 .
Bohnsack , R. , Bidmon , C.M. and Pinkse , J. ( 2022 ), “ Sustainability in the digital age: Intended and unintended consequences of digital technologies for sustainable development ”, Business Strategy and the Environment , Kagermann , Frankfurt , Vol. 31 , pp. 599 - 602 .
Cagliano , R. , Canterino , F. , Longoni , F. and Bartezzaghi , E. ( 2019 ), “ The interplay between smart manufacturing technologies and work organization ”, International Journal of Operations and Production Management , Vol. 39 , pp. 913 - 934 .
Chan , F.L. , Hon , C.-Y. , Tarlo , S.M. , Rajaram , N. and House , R. ( 2020 ), “ Emissions and health risks from the use of 3D printers in an occupational setting ”, Journal of Toxicology and Environmental Health, Part A , Vol. 83 , pp. 279 - 287 .
Chiarini , A. ( 2021 ), “ Industry 4.0 technologies in the manufacturing sector: are we sure they are all relevant for environmental performance? ”, Business Strategy and the Environment , Vol. 30 , pp. 3194 - 3207 .
Cirillo , V. , Rinaldini , M. , Staccioli , J. and Virgillito , M.E. ( 2021 ), “ Technology vs workers: the case of Italy's industry 4.0 factories ”, Structural Change and Economic Dynamics , Vol. 56 , pp. 166 - 183 .
Coldwell , D.A.L. ( 2019 ), “ Negative influences of the 4th industrial revolution on the workplace: towards a theoretical model of entropic citizen behavior in toxic organizations ”, International Journal Environmental Research and Public Health , Vol. 16 No. 15 , p. 2670 .
Culot , G. , Orzes , G. , Sartor , M. and Nassimbeni , G. ( 2020 ), “ The future of manufacturing: a Delphi-based scenario analysis on industry 4.0 ”, Technological Forecasting and Social Change , Vol. 157 , 120092 .
Dachs , B. , Kinkel , S. and Jäger , A. ( 2019 ), “ Bringing it all back home? Backshoring of manufacturing activities and the adoption of Industry 4.0 technologies ”, Journal of World Business , Vol. 54 , 101017 .
Dalenogare , L.S. , Benitez , G.B. , Ayala , N.F. and Frank , A.G. ( 2018 ), “ The expected contribution of Industry 4.0 technologies for industrial performance ”, International Journal of Production Economics , Vol. 204 , pp. 383 - 394 .
Dalmarco , G. , Ramalho , F.R. , Barros , A.C. and Soares , A.L. ( 2019 ), “ Providing industry 4.0 technologies: the case of a production technology cluster ”, The Journal of High Technology Management Research , Vol. 30 , 100355 .
Di Carlo , F. , Mazzuto , G. , Bevilacqua , M. and Ciarapica , F.E. ( 2021 ), “ Retrofitting a process plant in an industry 4.0 perspective for improving safety and maintenance performance ”, Sustainability , Vol. 13 No. 2 , p. 646 .
Dieste , M. , Sauer , P.C. and Orzes , G. ( 2022 ), “ Organizational tensions in industry 4.0 implementation: a paradox theory approach ”, International Journal of Production Economics , Vol. 251 , 108532 .
Durach , C.F. , Kembro , J. and Wieland , A. ( 2017 ), “ A new paradigm for systematic literature reviews in supply chain management ”, Journal of Supply Chain Management , Vol. 53 , pp. 67 - 85 .
European Commission ( 2022 ), Industry 5.0, a Transformative Vision for Europe : Governing Systemic Transformations towards a Sustainable Industry , Publications Office of the European Union , Brussels .
Ford , S. and Despeisse , M. ( 2016 ), “ Additive manufacturing and sustainability: an exploratory study of the advantages and challenges ”, Journal of Cleaner Production , Vol. 137 , pp. 1573 - 1587 .
Frank , A.G. , Dalenogare , L.S. and Ayala , N.F. ( 2019 ), “ Industry 4.0 technologies: implementation patterns in manufacturing companies ”, International Journal of Production Economics , Vol. 210 , pp. 15 - 26 .
Garrido-Hidalgo , C. , Ramirez , F.J. , Olivares , T. and Roda-Sanchez , L. ( 2020 ), “ The adoption of internet of things in a circular supply chain framework for the recovery of WEEE: the case of lithium-ion electric vehicle battery packs ”, Waste Management , Vol. 103 , pp. 32 - 44 .
Ghobakhloo , M. ( 2020 ), “ Industry 4.0, digitization, and opportunities for sustainability ”, Journal of Cleaner Production , Vol. 252 , 119869 .
Ghobakhloo , M. and Fathi , M. ( 2019 ), “ Corporate survival in industry 4.0 era: the enabling role of lean-digitized manufacturing ”, Journal of Manufacturing Technology Management , Vol. 31 No. 1 , pp. 1 - 30 .
Ghobakhloo , M. , Fathi , M. , Iranmanesh , M. , Maroufkhani , P. and Morales , M.E. ( 2021 ), “ Industry 4.0 ten years on: a bibliometric and systematic review of concepts, sustainability value drivers, and success determinants ”, Journal of Cleaner Production , Vol. 302 , 127052 .
Global Reporting Initiative ( 2018 ), “ GRI standards, Amsterdam ”, available at: https://www.globalreporting.org/standards
Grigore , G. , Molesworth , M. , Miles , C. and Glozer , S. ( 2021 ), “ (Un) resolving digital technology paradoxes through the rhetoric of balance ”, Organization , Vol. 28 , pp. 186 - 207 .
Huang , S.H. , Liu , P. , Mokasdar , A. and Hou , L. ( 2013 ), “ Additive manufacturing and its societal impact: a literature review ”, The International Journal of Advanced Manufacturing Technology , Vol. 67 , pp. 1191 - 1203 .
Kagermann , H. , Helbig , J. , Hellinger , A. and Wahlster , W. ( 2013 ), Recommendations for Implementing the Strategic Initiative Industrie 4.0: Securing the Future of German Manufacturing Industry; Final Report of the Industrie 4.0 Working Group , Forschungsunion .
Kamble , S.S. , Gunasekaran , A. and Gawankar , S.A. ( 2018a ), “ Sustainable industry 4.0 framework: a systematic literature review identifying the current trends and future perspectives ”, Process Safety and Environmental Protection , Vol. 117 , pp. 408 - 425 .
Kamble , S.S. , Gunasekaran , A. and Sharma , R. ( 2018b ), “ Analysis of the driving and dependence power of barriers to adopt industry 4.0 in Indian manufacturing industry ”, Computers in Industry , Vol. 101 , pp. 107 - 119 .
Kembro , J. , Näslund , D. and Olhager , J. ( 2017 ), “ Information sharing across multiple supply chain tiers: a Delphi study on antecedents ”, International Journal of Production Economics , Vol. 193 , pp. 77 - 86 .
Kiel , D. , Müller , J.M. , Arnold , C. and Voigt , K.-I. ( 2020 ), “ Sustainable industrial value creation: benefits and challenges of industry 4.0 ”, International Journal of Innovation Management , Vol. 21 No. 8 , 1740015 .
Landeta , J. ( 2006 ), “ Current validity of the Delphi method in social sciences ”, Technological Forecasting and Social Change , Vol. 73 , pp. 467 - 482 .
Leso , V. , Fontana , L. and Iavicoli , I. ( 2018 ), “ The occupational health and safety dimension of Industry 4.0 ”, La Medicina del lavoro , Vol. 109 , 327 .
Li , Z. , Ye , J. and Wu , H. ( 2019 ), “ A virtual sensor for collision detection and distinction with conventional industrial robots ”, Sensors , Vol. 19 , 2368 .
Li , Y. , Dai , J. and Cui , L. ( 2020 ), “ The impact of digital technologies on economic and environmental performance in the context of industry 4.0: a moderated mediation model ”, International Journal of Production Economics , Vol. 229 , 107777 .
Linstone , H.A. and Turoff , M. ( 1975 ), The Delphi Method , Addison-Wesley , Reading, MA .
Lopes de Sousa Jabbour , A.B. , Jabbour , C.J.C. , Godinho Filho , M. and Roubaud , D. ( 2018 ), “ Industry 4.0 and the circular economy: a proposed research agenda and original roadmap for sustainable operations ”, Annals of Operations Research , Vol. 270 , pp. 273 - 286 .
MacCarthy , B.L. , Lewis , M. , Voss , C. and Narasimhan , R. ( 2013 ), “ The same old methodologies? Perspectives on OM research in the post‐lean age ”, International Journal of Operations and Production Management , Vol. 33 No. 7 , pp. 934 - 956 .
Merton , R.K. ( 1936 ), “ The unanticipated consequences of purposive social action ”, American Sociology Review , Vol. 1 , pp. 894 - 904 .
Mitchell , V.W. ( 1991 ), “ The Delphi technique: an exposition and application ”, Technology Analysis and Strategic Management , Vol. 3 , pp. 333 - 358 .
Müller , J.M. and Voigt , K.-I. ( 2018 ), “ Sustainable industrial value creation in SMEs: a comparison between industry 4.0 and made in China 2025 ”, International Journal of Precision Engineering and Manufacturing-Green Technology , Vol. 5 , pp. 659 - 670 .
Müller , J.M. , Buliga , O. and Voigt , K.I. ( 2018 ), “ Fortune favors the prepared: how SMEs approach business model innovations in industry 4.0 ”, Technological Forecasting and Social Change , Vol. 132 , pp. 2 - 17 .
Narayanamurthy , G. and Tortorella , G. ( 2021 ), “ Impact of COVID-19 outbreak on employee performance–moderating role of industry 4.0 base technologies ”, International Journal of Production Economics , Vol. 234 , 108075 .
Okoli , C. and Pawlowski , S.D. ( 2004 ), “ The Delphi method as a research tool: an example, design considerations and applications ”, Information and Management , Vol. 42 , pp. 15 - 29 .
Pegoraro , D. , De Propris , L. and Chidlow , A. ( 2022 ), “ Regional factors enabling manufacturing reshoring strategies: a case study perspective ”, Journal of International Business Policy , Vol. 5 , pp. 112 - 133 .
Rodriguez , F.S. , Saleem , K. , Spilski , J. and Lachmann , T. ( 2021 ), “ Performance differences between instructions on paper vs digital glasses for a simple assembly task ”, Applied Ergonomics , Vol. 94 , 103423 .
Schneider , S. and Kokshagina , O. ( 2021 ), “ Digital transformation: what we have learned (thus far) and what is next ”, Creativity and Innovation Management , Vol. 30 , pp. 384 - 411 .
Seuring , S. and Gold , S. ( 2012 ), “ Conducting content‐analysis based literature reviews in supply chain management ”, Supply Chain Management: An International Journal , Vol. 17 No. 5 , pp. 544 - 555 .
Seuring , S. , Brandenburg , M. , Sauer , P.C. , Schünemann , D.-S. , Warasthe , R. , Aman , S. , Qian , C. , Petljak , K. , Neutzling , D.M. and Land , A. ( 2022 ), “ Comparing regions globally: impacts of COVID-19 on supply chains–a Delphi study ”, International Journal of Operations and Production Management , Vol. 42 No. 8 , pp. 1077 - 1108 .
Singh , R. and Bhanot , N. ( 2020 ), “ An integrated DEMATEL-MMDE-ISM based approach for analysing the barriers of IoT implementation in the manufacturing industry ”, International Journal of Production Research , Vol. 58 , pp. 2454 - 2476 .
Stock , T. and Seliger , G. ( 2016 ), “ Opportunities of sustainable manufacturing in industry 4.0 ”, Procedia CIRP , Vol. 40 , pp. 536 - 541 .
Stock , T. , Obenaus , M. , Kunz , S. and Kohl , H. ( 2018 ), “ Industry 4.0 as enabler for a sustainable development: a qualitative assessment of its ecological and social potential ”, Process Safety and Environmental Protection , Vol. 118 , pp. 254 - 267 .
Sugiyama , M. , Deguchi , H. , Ema , A. , Kishimoto , A. , Mori , J. , Shiroyama , H. and Scholz , R.W. ( 2017 ), “ Unintended side effects of digital transition: perspectives of Japanese Experts ”, Sustainability , Vol. 9 , 2193 .
Tsai , C.-H. and Huang , J.-Y. ( 2018 ), “ Augmented reality display based on user behavior ”, Computer Standards and Interfaces , Vol. 55 , pp. 171 - 181 .
Väisänen , A.J.K. , Hyttinen , M. , Ylönen , S. and Alonen , L. ( 2019 ), “ Occupational exposure to gaseous and particulate contaminants originating from additive manufacturing of liquid, powdered, and filament plastic materials and related post-processes ”, Journal of Occupational and Environmental Hygiene , Vol. 16 , pp. 258 - 271 .
von der Gracht , H.A. ( 2012 ), “ Consensus measurement in Delphi studies: review and implications for future quality assurance ”, Technological Forecasting and Social Change , Vol. 79 , pp. 1525 - 1536 .
Wang , C.-H. , Tsai , N.-H. , Lu , J.-M. and Wang , M.-J.J. ( 2019 ), “ Usability evaluation of an instructional application based on google glass for mobile phone disassembly tasks ”, Applied Ergonomics , Vol. 77 , pp. 58 - 69 .
Yoon , H.-S. , Lee , J.-Y. , Kim , H.-S. , Kim , M.-S. , Kim , E.-S. , Shin , Y.-J. , Chu , W.-S. and Ahn , S.-H. ( 2014 ), “ A comparison of energy consumption in bulk forming, subtractive, and additive processes: review and case study ”, International Journal of Precision Engineering and Manufacturing-Green Technology , Vol. 1 , pp. 261 - 279 .
Zheng , T. , Ardolino , M. , Bacchetti , A. and Perona , M. ( 2021 ), “ The applications of Industry 4.0 technologies in manufacturing context: a systematic literature review ”, International Journal of Production Research , Vol. 59 , pp. 1922 - 1954 .
Acknowledgements
Funding: This paper is part of the research activities of the project BT-OSCM funded by the Free University of Bozen-Bolzano with the RTD 2020 call [CUP I54I20001030005].
The authors would like to thank Prof. Shane Schvaneveldt (Weber State University, USA) and Dr. Matteo Podrecca (University of Udine, Italy) for their advice and comments on the study. They extend their gratitude to all the participants in the Delphi study for their contribution to the data collection.
Corresponding author
Related articles, we’re listening — tell us what you think, something didn’t work….
Report bugs here
All feedback is valuable
Please share your general feedback
Join us on our journey
Platform update page.
Visit emeraldpublishing.com/platformupdate to discover the latest news and updates
Questions & More Information
Answers to the most commonly asked questions here
What are Industry 4.0, the Fourth Industrial Revolution, and 4IR?
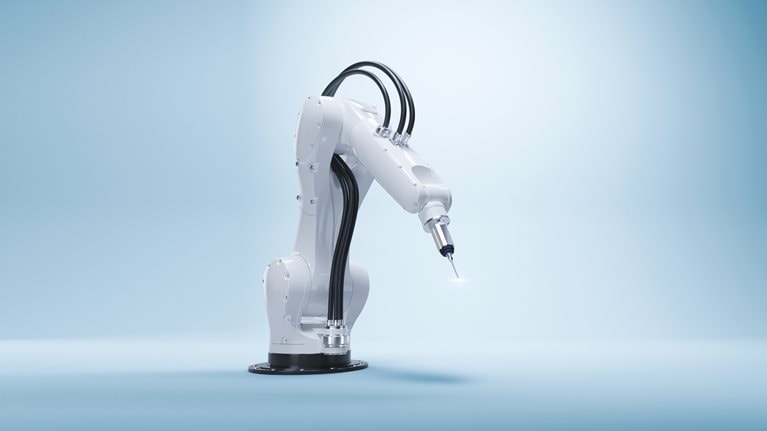
Augmented reality, machine automation, and more: the 21st-century industrial revolution is digital. Industry 4.0, the Fourth Industrial Revolution, and 4IR all refer to the current era of connectivity, advanced analytics, automation, and advanced-manufacturing technology that has been transforming global business for years. This wave of change in the manufacturing sector began in the mid-2010s and holds significant potential for operations and the future of production .
What is the Fourth Industrial Revolution?
Get to know and directly engage with senior mckinsey experts on industry 4.0..
Enno de Boer is a senior partner in McKinsey’s New Jersey office. Kweilin Ellingrud is a senior partner and a director of McKinsey Global Institute in the Minneapolis office. Gérard Richter is a senior partner in the Frankfurt office and leader of McKinsey Digital Hubs and Build by McKinsey in Europe. Daniel Swan is a senior partner in the Stamford, Connecticut, office.
Steam propelled the original Industrial Revolution ; electricity powered the second; preliminary automation and machinery engineered the third; and cyberphysical systems—or intelligent computers—are shaping the Fourth Industrial Revolution .
Before 2014, the Google search term “Industry 4.0” was practically nonexistent, but by 2019, 68 percent of respondents to a McKinsey global survey regarded Industry 4.0 as a top strategic priority. Seventy percent said their companies were already piloting or deploying new technology.
4IR builds on the inventions of the Third Industrial Revolution—or digital revolution—which unfolded from the 1950s and to the early 2000s and brought us computers, other kinds of electronics, the Internet, and much more. Industry 4.0 brings these inventions beyond the previous realm of possibility with four foundational types of disruptive technologies (examples below) that can be applied all along the value chain:
- connectivity, data, and computational power: cloud technology , the Internet, blockchain , sensors
- analytics and intelligence: advanced analytics, machine learning, artificial intelligence
- human–machine interaction: virtual reality (VR) and augmented reality (AR) , robotics and automation , autonomous guided vehicles
- advanced engineering: additive manufacturing (such as, 3-D printing), renewable energy , nanoparticles
Technology, however, is only half of the Industry 4.0 equation. To thrive in the Fourth Industrial Revolution, companies must ensure that their workers are properly equipped through upskilling and reskilling and then hire new people when necessary. Upskilling means that employees learn new skills to help them in their current positions as the skills they need evolve. Reskilling is the real challenge: workers are retrained with new skills that will enable them to fill different positions within their companies.
This is increasingly vital as disruptive technologies transform job requirements, but the outlook on reskilling differs geographically . In Europe, 94 percent of surveyed executives believe that the balance between hiring and reskilling should be equal or tip toward reskilling, compared with only 62 percent of US respondents.
The end-to-end skill transformation has three phases:
- scout —analyze the skills required to achieve a company’s ambitions
- shape —identify talent gaps that must be addressed and design the program infrastructure to address them
- shift —develop and implement content and delivery mechanisms to train workers at scale
A conversation with Francisco Betti (head of the Platform for Shaping the Future of Advanced Manufacturing and Production, launched by the World Economic Forum in 2017) and the CEOs of Flex, Protolabs, and Western Digital offers perspective and real-world insights on building workforce capabilities and shifting mindsets for successful digital transformations in manufacturing. The benefits can go far beyond business outcomes. In the words of Western Digital CEO David Goeckeler, “It’s not just about our company being better and us being prepared for the future; it’s about all of our employees being ready for that future—keeping them at the center, having them highly engaged, all of the reskilling, getting them excited about what the future holds.”
Learn more about our Operations , Advanced Electronics , Financial Services , Technology , Media & Telecommunications , and Sustainability practices.
What is The Global Lighthouse Network?
The World Economic Forum , in collaboration with McKinsey, launched the Global Lighthouse Network (GLN) in 2018 to identify organizations and technologies in the vanguard of the Fourth Industrial Revolution. A lighthouse (in this context) is a manufacturing site that has successfully implemented 4IR technologies at scale, with a significant operational impact.
Lighthouses aim to capture more than 80 percent of the identified value of chosen use cases—meaning those involving 4IR technologies. Ultimately, these sites are intended to serve as an Industry 4.0 benchmark for the transformation of other sites. This may sound similar to the concept of a digital factory—because it is. Digital factories serve as “construction sites” for companies to implement 4IR technologies and test-run new operations before applying the advances at scale. The difference is that the GLN specifically identifies lighthouses as successful 4IR trailblazers.

Introducing McKinsey Explainers : Direct answers to complex questions
Today, 103 lighthouses—such as Tata Steel’s plant in Kalinganagar, India , and select Henkel Laundry & Home Care production sites—have been identified around the world.
Lighthouses can be built practically anywhere, by small or big companies, in developing or developed economies, and at greenfield or brownfield locations.
Insights from lighthouses that are successfully using 4IR technologies today offer something of a playbook for organizations shaping the future of manufacturing . Digital transformation at scale isn’t easy, but responsible production—combining productivity, sustainability, and active workforce engagement—is within reach.
To get there, six core enablers can boost the odds of success for your company’s 4IR transformation:
- An agile approach that incorporates quick iterations, fast fails, and continuous learning, with teams transforming bundled use cases in waves to drive innovation and ongoing refinements.
- Agile digital studios can help people collaborate effectively, providing designated space where team members from different functions are in proximity for co-creation.
- The IIoT stack allows for seamless integration of IIoT infrastructure (both legacy and new) to build a stable, flexible tech backbone. Costs can by limited by leveraging existing systems with efficient investment in a new technology stack.
- An IIoT academy uses adult-learning best practices to upskill the workforce, offering customized learning programs based on the unique individual needs.
- Tech ecosystems partner with vendors, suppliers, customers, and related industries to source the latest capabilities, offering access to extensive data sets and creating opportunities for innovating together.
- Transformation offices can form a governance hub to support the launch and scale-up of a lighthouse, making progress and priorities transparent, ensuring value continues to be captured, and accelerating change.
Of these, two are particularly important: an agile approach and a transformation office .
Any company can begin its Industry 4.0 journey in a small way at one site and then scale up quickly. Otherwise, it could be doomed to “ pilot purgatory ”: companies try out (or pilot) new technologies but fail to apply them at scale, stalling the 4IR transition. As of late 2020, about 74 percent of surveyed companies reported being in pilot purgatory.
What are the advantages of the Fourth Industrial Revolution?
The Fourth Industrial Revolution could make products and services more easily accessible and transmissible for businesses, consumers, and stakeholders all along the value chain. Preliminary data indicate that successfully scaling 4IR technology makes supply chains more efficient and working hours more productive, reduces factory waste, and has countless other benefits for employees, stakeholders, and consumers.
Implementing Industry 4.0 technology is also especially advantageous amid the challenges of the pandemic. In fact, COVID-19 has accelerated the 4IR transition because physical distancing and shifting consumer demands forced companies to embrace digitization and contactless operations. Six months into the pandemic, 94 percent of the respondents to a McKinsey survey said that Industry 4.0 had helped keep the operations of their companies running, and 56 percent considered these technologies critical to the crisis response.
Before the pandemic, the top motivators for companies to digitize varied by industry. But in 2020, three drivers were common across all sectors and geographies: agility, flexibility, and manufacturing efficiency. Companies that had already scaled up Industry 4.0 technologies before COVID-19 were better positioned to handle the challenges that arose.
- One consumer-packaged-goods company in Asia built a digital twin of its supply chain to simulate different scenarios. During the pandemic, the company used this simulator to prepare for sudden shutdowns or disruptions in the supply of materials.
- When one coffee machine plant in Treviso, Italy, transformed itself from an uncompetitive site into a manufacturing lighthouse, labor productivity rose by 33 percent and lead times fell by 82 percent.
- Of the surveyed companies that had not begun implementing Industry 4.0 technologies, 56 percent felt constrained in their responses to the pandemic’s challenges as a result.
Learn more about our Operations practice.
Tell me more about the Fourth Industrial Revolution, workforce engagement, and the future of manufacturing?
Workforce engagement is vital to a successful 4IR transformation. Indeed, even a company with the best tools, newest technology, and immense resources is unlikely to scale up a 4IR transformation successfully if the workforce is not engaged. A concerted focus on people has also helped organizations build resilience by helping workers develop new skills, so that the business can respond more flexibly to change. In practice, this could entail rethinking training and skill development pathways, and make structural changes for the longer term.
Here are five areas where manufacturers are already promoting high workforce engagement:
- learning and development (for example, with extended competencies and skills, via the combination of hard, soft and digital skills, and apprenticeship )
- empowerment and ownership (for instance, through outcome and results-oriented steering, or by encouraging workers to make their own decisions)
- collaborations and connections (say, by working with cross-functional and multiskilled teams, or developing extended networks in the organization and beyond)
- impact and recognition (for example, by creating accountability for achievements or by celebrating successes)
- the voice of the worker (for instance, by using digital channels and data to gather input from workers’ voices, or by seeking to understand their hidden needs)
How about Industry 4.0 digitization and opportunities for sustainability?
The Fourth Industrial Revolution creates opportunities for sustainability and, more important, these advances are inherently more sustainable than current business practices. Some people think productive operations are hard to square with environmental responsibility, but sustainable lighthouses challenge that notion: 4IR transformations facilitate a viable kind of eco-efficiency that intrinsically meshes sustainability with competitive excellence.
Eco-efficiency includes three dimensions of digital technology:
- enabling data-informed actions in production and the broader end-to-end value chain
- realizing improvements across performance indicators, such as cost, agility, convenience, and quality
- driving sustainability gains by limiting consumption, resource waste, and emissions
Consider a few examples of how Industry 4.0 technologies that maximize efficiency also minimize waste:
- One Singapore lighthouse decreased its scrap output from building semiconductors by 22 percent in a smart factory enabled by the industrial Internet of Things, or IIoT. (See a related Explainer, “ What is the Internet of Things? ,” for more.)
- Schneider Electric’s smart factory in Lexington, Kentucky, combined IoT connectivity and predictive analytics to lower energy use by 26 percent, CO 2 emissions by 30 percent, and water use by 20 percent.
- Sixty percent of the 103 lighthouses identified by the Global Lighthouse Network include sustainability among their top five Fourth Industrial Revolution use cases.
And more broadly, lighthouses demonstrate how 4IR technologies can promote responsible growth in the long term. How? Through action in three broad areas:
- Environmental: Taking care of our planet and the surrounding environment. Areas of focus for lighthouses in this category include energy, water, waste, greenhouse-gas emissions, and the circular economy .
- Social: Building a stronger workforce and community. For lighthouses, focus areas might include human-capital development, the voice of the worker, health and safety, and labor standards.
- Governance: Establishing a set of practice, controls, and procedures to govern, make decisions, and meet the needs of stakeholders. This can encompass focus areas such as ownership, accountability, business ethics, and governance structure.
Learn more about our Operations , Digital McKinsey , and Sustainability practices.
What is Industry 4.0’s impact on the economy?
Industry 4.0 will continue to have a significant impact on the economy. The greatest economic boons will go to the fastest-acting companies.
According to a 2018 McKinsey Global Institute analysis, Industry 4.0 front-runners—facilities well on their way to adopting AI and other advanced technologies by 2025—can expect a 122 percent positive cash flow change . Follower companies can expect just 10 percent, while companies that wholly fail to adopt AI could see a 23 percent downturn.
Industry 4.0 is also projected to transform the skill sets of the workforce by shifting the standards for sought-after talent. Over the coming decade, we will see these changes as more and more companies embrace robotics:
- Demand for physical and manual skills in repeatable tasks, like those on assembly lines, will decline by nearly 30 percent.
- Demand for basic literacy and numeracy skills will decline by almost 20 percent.
- Demand for technological skills such as coding will rise by more than 50 percent.
- Demand for complex cognitive skills will rise by about 33 percent.
- Demand for high-level social and emotional skills will rise by more than 30 percent.
In 2025, the value creation potential of Industry 4.0 for manufacturers and suppliers is expected to reach $3.7 trillion .

What industries are being transformed by Industry 4.0?
Every single industry will be transformed during the Fourth Industrial Revolution, but some to a greater degree than others. The nature of the Industry 4.0 transition will differ by the specific types of technology being adopted, as well as the existing infrastructure and skills of organizations. The transformation can be broken down into three archetypes of adoption pathways :
- Accelerated. Regardless of a company’s existing tech infrastructure (whether advanced or nonexistent) certain inexpensive digital, augmented-reality, and automation solutions are rapidly adoptable without transition headaches.
- Differential. The existing tech infrastructure will affect how quickly some technologies are adopted. Companies with less foundational information technology (IT), operations technology, and data infrastructure will need time to transition. More advanced companies are better equipped for quick implementation.
- Slowed or deferred. Even at companies with an advanced tech infrastructure, the adoption of the most cutting-edge innovations (such as full end-to-end automation) will be slow because of the high level of required capital expenditure and the unclear long-term payback.
Operationally intensive sectors, such as manufacturing, transportation, and retailing, will experience the greatest change because many companies in these sectors employ large numbers of people for tasks particularly suited for automation or digitization. Operations-intensive sectors have 1.3 times more automation potential than others do.
In these operations-intensive sectors, McKinsey analysis indicates that up to 58 percent of work activities could be automated with current technology. Education, by contrast, is projected to undergo the least degree of change during Industry 4.0; only 25 percent of the sector’s work is automatable.
Learn more about our Operations practice.
For more in-depth exploration of these topics, see McKinsey’s collection of insights on operations . Learn more about manufacturing and supply chain consulting , and check out manufacturing-related job opportunities if you’re interested in working at McKinsey.
Articles referenced include:
- “ CEO dialogue: Perspectives on productivity and sustainability ,” November 3, 2021, Enno de Boer , Yves Giraud, and Daniel Swan
- “ Lighthouses unlock sustainability through 4IR technologies ,” September 27, 2021, Francisco Betti, Enno de Boer , and Yves Giraud
- “ A manufacturer’s guide to scaling industrial IoT ,” February 5, 2021, Andreas Behrendt , Enno de Boer , Tarek Kasah, Bodo Koerber, Niko Mohr , and Gérard Richter
- “ Building the vital skills for the future of work in operations ,” August 7, 2020, Kweilin Ellingrud , Rahul Gupta, and Julian Salguero
- “ Industry 4.0: Reimagining manufacturing operations after COVID-19 ,” July 29, 2020, Mayank Agrawal, Karel Eloot , Matteo Mancini , and Alpesh Patel
- “ Lighthouses reveal a playbook for responsible industry transformation ,” March 30, 2022, Francisco Betti, Enno de Boer , and Yves Giraud
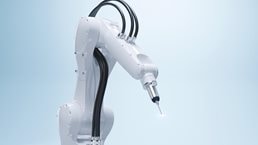
Want to know more about Industry 4.0?
Related articles.
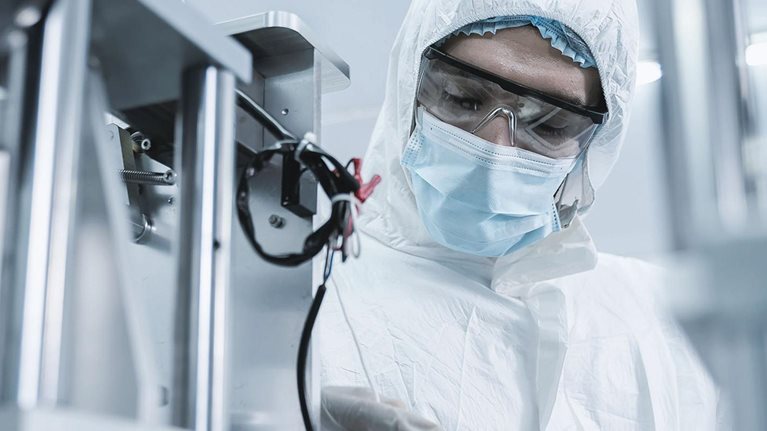
Lighthouses reveal a playbook for responsible industry transformation
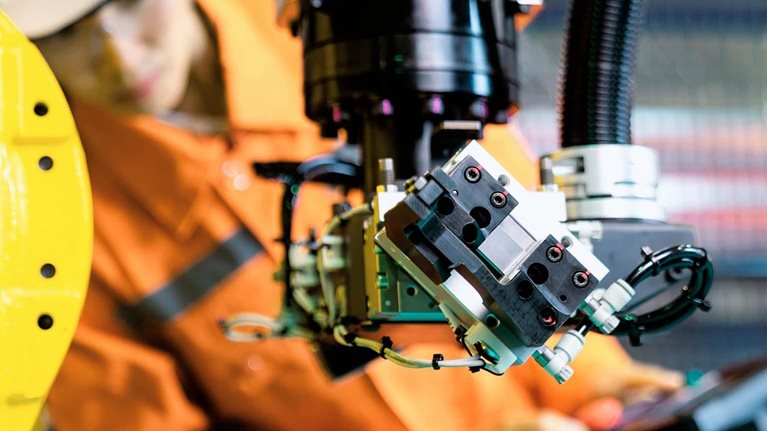
CEO dialogue: Perspectives on productivity and sustainability
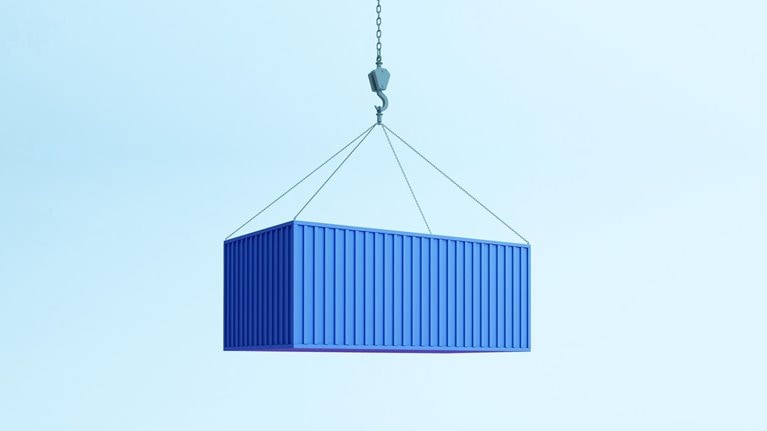
What is supply chain?
- Reviews / Why join our community?
- For companies
- Frequently asked questions
The Fourth Industrial Revolution
What is the fourth industrial revolution.
The Fourth Industrial Revolution (4IR), also known as Industry 4.0, is a new era of development in which digital, physical and biological systems converge, fundamentally transforming industries, economies and societies.
The term Fourth Industrial Revolution was coined by Klaus Schwab, Founder and Executive Chairman of the World Economic Forum (WEF). He introduced this concept in his book, The Fourth Industrial Revolution, published in 2016. In it, he discusses how emerging technologies like artificial intelligence (AI), the Internet of Things (IoT) and robotics have begun to merge with the physical, digital and biological worlds and, thus, have revolutionized economies, industries and societies in the process.
In this video, discover how the 4IR is transforming the world:
- Transcript loading…
The 4IR’s alternate name, Industry 4.0, is usually referred to in the context of the manufacturing and industrial sectors. This term highlights the revolution's focus on the integration of digital technologies into the heart of industry to create smart factories that embody the convergence of the physical and digital worlds.
This revolution is distinguished by its unprecedented speed, scope and impact on human life—it offers immense opportunities for progress but also poses significant challenges, including ethical considerations and the potential for increased inequality. Klaus Schwab argues that this era is more than just a technological upgrade—it’s an opportunity to help everyone, including leaders, policymakers and people from all income groups and nations, to harness converging technologies in order to create an inclusive, human-centered future. The 4IR compels us to rethink how we create, exchange and distribute value, with particular emphasis on the need for global cooperation and inclusive policies to harness its potential for the betterment of humanity.
The 4IR expands upon the breakthroughs of the Third Industrial Revolution, also known as the digital revolution, that occurred from the 1950s through the early 2000s. During this time, innovations like computers, diverse electronic devices, the Internet and numerous other technological advances emerged.
Fourth Industrial Revolution: Integration of Design and Technology
The 4IR is marked by the integration of technologies like AI, IoT, robotics and VR, which demands a holistic design approach that considers not only the form and function but also the interconnectedness and intelligence of products and systems.
The Apple Vision Pro epitomizes the convergence of design, technology, AI and VR—it’s a significant release of the Fourth Industrial Revolution. This device combines Apple's renowned design ethos with cutting-edge virtual reality capabilities to offer users immersive experiences that blur the line between the digital and physical worlds. The Vision Pro is powered by sophisticated AI to deliver personalized, intuitive interactions—it’s expected to set a new standard for how technology interfaces with human behavior.
Watch Apple’s first announcement video for the Vision Pro:
As technology becomes more embedded in everyday life, design in the 4IR emphasizes user-centric solutions and personalized experiences, enabled by data analytics and machine learning. There's also a growing focus on sustainable and circular design principles driven by global challenges like climate change and resource scarcity.
The complexity of 4IR technologies requires designers to work collaboratively across disciplines, integrating insights from engineering, biology, computer science and psychology. This interdisciplinary approach is crucial for innovation and for addressing the ethical, social and environmental implications of new technologies.
The 4IR encourages designers to engage in speculative and critical design practices, exploring future scenarios and the societal impact of emerging technologies. This approach helps to envision potential futures and guide the development of technology in a responsible and human-centered direction.
What Are the Key Technologies of the 4IR
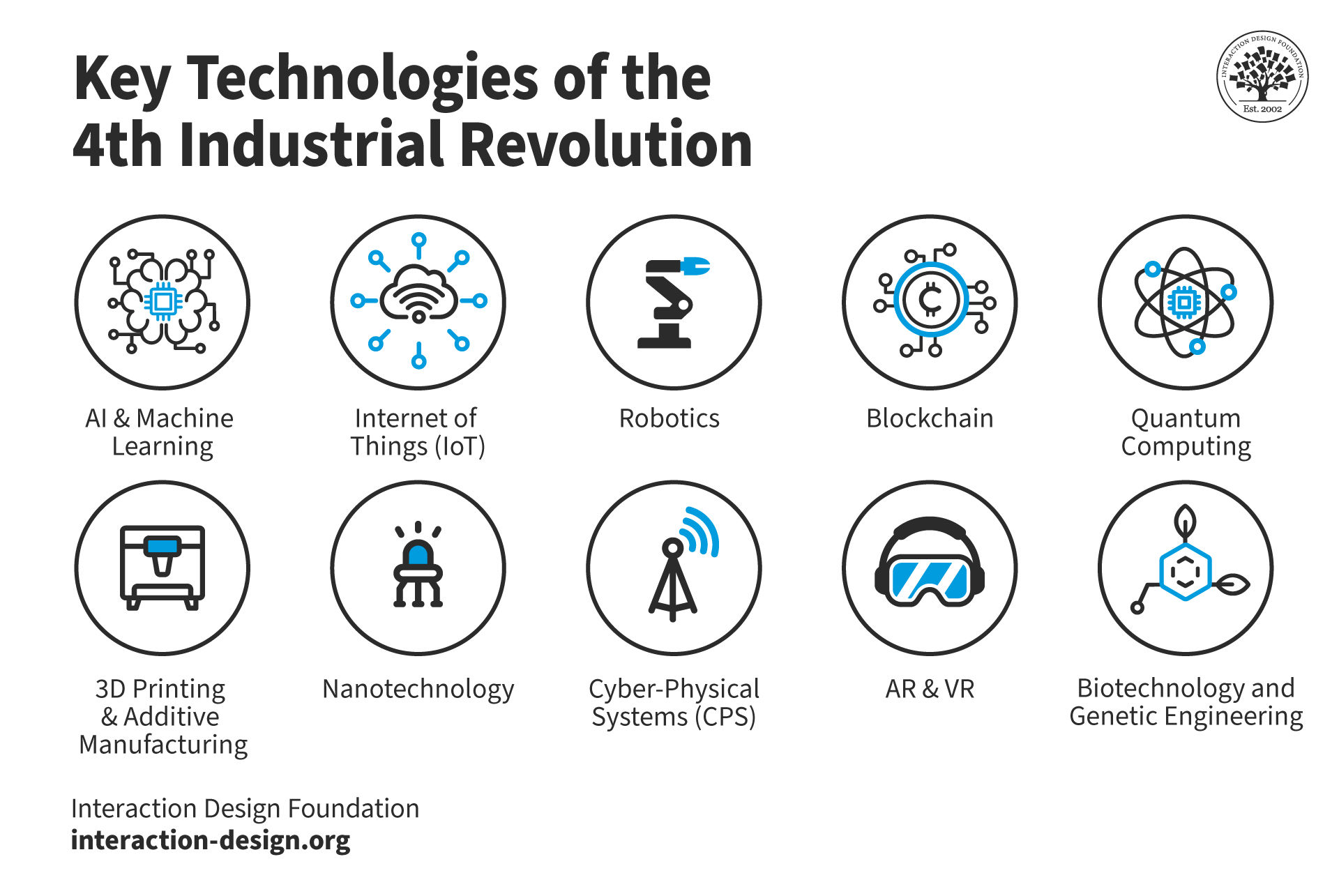
© Interaction Design Foundation, CC BY-SA 4.0
Artificial Intelligence (AI) and Machine Learning
AI involves machines and programs capable of performing tasks that typically require human intelligence. Machine learning, a subset of AI, enables computers to learn from data and improve over time. These technologies are revolutionizing sectors by enhancing decision-making, automating tasks and creating new services and products.
In this video, AI Product Designer Ioana Teleanu discusses AI’s impact on the world:
Learn more about machine learning in this video:
Internet of Things (IoT)
IoT refers to the network of physical objects embedded with sensors, software and other technologies for the purpose of connecting and exchanging data with other devices and systems over the internet. This interconnectivity enables more efficient processes and improved data analytics, which impacts everything from home automation to industrial manufacturing.
Smart lighting product, Philips Hue, uses IoT technology to offer a wide range of smart bulbs, lamps, and light fixtures that can be controlled via the Philips Hue app or through integration with other smart home systems. These lights can change color, brightness, and even sync with media content for an immersive experience. See how Philips uses IoT in their product expansion, Philips Hue Secure, in this video:
Robotics technology involves the design, construction, operation and use of robots for various tasks. With advancements in AI and machine learning, robots are becoming increasingly sophisticated, capable of performing complex tasks autonomously or augmenting human capabilities in industries like manufacturing, healthcare and services.
In this video, Robotic company Boston Dynamics demonstrates how their robot Atlas can aid in construction:
Blockchain
Blockchain is a decentralized ledger of all transactions across a network, which enables secure, transparent and tamper-proof record-keeping. While it underpins cryptocurrencies like Bitcoin, its applications extend to secure transactions, smart contracts and supply chain management.
Organizations like IBM's Food Trust network uses blockchain to trace the production, processing, and distribution of food products to enhance safety and reduce waste.
Quantum Computing
Quantum computing represents a significant leap forward in computing power—it uses principles of quantum mechanics to process information at speeds unattainable by traditional computers. This technology has the potential to revolutionize fields such as cryptography, drug discovery and complex system simulation.
Google's quantum AI lab is researching how quantum computing could accelerate machine learning tasks by processing complex data more efficiently than classical computers. Learn more in this video:
3D Printing and Additive Manufacturing
3D printing builds objects layer by layer from digital models. This offers unprecedented flexibility in manufacturing. It enables rapid prototyping, custom manufacturing and complex designs not possible with traditional methods which impacts industries from healthcare (with prosthetics and organ printing) to aerospace and automotive.
In this video by Mayo Clinic, 3D printing is used to create more hygienic and effective casts and splints for a patient with fractures and other injuries:
Biotechnology and Genetic Engineering
Advances in biotechnology and genetic engineering have enabled us to manipulate living organisms or their components to develop or make products, which improves healthcare, agriculture and environmental sustainability. Techniques like CRISPR-Cas9 gene editing have opened new possibilities for disease treatment and precision medicine.
Learn more about gene editing in this video by TED-Ed:
Nanotechnology
Nanotechnology manipulates matter at the atomic and molecular scale and promises significant advancements in materials science, medicine and electronics. Its applications range from more effective drug delivery systems to water treatment processes that remove contaminants at a molecular level.
In this video by Johns Hopkins Institute for NanoBioTechnology, learn how nanotechnology can be used to fight cancer:
Augmented Reality (AR) and Virtual Reality (VR)
AR and VR technologies are changing the way we interact with digital environments. AR overlays digital information onto the physical world, while VR creates immersive digital environments. These technologies have applications in education, training, entertainment and beyond.
Learn more about VR, its history and its future in this video:
Cyber-Physical Systems (CPS)
CPS are integrations of computation, networking and physical processes. Embedded computers and networks monitor and control the physical processes, with feedback loops where physical processes affect computations and vice versa. This integration is foundational for smart grids, autonomous vehicle systems and smart factories.
In this video watch how a Tesla vehicle drives itself:
These technologies are not only transformative in their own right, but are also interrelated. They often converge to create innovative solutions and opportunities across a variety of sectors and different levels of society and the economy. The potential of the 4IR lies in how these technologies are harnessed to drive forward human progress, address global challenges and reshape the world for the better.
The Impact of the 4IR: Case Studies
Environmental protection: iot for monitoring and conservation .
Rainforest Connection transforms recycled smartphones into solar-powered acoustic devices that monitor rainforest sounds. AI algorithms analyze these sounds to detect illegal logging and poaching in real time, enabling rapid response to protect wildlife and forests. This case study highlights how 4IR technologies can be creatively applied to combat environmental destruction and biodiversity loss.
Learn more about Rainforest Connection’s work in this video:
Agro 4.0: More Efficient Farming
The World Economic Forum’s (WEF) Centre for the Fourth Industrial Revolution (C4IR) introduced technology to small and medium farms in Colombia. The technology includes soil, water and climate sensors, as well as AI, cloud computing and drones. The project managed to reduce the farmer's costs by 30% and increase their yields by 20%.
Watch the C4IR video to learn more
Healthcare: AI-Driven Diagnostics and Personalized Medicine
Google's DeepMind developed an artificial intelligence system that can accurately detect over 50 types of eye diseases from 3D scans. Scientists from Google's DeepMind division, University College London (UCL) and Moorfields Eye Hospital developed software through deep learning techniques that can detect numerous prevalent eye conditions from 3D scans and subsequently recommend treatment options for the patient. This technology enables early diagnosis and treatment to potentially prevent vision loss in millions of people worldwide. Not only does it improve diagnostic accuracy and patient outcomes, but it can also reduce healthcare costs.
© UCL, Moorfields, DeepMind, et al, Fair Use
What are the Impacts of the 4IR?
The 4IR is not just a technological revolution; it's a catalyst for comprehensive change—how we live, work and relate to one another. Here are some of the major impacts and implications of the 4IR:
Economic Transformation
Productivity and efficiency : The integration of technologies like AI, robotics and IoT significantly boosts productivity and operational efficiencies across industries. In most cases, this leads to reduced costs, improved production rates and enhanced product quality.
New business models and markets : The 4IR has enabled new, innovative business models (e.g., platform-based economies like Airbnb and sharing economies like Uber) and the creation of markets that didn't exist before, particularly in the digital and service sectors.
Job displacement and creation : While automation and AI have displaced many traditional jobs, particularly in manufacturing and routine white-collar tasks, they also create new jobs that require advanced digital skills and competencies in technology development, data analysis and cybersecurity.
Societal Changes
Education and skill development : There's a growing need for education systems to adapt and an emphasis on STEM education, critical thinking, creativity and lifelong learning to prepare individuals for the jobs of the future.
Inequality and digital divide : The benefits of the 4IR risk being unevenly distributed, which could exacerbate income inequality and widen the digital divide between those with access to new technologies and skills and those without.
Enhanced connectivity and communication : The global proliferation of the internet and mobile devices has led to unprecedented levels of connectivity to enable new forms of social interaction, collaboration and information exchange.
Technological Advancements
Accelerated innovation : The rapid pace of technological advancement in fields like biotechnology, nanotechnology and quantum computing has already begun to revolutionize healthcare, energy and other industries.
Cybersecurity challenges : As more devices and systems are connected, vulnerabilities to cyber-attacks increase. Data privacy and system security are increasingly critical challenges.
Environmental Considerations
Sustainable development : Technologies emerging from the 4IR offer promising solutions to environmental challenges, including more efficient resource use, renewable energy technologies and smarter, more sustainable cities.
Climate change mitigation : Advances in technology are crucial for monitoring environmental changes, improving energy efficiency and developing new methods for carbon capture and storage to combat climate change.
Ethical and Governance Issues
Ethical considerations : The development and application of technologies like AI and genetic engineering raise profound ethical questions about privacy, consent and the nature of human identity.
Regulation and governance : There is an increasing need for effective governance frameworks to ensure that the development and deployment of new technologies are aligned with societal values and ethical principles. Policymakers are challenged to keep pace with technological innovation while safeguarding public interests.
The History of the World’s Industrial Revolutions
The 4IR is built upon the foundation laid by the three previous industrial revolutions, each marked by a significant leap in technological capabilities that transformed societies and economies. It's important to understand these precursors as they provide essential context to grasp the scale and scope of the changes the 4IR represents.
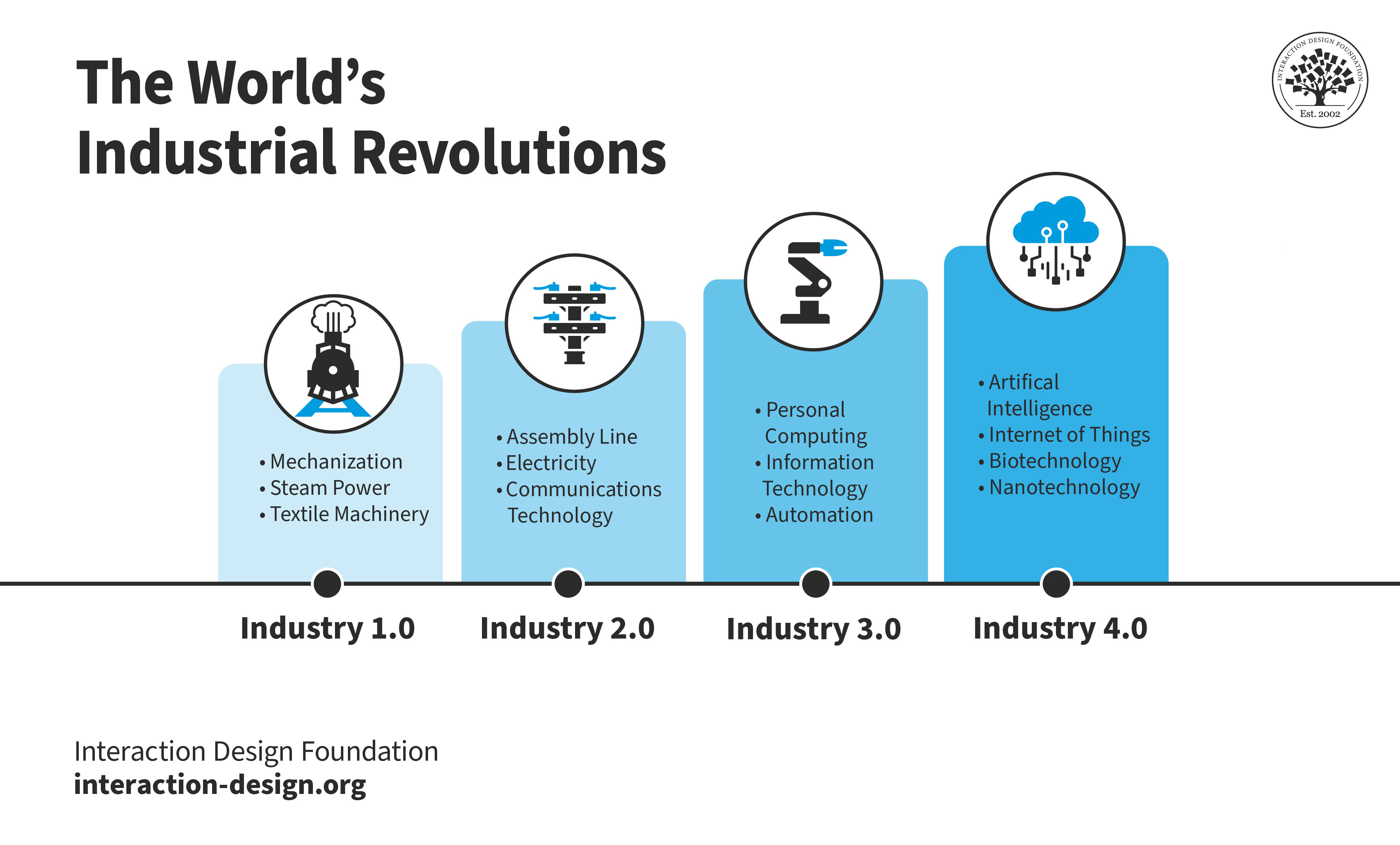
First Industrial Revolution: Late 18th to Early 19th Century
The first Industrial Revolution’s start and end date are widely debated, but the general consensus is that it spanned from about 1760 to 1840. It was characterized by the transition from hand production methods to machines through the use of steam power and water power. The textile industry was among the first to be transformed, with the invention of the spinning jenny and the power loom. This era saw the rise of mechanized factories, which significantly increased production capabilities and led to urbanization as people moved to cities for work.
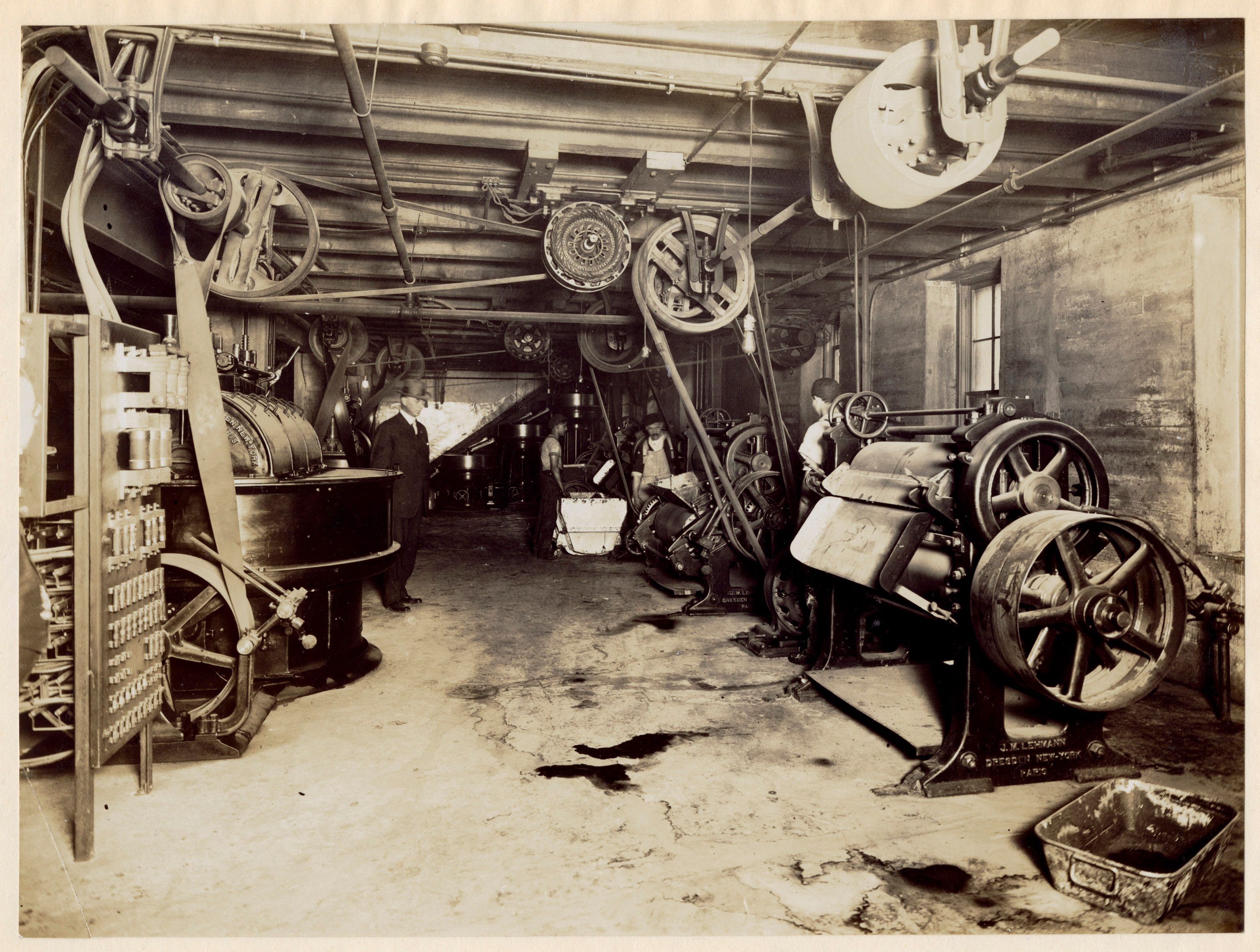
A factory from the First Industrial Revolution. The machinery harnessed steam and water power.
© National Geographic, CC BY-SA 4.0
Second Industrial Revolution: Late 19th to Early 20th Century
This period is roughly dated between 1870 and the beginning of World War I in 1914. The Second Industrial Revolution was marked by the introduction of electricity—this transformation led to more advanced manufacturing and production technologies. The development of the assembly line, notably used by Henry Ford in the mass production of automobiles, drastically increased efficiency and made goods more accessible to the masses. This period also saw significant advancements in chemical, electrical and steel production.
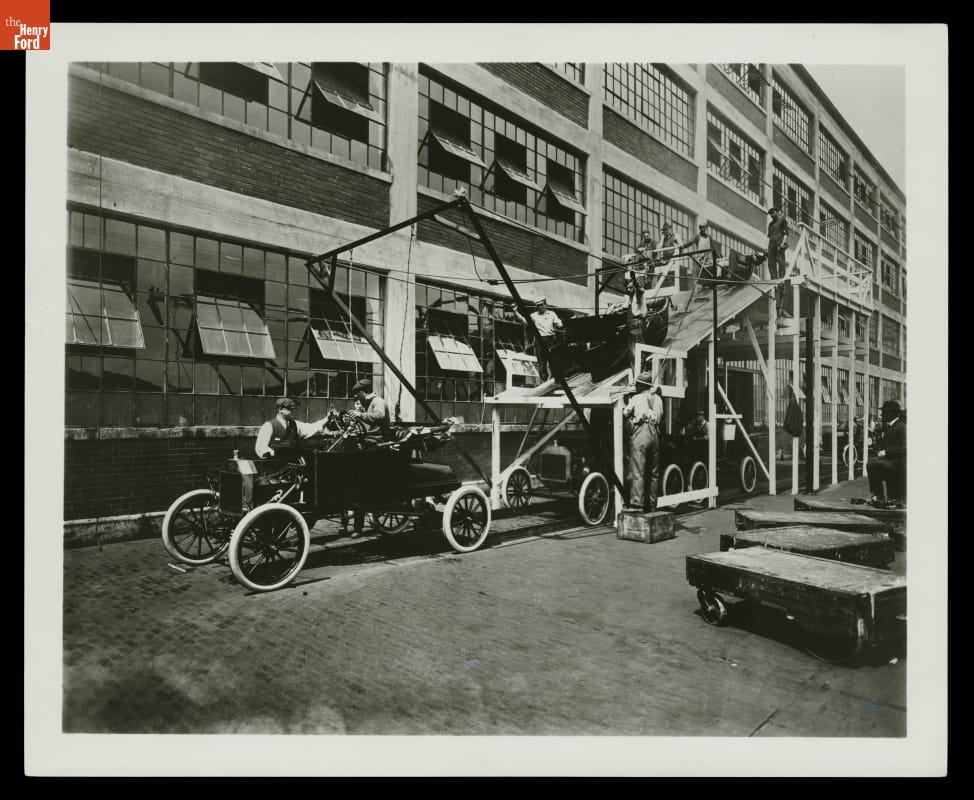
The Ford Model T assembly line circa 1913-1914. Henry Ford was one of the first to use an assembly line for mass production. When a Model T left the assembly line at Ford's Highland Park plant to be shipped by rail, it was not fully assembled. In this photograph, workers temporarily place bodies onto a chassis. At the loading dock, bodies and wheels would be removed and packed separately to conserve freight car space. Full assembly took place at branch plants closer to the vehicles' final destination.
© The Henry Ford, CC BY-SA 4.0
Third Industrial Revolution: Mid-Late 20th Century
Also known as the Digital Revolution, this era started around the 1950s-1970s. It’s defined by the move from analog electronic and mechanical devices to digital technologies. The invention of the personal computer, the internet and information and communications technology (ICT) transformed the way people live, work and communicate. It laid the groundwork for the globalized, interconnected world of today. The Third Industrial Revolution transitioned into the Fourth Industrial Revolution around the early 21st century, so there is no definitive end date for this period.
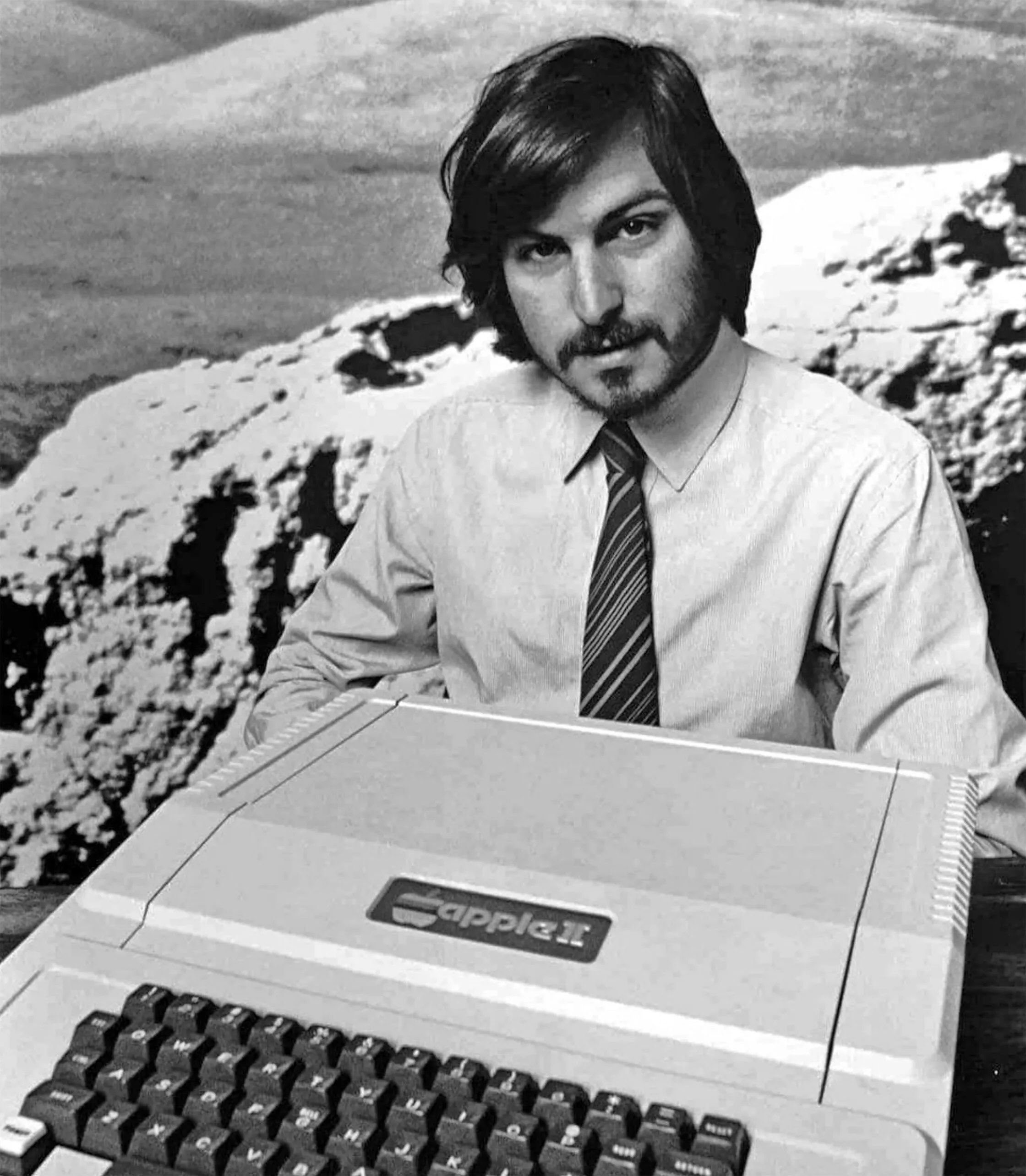
Steve Jobs with the Apple II. It was released in 1977 and is an example of an early personal computer.
© Alamy, CC BY-SA 4.0
Fourth Industrial Revolution: 21st Century
The 4IR builds on the digital revolution and is marked by a fusion of technologies that blur the lines between the physical, digital and biological. It’s characterized by breakthroughs in a range of areas including AI, robotics, the Internet of Things, genetic engineering, quantum computing and others. Unlike previous revolutions, the 4IR evolves at an exponential rate, transforming almost every industry and many aspects of human life.
Each industrial revolution brought about drastic changes in economic structures, social systems and the global order. While the first three revolutions introduced and then expanded upon mechanization, electrification and digitization, respectively, the 4IR stands out for its potential to integrate cyber-physical systems and impact all disciplines, economies and industries on a global scale.
How the Industrial Revolutions Have Impacted Design
The industrial revolutions have profoundly influenced design. The technological, social and economic shifts of each era have shaped how, what and why humans design. Here's how each industrial revolution has impacted design:
First Industrial Revolution
Mass Production : The advent of steam-powered machinery enabled the mass production of goods, leading to product standardization. Design during this period focused on functionality and manufacturability, often at the expense of aesthetics and individuality.
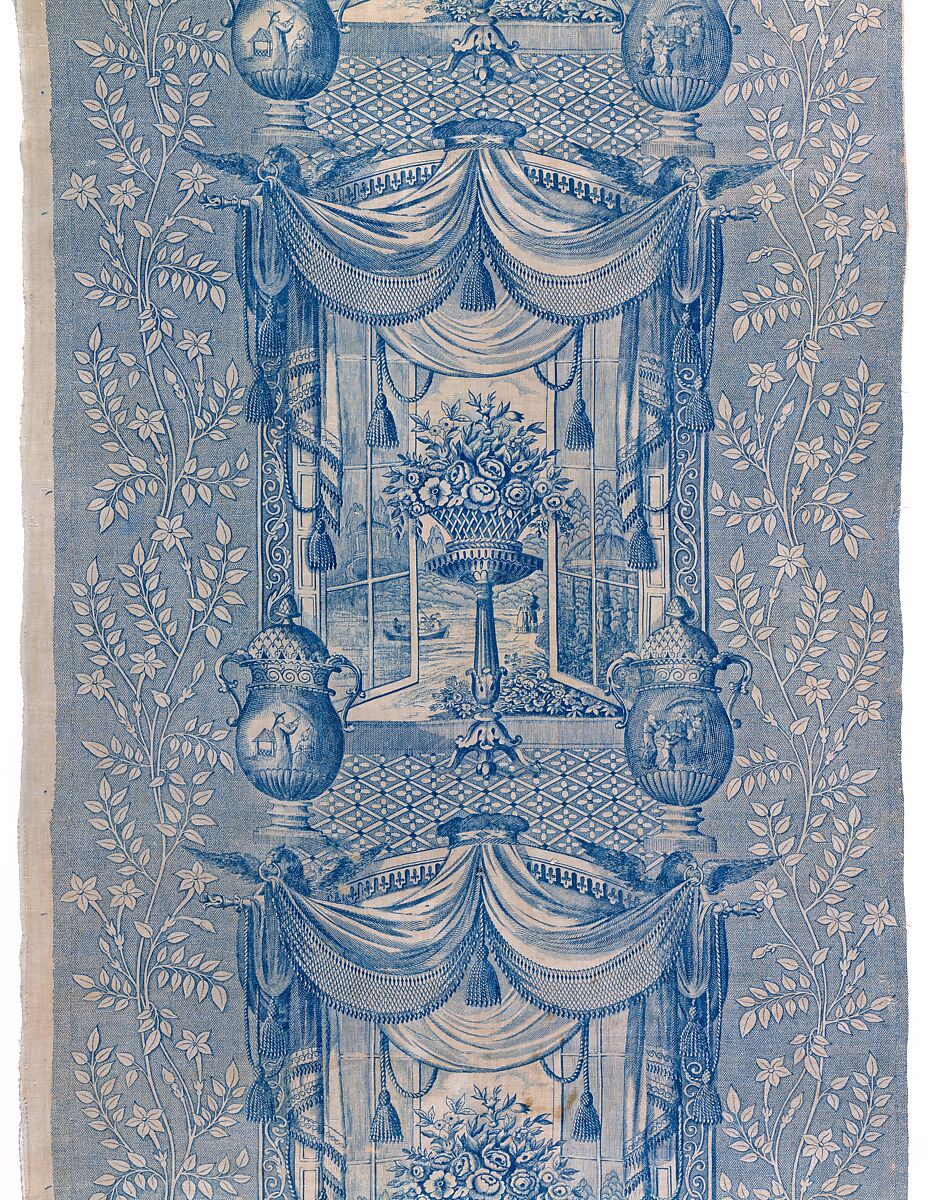
This British printed cotton textile is an example of the 1820 is an example of Regency design.
Second Industrial Revolution
Industrial design : The introduction of assembly line manufacturing and advancements in materials and processes, such as steel production and electrical engineering, birthed the discipline of industrial design. Designers began to focus on the user experience, ergonomics and aesthetic appeal of products and thus recognized the value of design in marketing and brand differentiation.
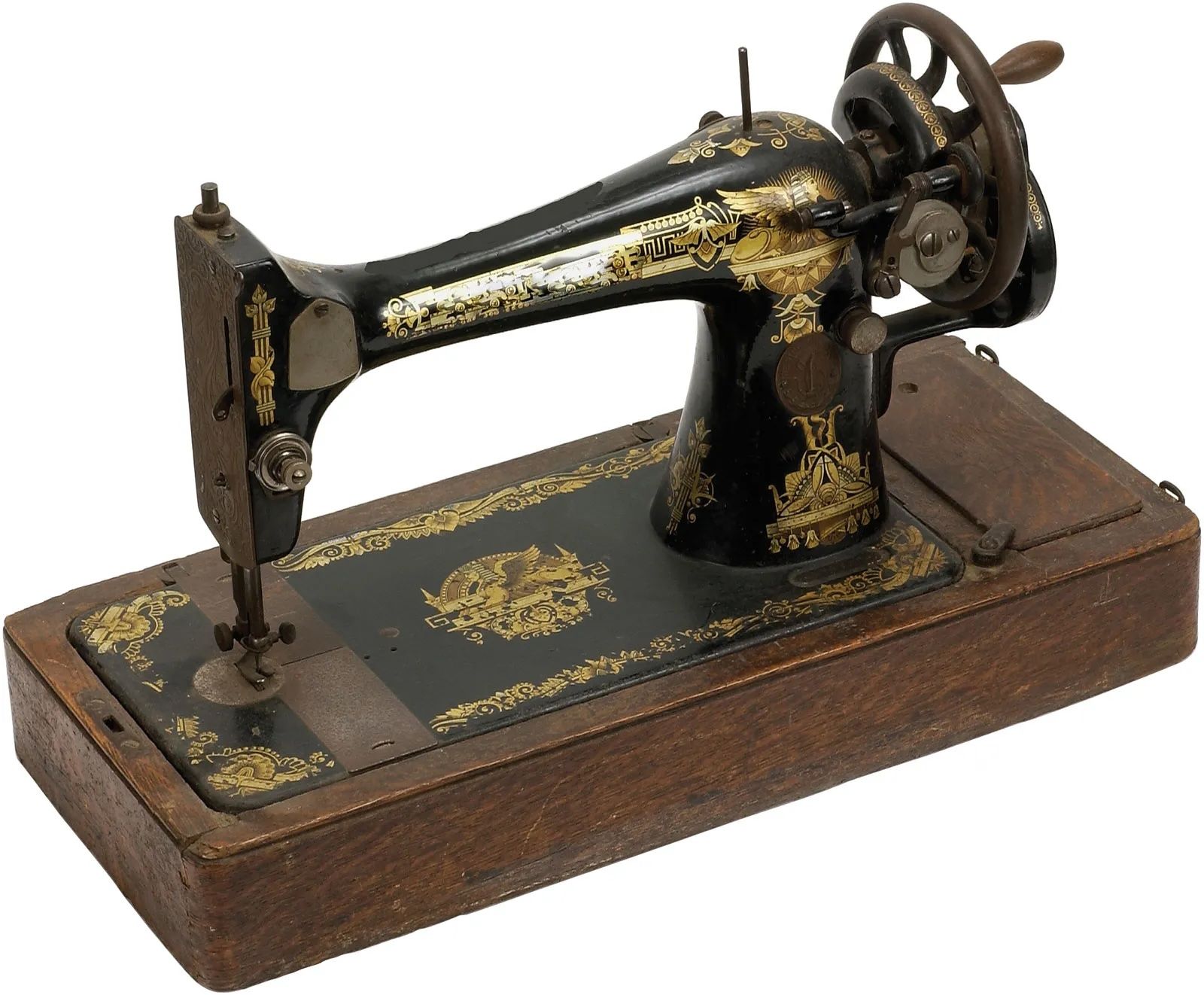
A Singer sewing machine circa 1880.
© Singer, Fair Use
The Singer sewing machine is a pivotal and recognizable invention from the 19th Century. Isaac Merritt Singer, an American inventor, patented the first practical sewing machine in 1851. Their machines were a combination of practical functionality with elaborate Victorian aesthetics. Its design not only made sewing more efficient and less labor-intensive but also turned the sewing machine into a desirable household item. In 1889, they released the first electric sewing machine. The Singer Company's innovations in mass production and global marketing strategies are classic examples of Second Industrial Revolution practices.
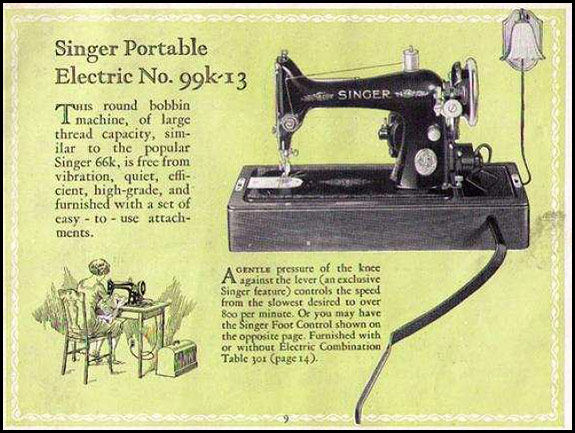
An advertisement for the Singer 99k-13, the first electric sewing machine released in 1889.
Third Industrial Revolution
Digital design : The Digital Revolution introduced computers and digital technology which revolutionized the way designers work. Computer-Aided Design (CAD) and other digital tools enabled more complex and precise designs to foster innovation in product development, architecture and graphic design. The rise of the internet also opened new avenues for digital and web design and emphasized user interface (UI) and user experience (UX) design.
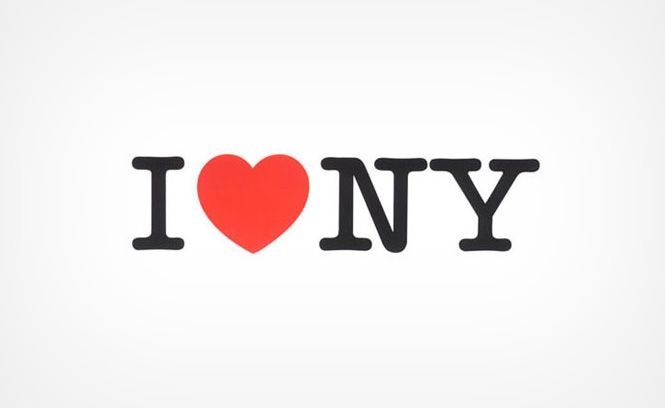
Milton Glaser's "I Love NY" logo was designed in 1977 for a New York State advertising campaign—it’s one of the most iconic works in graphic design. With its simple yet impactful composition, the American Typewriter font paired with a heart symbol replacing the word "love", Glaser's design captured the essence of New York City's resilience and appeal during a time of economic hardship and social unrest. This logo revitalized New York's image and showcased the power of graphic design in shaping public perception and fostering a sense of community and pride. Although the Digital Revolution was in its nascent stage, the impact of evolving technologies on design practices was becoming increasingly apparent.
© Milton Glaser, Fair Use
Learn More About the Fourth Industrial Revolution
Read Klaus Schwab’s book The Fourth Industrial Revolution .
Visit the World Economic Forum’s Centre for the Fourth Industrial Revolution .
Read McKinsey and Company’s piece, What are Industry 4.0, the Fourth Industrial Revolution, and 4IR?
Read about the World Economic Forum’s various 4IR projects .
Check out National Geographic’s collection on the Industrial Revolution . 
Questions about The Fourth Industrial Revolution
Emerging technologies such as AI and IoT are fundamentally transforming the design industry through the introduction of new capabilities for automation, personalization and connectivity. AI is being leveraged to automate routine design tasks, generate innovative design options and provide data-driven insights that can enhance efficiency and creativity. For example, Autodesk's Dreamcatcher is an AI-based generative design system that enables designers to input design goals along with parameters such as materials, manufacturing methods and cost constraints. The system then explores all the possible permutations of a solution and quickly generates design alternatives. IoT, on the other hand, integrates physical objects with sensors and software to allow designers to create interconnected products that can communicate with each other and with users in real-time. A notable example is the Philips Hue lighting system, which allows users to control light settings from their mobile devices, creating personalized environments.
Learn more about how AI is changing design and the world in this video with AI Product Designer, Ioana Teleanu:
In the 4IR, essential skills for designers extend beyond traditional design competencies to include digital literacy, an understanding of emerging technologies and the ability to work with data. Proficiency in tools and platforms that leverage AI, IoT, VR/AR and 3D printing has become increasingly important. For instance, designers must be adept at using AI for user experience personalization and predictive analytics, as seen in platforms like Adobe Sensei, which helps automate and enhance creative tasks. Additionally, critical thinking, creativity and problem-solving remain foundational and enable designers to devise innovative solutions to complex problems. Collaboration skills are also vital, as the multidisciplinary nature of 4IR projects often requires working closely with engineers, data scientists and other specialists. The ability to continuously learn and adapt is crucial, given the rapid pace of technological change.
Learn more about essential skills for the 4IR in our courses AI for Designers , UX Design for Virtual Reality and UX Design for Augmented Reality .
The 4IR has significantly impacted UX and UI design practices by pushing the boundaries of customization, interactivity and user engagement. With the integration of technologies such as AI, IoT, VR and AR, designers are now able to create more personalized and immersive experiences. AI and machine learning offer the ability to analyze user data in real-time which enables the creation of interfaces that adapt to user behaviors and preferences. For example, Spotify uses machine learning to tailor music recommendations to individual tastes to enhance the user experience through personalization.
In addition, VR and AR technologies are redefining user interactions with digital products by offering immersive experiences that were previously not possible. AR apps like IKEA Place allow users to visualize furniture in their homes before making a purchase, merging digital and physical realities to improve decision-making and satisfaction. These advancements demand that UX/UI designers not only focus on traditional design principles but also on understanding and leveraging these emerging technologies to create seamless, intuitive and engaging user experiences. The emphasis on user-centered design has never been more critical as designers strive to ensure that technological advancements enhance rather than complicate the user experience.
Learn more about UX and UI Design for AR, VR and XR in our courses UX Design for Virtual Reality and UX Design for Augmented Reality , as well as our Master Classes How To Craft Immersive Experiences in XR and How to Innovate with XR .
Virtual and Augmented Reality (VR/AR) are transforming product design by enabling designers to create immersive and interactive prototypes which enhances the design process, user testing and user engagement. This capability is invaluable for industries such as automotive and architecture, where designers and engineers can virtually walk through a building or experience a car's interior before any physical prototype is built. For example, Ford uses VR to simulate car designs to allow for rapid iteration and testing of ergonomic and aesthetic features without the need for physical models.
AR, on the other hand, overlays digital information onto the real world to enhance a user's perception of reality. This technology is particularly transformative in retail and interior design, as seen in. IKEA's AR app, IKEA Place.
VR and AR technologies offer powerful tools for designers to not only improve the efficiency and effectiveness of the design process but also to create products and experiences that are more aligned with user needs and expectations. These technologies facilitate a more iterative design process, where feedback can be gathered and implemented quickly and lead to higher-quality and more user-friendly products.
Learn more about UX Design for VR and AR in our courses UX Design for Virtual Reality and UX Design for Augmented Reality .
Klaus Schwab, Founder and Executive Chairman of the World Economic Forum (WEF) coined the term term the Fourth Industrial Revolution. He introduced this concept in his 2016 book of the same name. It remains the most influential book on the topic.
Schwab, K. (2016). The Fourth Industrial Revolution. Portfolio.
In the 4IR, data analytics plays a crucial role in design—it empowers designers with insights that drive more informed, user-centric decisions. Through the analysis of large datasets, designers can uncover patterns, trends and user behaviors that inform every stage of the design process, from conceptualization to final product development. This data-driven approach enables the creation of products and services that truly meet user needs and preferences.
For example, in UX/UI design, data analytics can optimize user interfaces based on actual user interaction data and lead to more intuitive and effective designs. Companies like Netflix use data analytics to tailor content and recommendations to individual users, which enhances user experience. In product design, data analytics can inform feature development, usability improvements and even predict future trends, to ensure products remain relevant and competitive.
Additionally, in the context of sustainable design, data analytics can identify areas where resources can be optimized or reduced, contributing to more environmentally friendly design solutions. Overall, data analytics bridges the gap between user expectations and design outcomes, making it an indispensable tool in the 4IR design toolkit.
Learn more about data-driven design in our course Data-Driven Design: Quantitative Research for UX .
Designers can leverage machine learning (ML) and AI in their work to enhance creativity, efficiency and user experience. One primary way is through the automation of routine tasks such as data analysis, which allows designers to focus more on the creative aspects of their projects. For example, Adobe Sensei, Adobe's AI and ML technology, automates complex processes like image editing and pattern recognition, to speed up the design workflow.
Additionally, ML and AI can generate design alternatives and suggest improvements by learning from vast datasets of design elements and user interactions. This capability supports designers in exploring a wider range of options and making informed decisions based on predicted user preferences and behaviors.
AI can also personalize user experiences in real-time by adapting interfaces, content and recommendations to individual user needs. Streaming services like Netflix and Spotify use AI to analyze viewing or listening habits, respectively, to deliver highly personalized content recommendations, to improve user satisfaction.
Additionally, designers can use AI for more accurate user testing and feedback gathering. Tools powered by AI can simulate how users interact with designs to provide valuable insights without the need for extensive user testing sessions.
Learn more about AI and ML, especially in the context of design, in our course AI for Designers .
Watch the trailer here:
In the Fourth Industrial Revolution, designers face several ethical considerations that stem from the increased use of emerging technologies like AI, IoT and big data analytics. Key ethical considerations include:
Privacy and data protection : With the extensive collection and analysis of user data, designers must ensure they respect user privacy and comply with data protection laws. This involves designing systems that are secure by default and transparent about how user data is collected, used and stored.
Bias and fairness : AI and machine learning algorithms can inadvertently perpetuate biases present in their training data, leading to unfair or discriminatory outcomes. Designers must strive to use diverse datasets and regularly audit algorithms to minimize bias.
Accessibility and inclusiveness : The 4IR offers opportunities to make designs more accessible to a wider audience, including people with disabilities. Designers have a responsibility to ensure their products and services are inclusive, providing equal access and opportunities for everyone.
Sustainability : With the growing concern over environmental issues, designers must consider the ecological impact of their designs. This includes choosing sustainable materials, designing for energy efficiency and considering the entire lifecycle of products to minimize waste.
Accountability and transparency : As AI systems become more autonomous, designers must ensure that these systems are transparent in their decision-making processes and that there are mechanisms in place for accountability, especially in critical applications like healthcare or autonomous vehicles.
User autonomy and manipulation : Designers need to be mindful of not creating manipulative designs that exploit user psychology for profit, such as dark patterns that trick users into making decisions against their interests.
An example of ethical design in practice is the development of AI in healthcare, where designers and developers are working to ensure systems are transparent, explainable and free from bias to recognize the critical impact these systems have on patient care and outcomes. Ethical considerations in the 4IR are complex and evolving, requiring designers to stay informed and engaged with the latest developments in technology ethics.
Learn more about the ethics and transparency in AI in the article AI Challenges and How You Can Overcome Them: How to Design for Trust .
The role of human-centered design (HCD) is evolving significantly with the advent of the 4IR technologies, such as AI, IoT, VR/AR and big data analytics. HCD's core principle is to design with a deep focus on the needs, wants and limitations of end-users. That remains intact, but the scope and impact of this approach have expanded dramatically.
In the 4IR, HCD is not just about products and services that are easy and intuitive to use; it's increasingly about how designers can leverage technology to make life better, work more productive and societies more inclusive. For example, AI and machine learning are being used to create more personalized experiences in everything from healthcare apps that provide tailored health advice, to educational platforms that adapt to the learning pace of individual students.
In addition, HCD in the 4IR means designing for ethics and sustainability—to consider not just the immediate impact of a design on users, but also its long-term effects on society and the environment. This includes using IoT to create smart cities that enhance the quality of life, employing VR to train medical professionals without the need for physical resources and applying big data analytics to tackle complex social issues like poverty and climate change.
Learn more about HCD in our Master Class Human-Centered Design for AI and our article Human-Centered Design: How to Focus on People When You Solve Complex Global Challenges .
The Fourth Industrial Revolution has had a profound impact on sustainable and inclusive design—it’s offered new opportunities and challenges to create solutions that are environmentally friendly and accessible to all. The integration of technologies such as AI, IoT, VR/AR and big data analytics into the design process enables more informed decision-making, which leads to designs that can better address environmental concerns and social inequalities.
In terms of sustainability, 4IR technologies allow for the optimization of resources and energy efficiency in product design and manufacturing processes. For example, AI can be used to analyze and predict patterns in energy consumption, which leads to the development of smarter, more energy-efficient buildings. Similarly, 3D printing technology enables the production of components with minimal waste and the use of sustainable materials further reduces the environmental footprint of manufactured goods.
From an inclusivity perspective, 4IR technologies are breaking down barriers for people with disabilities and those in marginalized communities. For instance, AI-powered assistive devices can improve the quality of life for people with visual or auditory impairments, while AR and VR technologies offer new ways to experience content and services for those who may be physically unable to access them in traditional ways.
Moreover, big data analytics play a crucial role in identifying and addressing gaps in accessibility and inclusivity and enable designers to create products and services that cater to a wider range of needs and preferences. This data-driven approach ensures that design decisions are based on real-world insights for more effective and impactful solutions.
Learn more about sustainable design in our piece What is Sustainable Design? Take our course Design for Better World with Don Norman for an in-depth learning experience.
Literature on The Fourth Industrial Revolution
Here’s the entire UX literature on The Fourth Industrial Revolution by the Interaction Design Foundation, collated in one place:
Learn more about The Fourth Industrial Revolution
Take a deep dive into The Fourth Industrial Revolution with our course Design for a Better World with Don Norman .
“Because everyone designs, we are all designers, so it is up to all of us to change the world. However, those of us who are professional designers have an even greater responsibility, for professional designers have the training and the knowledge to have a major impact on the lives of people and therefore on the earth.” — Don Norman, Design for a Better World
Our world is full of complex socio-technical problems:
Unsustainable and wasteful practices that cause extreme climate changes such as floods and droughts.
Wars that worsen hunger and poverty .
Pandemics that disrupt entire economies and cripple healthcare .
Widespread misinformation that undermines education.
All these problems are massive and interconnected. They seem daunting, but as you'll see in this course, we can overcome them.
Design for a Better World with Don Norman is taught by cognitive psychologist and computer scientist Don Norman. Widely regarded as the father (and even the grandfather) of user experience, he is the former VP of the Advanced Technology Group at Apple and co-founder of the Nielsen Norman Group.
Don Norman has constantly advocated the role of design. His book “The Design of Everyday Things” is a masterful introduction to the importance of design in everyday objects. Over the years, his conviction in the larger role of design and designers to solve complex socio-technical problems has only increased.
This course is based on his latest book “Design for a Better World,” released in March 2023. Don Norman urges designers to think about the whole of humanity, not just individual people or small groups.
In lesson 1, you'll learn about the importance of meaningful measurements . Everything around us is artificial, and so are the metrics we use. Don Norman challenges traditional numerical metrics since they do not capture the complexity of human life and the environment. He advocates for alternative measurements alongside traditional ones to truly understand the complete picture.
In lesson 2, you'll learn about and explore multiple examples of sustainability and circular design in practice. In lesson 3, you'll dive into humanity-centered design and learn how to apply incremental modular design to large and complex socio-technical problems.
In lesson 4, you'll discover how designers can facilitate behavior-change , which is crucial to address the world's most significant issues. Finally, in the last lesson, you'll learn how designers can contribute to designing a better world on a practical level and the role of artificial intelligence in the future of design.
Throughout the course, you'll get practical tips to apply in real-life projects. In the " Build Your Case Study" project, you'll step into the field and seek examples of organizations and people who already practice the philosophy and methods you’ll learn in this course.
You'll get step-by-step guidelines to help you identify which organizations and projects genuinely change the world and which are superficial. Most importantly, you'll understand what gaps currently exist and will be able to recommend better ways to implement projects. You will build on your case study in each lesson, so once you have completed the course, you will have an in-depth piece for your portfolio .
All open-source articles on The Fourth Industrial Revolution
Use circular design to reverse harm.
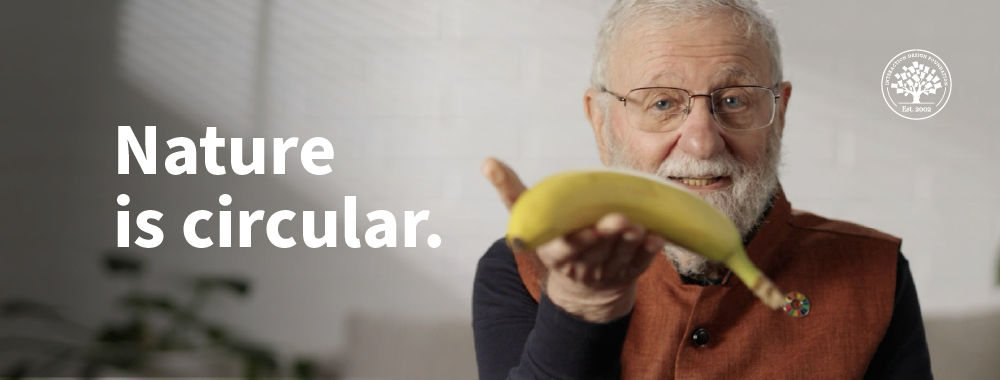
Open Access—Link to us!
We believe in Open Access and the democratization of knowledge . Unfortunately, world-class educational materials such as this page are normally hidden behind paywalls or in expensive textbooks.
If you want this to change , cite this page , link to us, or join us to help us democratize design knowledge !
Privacy Settings
Our digital services use necessary tracking technologies, including third-party cookies, for security, functionality, and to uphold user rights. Optional cookies offer enhanced features, and analytics.
Experience the full potential of our site that remembers your preferences and supports secure sign-in.
Governs the storage of data necessary for maintaining website security, user authentication, and fraud prevention mechanisms.
Enhanced Functionality
Saves your settings and preferences, like your location, for a more personalized experience.
Referral Program
We use cookies to enable our referral program, giving you and your friends discounts.
Error Reporting
We share user ID with Bugsnag and NewRelic to help us track errors and fix issues.
Optimize your experience by allowing us to monitor site usage. You’ll enjoy a smoother, more personalized journey without compromising your privacy.
Analytics Storage
Collects anonymous data on how you navigate and interact, helping us make informed improvements.
Differentiates real visitors from automated bots, ensuring accurate usage data and improving your website experience.
Lets us tailor your digital ads to match your interests, making them more relevant and useful to you.
Advertising Storage
Stores information for better-targeted advertising, enhancing your online ad experience.
Personalization Storage
Permits storing data to personalize content and ads across Google services based on user behavior, enhancing overall user experience.
Advertising Personalization
Allows for content and ad personalization across Google services based on user behavior. This consent enhances user experiences.
Enables personalizing ads based on user data and interactions, allowing for more relevant advertising experiences across Google services.
Receive more relevant advertisements by sharing your interests and behavior with our trusted advertising partners.
Enables better ad targeting and measurement on Meta platforms, making ads you see more relevant.
Allows for improved ad effectiveness and measurement through Meta’s Conversions API, ensuring privacy-compliant data sharing.
LinkedIn Insights
Tracks conversions, retargeting, and web analytics for LinkedIn ad campaigns, enhancing ad relevance and performance.
LinkedIn CAPI
Enhances LinkedIn advertising through server-side event tracking, offering more accurate measurement and personalization.
Google Ads Tag
Tracks ad performance and user engagement, helping deliver ads that are most useful to you.
Share Knowledge, Get Respect!
or copy link
Cite according to academic standards
Simply copy and paste the text below into your bibliographic reference list, onto your blog, or anywhere else. You can also just hyperlink to this page.
New to UX Design? We’re Giving You a Free ebook!

Download our free ebook The Basics of User Experience Design to learn about core concepts of UX design.
In 9 chapters, we’ll cover: conducting user interviews, design thinking, interaction design, mobile UX design, usability, UX research, and many more!
Academia.edu no longer supports Internet Explorer.
To browse Academia.edu and the wider internet faster and more securely, please take a few seconds to upgrade your browser .
- We're Hiring!
- Help Center
Industrial revolution 4.0
- Most Cited Papers
- Most Downloaded Papers
- Newest Papers
- Save to Library
- Last »
Enter the email address you signed up with and we'll email you a reset link.
- Academia.edu Publishing
- We're Hiring!
- Help Center
- Find new research papers in:
- Health Sciences
- Earth Sciences
- Cognitive Science
- Mathematics
- Computer Science
- Academia ©2024
- Skip to main content
- Skip to search
- Skip to footer
Products and Services
Contact cisco.
To get global contact information, please make your selections in the drop-down menus.
Country/region and language
Get in touch
Please reach out to sales for general inquiries or to chat with a live agent.
Sales inquiries
1 800 553 6387 , press 1
Order and billing
1 800 553 6387 , press 2-1
Monday to Friday 8 a.m. to 5 p.m. Eastern Time Chat is available to you 24/7.
Find technical support for products and licensing, access to support case manager, and chat with support assistant. Technical support is available 24/7.
Enterprise and service providers
1 800 553 2447 (U.S. and Canada)
Small business
1 866 606 1866 (U.S. and Canada)
Training and certifications
1 800 553 6387 , press 4
Explore support
Explore certification support
Cisco partners
Become a partner, locate a partner, get updates, and partner support.
Explore Cisco partners
Get partner support
Find a Cisco office
Find offices around the world.
Locate offices
Corporate headquarters
300 East Tasman Drive San Jose, CA 95134
Legal mailing address
Cisco Systems, Inc. 170 West Tasman Drive San Jose, California 95134

Complete the form below or log in and the form will autofill. One of our sales specialists will call you within 15 minutes or on a date or time you request. Specialists are available Monday through Friday, 8 a.m. to 5 p.m. Eastern Time. We are currently experiencing delays in response times. If you require an immediate sales response – please call us 1 800-553-6387. Otherwise, a sales advisor will call you as soon as possible. * Required
Want to use a different email? Sign out * Required

IMAGES
VIDEO
COMMENTS
Introduction. Since the term Industry 4.0 was coined in 2011 (Liao et al., 2017), a growing number of studies from different streams of research has been published on this concept (Culot et al., 2020).The literature has devoted special attention to the digital transformation of industries and companies towards an "Industry 4.0" level (Dalenogare et al., 2018).
The core concept of Industry 4.0 is to integrate advanced information technologies, especially emerging technologies, such as the Internet of Things, 5G & 6G, data analytics and management, artificial intelligence, cloud computing, and blockchain, to achieve a consistent transformation and upgrade of manufacturing and to reshape the value chain of industry and society. More research focuses on ...
The Fourth Industrial Revolution refers to the technological transformation society is undergoing in the 21st Century. This paper explores how technologies such as Artificial Intelligence (AI), Internet of Things (IoT) and autonomous vehicles are increasingly merging with human lives and creating a radical shift for employees, organisations ...
This paper takes a historical approach to analyse the Fourth Industrial Revolution, denominated Industry 4.0. Although its technologies are more evolutive than disruptive, their combination and gradual improvement promise significant impacts on the economy and society, thus characterising a veritable revolution.
Since 2011, when the concepts of Industry 4.0 were first announced, this industrial revolution has grown and expanded from some theoretical concepts to real-world applications. Its practicalities can be found in many fields and affect nearly all of us in so many ways. While we are adapting to new changes, adjustments are starting to reveal on national and international levels. It is becoming ...
In this paper, the general definition of Industry 4.0 will be discussed. ... of the Fourth Industrial Revolution (4IR) technological innovations, new macro and micro-environmental dynamics are ...
Abstract. A new revolution known as Industry 4.0 is occurring where countless elements comprising industrial systems are being interfaced with internet communication technologies to form the smart ...
To understand Industry 4.0 as the Fourth Industrial Revolution, this paper uses a. systematic approach, a nalyzing three main elements of each r evolution: technical. advances, economic scenario ...
The Fourth Industrial Revolution (4IR) signifies an ongoing era characterized by revolutionary technological advancements that profoundly reshape society and the economy. This transformative period is driven by remarkable progress in cutting-edge technologies such as artificial intelligence (AI), robotics, the Internet of Things (IoT), and more.
According to Ngjeqari (Citation 2016) and Beier, Niehoff, and Xue (Citation 2018), the 4th Industrial Revolution is an enabler of sustainable development, but the convergence of digital transformation and sustainability remains underdeveloped (Beier, Niehoff, and Xue Citation 2018; Kiron and Unruh Citation 2018).Recently, some studies have identified several interlinks between Industry 4.0 ...
The development of Fourth Industrial Revolution (4IR) or Industry 4.0 in emerging technologies shall result in the creation of new opportunities for emerging economies growth and development. ... Table 2 indicates papers' inclusion and exclusion ... Industry 4.0 implies lean manufacturing: Research activities in industry 4.0 function as ...
Industry 4.0 (the fourth industrial revolution) encapsulates future industry development trends to achieve more intelligent manufacturing processes, including reliance on Cyber-Physical Systems (CPS), construction of Cyber-Physical Production Systems (CPPS), and implementation and operation of smart factories. This paper introduces relevant aspects of Industry 4.0 in relation to strategic ...
The "Industrial 4.0" concept appeared first in an article published by the German government in ... real-world businesses and research. This paper not only uses literature analysis to sort out the ... This paper uses Industry 4.0 to denote the generalized fourth industrial revolution. 4 BIBLIOMETRIC SURVEY FOR CORE TECHNOLOGIES OF INDUSTRY ...
In the existing literature on Industry 4.0 (I4.0) and sustainability, the prevailing assumption is that digitalization is the key to more efficient and sustainable manufacturing companies (see Beltrami et al., 2021; Birkel and Müller, 2021; Ghobakhloo, 2020 for a review on the topic). For example, some authors suggest that new technologies ...
Industry 4.0, the Fourth Industrial Revolution, and 4IR all refer to the current era of connectivity, advanced analytics, automation, and advanced-manufacturing technology that has been transforming global business for years. This wave of change in the manufacturing sector began in the mid-2010s and holds significant potential for operations ...
1. Introduction. Modern industry industrial development has lasted for several hundred years and has now the era of Industry 4.0 comes. The concept of Industry 4.0 was initially proposed for developing German economy in 2011 [60], [87].According to Lukač [45], the first industrial revolution begins began at the end of the 18th century and is was represented by mechanical production plants ...
Literature Education and Industrial Revolution 4.0. Manonmani Devi M. Annamalai Saravanan P Veeramuthu. Education. 2020. Purpose of the Study: This paper aims to investigate the effects of IR4.0 on literature teaching and education at the university level. In addition, this paper will also investigate the extent to….
The Fourth Industrial Revolution (4IR), also known as Industry 4.0, is a new era of development in which digital, physical and biological systems converge, fundamentally transforming industries, economies and societies. The term Fourth Industrial Revolution was coined by Klaus Schwab, Founder and Executive Chairman of the World Economic Forum ...
Industrial Revolution 4.0 has initiated digital transformation in Asia and resulted in Retail 4.0 forcing shopping malls to upgrade themselves digitally to remain competitive. The purpose of this research is to investigate the impact of upgrading shopping malls with digital retail technology (digital up-gradation) on shoppers' satisfaction ...
The Italian ceramic tiles district has a long tradition but is called to face new sustainability challenges related to the profitability, the environmental impact, and the capability to offer pleasant and motivating working conditions for new young employees. New Industry 4.0 approaches are emerging to evolve the current industrial organization and are expected to enhance the overall economic ...
To achieve this objective, this study performs a systematic literature review and content analysis of 93 papers from 75 journals. The main results of the research show that digital trends resulting from Industry 4.0 affect the field of HRM in 13 different themes, promoting trends and challenges for HRM, the workforce, and organizations.
Complete the form below, and one of our sales specialists will call you within 15 minutes or on a date and time you request. Specialists are available Monday through Friday, 8 a.m. to 5 p.m. Eastern Time.We are currently experiencing delays in response times. If you require an immediate sales response - please call us 1 800-553-6387.
DOI: 10.35940/ijitee.I7144 .0991120. Evolution of Industrial Revolutions: A Review. Ashwani Sharma, Bikram Jit Singh. Abstract: The new economy has seen tremendous strides. since its early ...
The first ith the na e echanical , the second ith Electrical evolution, the third uto ated revolution and the fourth ith igit d ev lution. ( obsba 1990). The 21st century has arrived ith a tsuna i of technological and scie tific achieve ents. o one kno s hat to call the . Even th fourth Industrial revolution has beco e Industry 4.0. ( onner 2018).